-
PDF
- Split View
-
Views
-
Cite
Cite
Tao Lu, Yiwei Huang, Yulei Qiao, Yongxing Zhang, Yu Liu, Evaluation of changes in cartilage viability in detergent-treated tracheal grafts for immunosuppressant-free allotransplantation in dogs, European Journal of Cardio-Thoracic Surgery, Volume 53, Issue 3, March 2018, Pages 672–679, https://doi.org/10.1093/ejcts/ezx317
- Share Icon Share
Abstract
The first tissue-engineered clinical tracheal transplant prepared using the detergent-enzymatic method resulted in graft stenosis, possibly from detergent-enzymatic method-induced graft non-viability. We reported on the transplantation of de-epithelialized tracheal allografts while maintaining cartilage viability in dogs. No lethal stenosis occurred in allografts. Herein, on the basis of previous experimentation, we assessed cartilage viability in detergent-treated cartilages.
Six canine tracheal grafts were treated with detergent [1% t-octylphenoxypolyethoxyethanol (Triton X-100)] before transplantation. The histoarchitecture was evaluated, and the viable chondrocytes ratio was calculated. Glycosaminoglycan was detected using safranin-O staining. Collagen II was tested using immunohistochemistry.
The epithelium was completely removed in 5 grafts. Compared with fresh tracheas, the viable chondrocyte ratio was significantly reduced in the de-epithelialized grafts (100 vs 54.70 ± 8.56%; P < 0.001). Image analysis revealed that the mean optical density of glycosaminoglycan (0.363 ± 0.027 vs 0.307 ± 0.012; P = 0.007) and collagen II (0.115 ± 0.013 vs 0.092 ± 0.011; P = 0.028) was decreased. The observation period ranged from 91 to 792 days. No stenosis occurred in 5 allografts; moderate stenosis developed in 1 allograft during the 4th week after surgery. The chondrocyte nuclei almost completely disappeared. Both glycosaminoglycan (0.307 ± 0.012 vs 0.164 ± 0.104; P = 0.044) and collagen II (0.092 ± 0.011 vs 0.068 ± 0.022; P = 0.022) were significantly degraded.
This study demonstrated successful tracheal transplantation; about 50% of the viable chondrocytes were retained in the cartilage that could prevent development of a lethal stenosis in tracheal grafts.
INTRODUCTION
Airway reconstruction of tracheal defects after extensive resection is a challenge and continues to bewilder thoracic surgeons [1, 2]. Several attempts such as tracheal allografts and tracheal prostheses have been made to provide permanent airway tracts for long-segment resection of the trachea, but all these strategies have potential drawbacks [3, 4]. At present, there is no recognized method to resolve this problem.
Recently, Macchiarini et al. [5] carried out tissue-engineered allogenic tracheal transplantation to reconstruct airway defects without immunosuppressants in a clinic. They used a detergent-enzymatic method (DEM) to prepare a decellularized scaffold to eliminate antigenicity. After transplantation, the short-term results (within 4 months) were promising, but long-term follow-up outcomes revealed that a progressive cicatricial stenosis had developed in the native trachea close to the tissue-engineered trachea anastomosis within 12 months of transplantation that necessitated repeated endoluminal stenting [6]. Subsequently, Elliott et al. [7] reported the results of a 2-year follow-up of a tracheal transplant in a child using a similar decellularized tracheal scaffold without immunosuppressants. Six weeks after the transplantation, the tracheal graft was malacic, necessitating the insertion of 2 bioabsorbable tracheal stents; then, granulation tissue developed, necessitating several balloon dilatations [7, 8]. Thus, the abovementioned outcomes from this clinical application remained unconvincing. However, this method, namely seeding stem cells on a decellularized scaffold, provided a new method of tracheal reconstruction that requires additional study.
We previously adopted a detergent treatment to remove tracheal mucous epithelium and mixed glands that were the primary source of major histocompatibility complex antigens. This method was relatively mild, thus leaving some viable chondrocytes after treatment. Then, we performed allotransplantation in dogs without immunosuppressants. All grafts were incorporated with the host trachea [9]. Meanwhile, we found that maintaining tracheal cartilage viability was necessary for successful immunosuppressant-free allotransplantation [10]. If chondrocyte viability was seriously damaged during the treatment, the transplanted tracheal graft would develop a lethal stenosis. Macchiarini et al. [5] showed that after 25 DEM cycles, the tracheal epithelial and glandular cells were removed, and the few visible chondrocytes were distorted and mostly anuclear. Thus, the treated trachea was regarded as an airway scaffold only, without viability that might have caused malacia or stenosis of the graft. If chondrocyte viability was maintained to some extent after decellularization, graft stenosis and malacia could be prevented or elevated after transplantation.
Our experimental study of tracheal allotransplantation had ended in the early 2000s. However, the clinical application of the first tissue-engineered airway and the outcomes reawakened our interests in the study of de-epithelialized tracheal grafts. Compared with the study by Macchiarini et al. [5], our experimental results were satisfactory. Therefore, we attempted to determine why the grafts in the dogs remained stable after transplantation. In our previous study, the cartilage viability of detergent-treated tracheal grafts was evaluated using only a haematoxylin and eosin stain for observation [10]. This method was simple and direct, but inevitably subjective and rough, so it was not a quantitative method. How to assess cartilage changes after the detergent treatment, including the degree of damage to chondrocyte viability and extracellular matrix (ECM) components and what kind of de-epithelialized tracheal graft could be used for transplantation, had remained to be studied. Therefore, in this study, we summarized our previous experimental data and supplemented it with our experimental data on cartilage viability. We mainly evaluated chondrocyte viability and ECM compositional changes in cartilage on a more detailed level after detergent treatment for the purpose of guiding tracheal graft preparation.
MATERIALS AND METHODS
Data of all animals and the tissue specimens (including fresh tracheas, de-epithelialized grafts and transplanted grafts) used in this analysis are taken from our previous study. This study is the continuation of the previous study [9].
The donor dogs (n = 5) were sacrificed, and their tracheas were harvested carefully and trimmed into several segments (7–8 rings) for detergent treatment. Six tracheal segments served as grafts.
Detergent treatment and allotransplantation
The de-epithelialized tracheal grafts were prepared with a detergent treatment, as described in a previous report [9]. In brief, the inner surface of the tracheal segments was scraped with a plastic blade and then rinsed with running water at room temperature. Then, the detergent treatment was performed at 4 °C. After being immersed in 3.0% saline for 10 min, the inner surface was scraped again. The segments were then immersed in the detergent [1% t-octylphenoxypolyethoxyethanol (Triton X-100); T-9284; Sigma Chemical Co., St Louis, MO, USA] while stirring for 12 h, and the inner surface was scraped again. This treatment was repeated 4 times for approximately 48 h. Subsequently, the grafts were rinsed thoroughly with physiological saline. One ring was resected from each end of every tracheal graft for evaluation. After the process, the grafts were stored in physiological saline for 2–39 h at 4 °C until transplantation. The detergent used in this study was different from the study by Macchiarini et al. In our opinion, the intensity, decellularization cycle times and choice of enzyme were the factors that influence the initial viability of chondrocytes prior to allotransplantation, not the type of detergent.
The grafts were then used as an intrathoracic 5-ring tracheal replacement without immunosuppressants in dogs (n = 6). Orthotopic transplantation was performed, and the anastomotic sites and grafts were wrapped with an omental pedicle. In this study, the entire transplantation procedure lasted approximately 1.5 h but not more than 2 h. After surgery, a bronchoscopic examination was performed at the 2nd and 4th week and monthly thereafter to examine the luminal surfaces of the tracheal allografts. The degree of tracheal stricture was designated as follows: <30% reduction of lumen, slight; 30–60% reduction, moderate and >60% reduction, severe. The animals were intended to be sacrificed during the 3rd and 6th month after transplantation. A single dog was expected to be kept alive for a longer period than the others. Upon the death or sacrifice of the animals, the tracheal allografts were harvested for evaluation.
The specimens including fresh tracheas, de-epithelialized tracheal grafts and transplanted grafts were embedded in paraffin, sectioned (5 μm in thickness) and used for examination.
TUNEL staining
To detect apoptotic chondrocytes, the sections were stained using a terminal deoxynucleotidyl transferase dUTP nick end labeling (TUNEL) test (In-situ Cell Death Detection Kit, Roche) according to the manufacturer’s protocol. Dark brown condensed nuclei in TUNEL-positive cells were considered a positive and sensitive indication of double-stranded DNA fragmentation and a unique feature of cell death/apoptosis. The apoptotic chondrocytes were identified under a high-power lens (magnification ×400).
Histological examination and viable chondrocyte counting
Tracheal sections were stained with haematoxylin and eosin (Servicebio Biotechnology Corp., Wuhan, China) to evaluate tissue morphology. All slices were observed under light microscopy. The chondrocytes and nuclei were observed in 5 randomly selected regions under a high-power lens (magnification ×400), and those that had heavily stained nuclei with intact membranes were regarded as viable cells. The remaining chondrocyte ratio was the ratio of the number of remaining chondrocytes to the total number of chondrocytes. The viable chondrocyte ratio was the ratio of the number of viable chondrocytes to the total number of chondrocytes. The total number of chondrocytes equals the number of remaining chondrocytes plus the number of lost chondrocytes. The mean ± standard deviation was determined for each analysis.
Safranin O/fast green for glycosaminoglycan
The sections were stained with safranin O/fast green (Servicebio Biotechnology Corp.) to detect typical glycosaminoglycan (GAG)-positive expression in hyaline cartilage that normally stains red. For each stained section, 5 randomly non-overlapping fields of view were photographed using the same light intensity at a magnification of ×400. The amount of GAG in cartilage was reflected by the intensity of safranin O staining in the histological sections. Image-Pro Plus 6.0 software (Media Cybernetics, Rockville, MD, USA) was used to determine the mean optical density (MOD) of the safranin O stain intensity and to quantitatively determine the GAG content. The MOD was calculated by dividing the integrated optical density by area.
Immunohistochemistry for collagen II and matrix metalloproteinase 13
The sample sections were stained using immunohistochemistry techniques to detect a type II collagen-positive matrix that normally stains brown. The amount of collagen II in the cartilage was reflected by the intensity of immunohistochemical staining in the histological sections. The primary antibody (1:50 dilution) was rabbit anti-dog collagen II antibody (ab34712, Abcam), and the secondary antibody (1:300 dilution) was horseradish peroxidase-conjugated goat anti-rabbit antibody (ab205718, Abcam). Each section was incubated sequentially at room temperature with the first primary antibody for 12 h and then with a secondary antibody for 50 min. Five random non-overlapping fields of view for each stained section were photographed with a microscope using the same light intensity (magnification ×200). Image-Pro Plus 6.0 software was used to analyse the MOD for the collagen II staining intensity.
The immunohistochemical staining steps for matrix metalloproteinase 13 (primary antibody: rabbit anti-dog matrix metalloproteinase 13; ab39012, Abcam) were similar to those for collagen II.
Statistical analysis
The SPSS software package 20.0 was used for statistical analysis. Continuous data are summarized as mean ± standard deviation. One-way analysis of variance was used to compare the differences among 3 groups. Least significant difference or Dunnett’s T3 was used in comparison between 2 groups. A 2-sided P-value of <0.05 was considered significant.
RESULTS
Histological examination revealed that complete removal of the epithelium and mixed glands was achieved after the detergent treatment in 5 of the 6 grafts (Fig. 1A), and partial residual epithelium was confirmed in the remaining graft (Fig. 1B). TUNEL test showed no apoptotic chondrocytes in the remaining cells in the cartilage (Fig. 2). As shown in Table 1, compared with the fresh trachea (Fig. 3A and B), the remaining chondrocyte ratio in the de-epithelialized tracheal grafts (Fig. 3C and D) was significantly decreased (P = 0.001; Fig. 3G), and the viable chondrocyte ratio remained at about 50%, demonstrating a significant reduction (P < 0.001; Fig. 3H). Immunohistochemical staining revealed that some matrix metalloproteinase 13-positive cells were scattered in the de-epithelialized tracheal graft (Fig. 4). Safranin O/fast green staining demonstrated GAG in red colour, and immunohistochemical staining demonstrated collagen II in brown colour. Compared with the fresh tracheas (Fig. 5A and B), optical density image analysis of the de-epithelialized tracheal graft after detergent treatment (Fig. 5C and D) showed a significant loss of GAG (P = 0.007; Fig. 5G) and collagen II contents (P = 0.028; Fig. 5H).
Category . | Fresh . | De-epithelialized . | Transplanted . | P-value (total) . | P-value (1) . | P-value (2) . |
---|---|---|---|---|---|---|
Ratio (%) | ||||||
Remaining chondrocyte | 100 | 93.72 ± 1.91 | 88.74 ± 3.74 | <0.001 | 0.001 | 0.058 |
Viable chondrocyte | 100 | 54.70 ± 8.56 | 4.80 ± 8.44 | <0.001 | <0.001 | <0.001 |
MOD | ||||||
GAG | 0.363 ± 0.027 | 0.307 ± 0.012 | 0.164 ± 0.104 | <0.001 | 0.007 | 0.044 |
Collagen II | 0.115 ± 0.013 | 0.092 ± 0.011 | 0.068 ± 0.022 | 0.001 | 0.028 | 0.022 |
Category . | Fresh . | De-epithelialized . | Transplanted . | P-value (total) . | P-value (1) . | P-value (2) . |
---|---|---|---|---|---|---|
Ratio (%) | ||||||
Remaining chondrocyte | 100 | 93.72 ± 1.91 | 88.74 ± 3.74 | <0.001 | 0.001 | 0.058 |
Viable chondrocyte | 100 | 54.70 ± 8.56 | 4.80 ± 8.44 | <0.001 | <0.001 | <0.001 |
MOD | ||||||
GAG | 0.363 ± 0.027 | 0.307 ± 0.012 | 0.164 ± 0.104 | <0.001 | 0.007 | 0.044 |
Collagen II | 0.115 ± 0.013 | 0.092 ± 0.011 | 0.068 ± 0.022 | 0.001 | 0.028 | 0.022 |
P-value (1): P-value (total) indicates fresh versus de-epithelialized.
P-value (2): P-value (total) indicates de-epithelialized versus transplanted.
GAG: glycosaminoglycan; MOD: mean optical density.
Category . | Fresh . | De-epithelialized . | Transplanted . | P-value (total) . | P-value (1) . | P-value (2) . |
---|---|---|---|---|---|---|
Ratio (%) | ||||||
Remaining chondrocyte | 100 | 93.72 ± 1.91 | 88.74 ± 3.74 | <0.001 | 0.001 | 0.058 |
Viable chondrocyte | 100 | 54.70 ± 8.56 | 4.80 ± 8.44 | <0.001 | <0.001 | <0.001 |
MOD | ||||||
GAG | 0.363 ± 0.027 | 0.307 ± 0.012 | 0.164 ± 0.104 | <0.001 | 0.007 | 0.044 |
Collagen II | 0.115 ± 0.013 | 0.092 ± 0.011 | 0.068 ± 0.022 | 0.001 | 0.028 | 0.022 |
Category . | Fresh . | De-epithelialized . | Transplanted . | P-value (total) . | P-value (1) . | P-value (2) . |
---|---|---|---|---|---|---|
Ratio (%) | ||||||
Remaining chondrocyte | 100 | 93.72 ± 1.91 | 88.74 ± 3.74 | <0.001 | 0.001 | 0.058 |
Viable chondrocyte | 100 | 54.70 ± 8.56 | 4.80 ± 8.44 | <0.001 | <0.001 | <0.001 |
MOD | ||||||
GAG | 0.363 ± 0.027 | 0.307 ± 0.012 | 0.164 ± 0.104 | <0.001 | 0.007 | 0.044 |
Collagen II | 0.115 ± 0.013 | 0.092 ± 0.011 | 0.068 ± 0.022 | 0.001 | 0.028 | 0.022 |
P-value (1): P-value (total) indicates fresh versus de-epithelialized.
P-value (2): P-value (total) indicates de-epithelialized versus transplanted.
GAG: glycosaminoglycan; MOD: mean optical density.
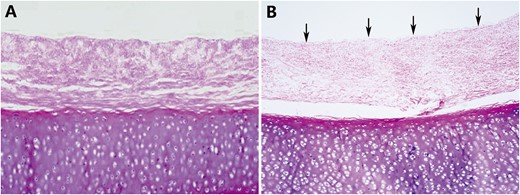
(A) A histological examination showed that the epithelium and mixed glands were completely removed and denuded after detergent treatment. (B) Residual epithelium (arrows) was confirmed in the remaining graft (original magnification ×40).
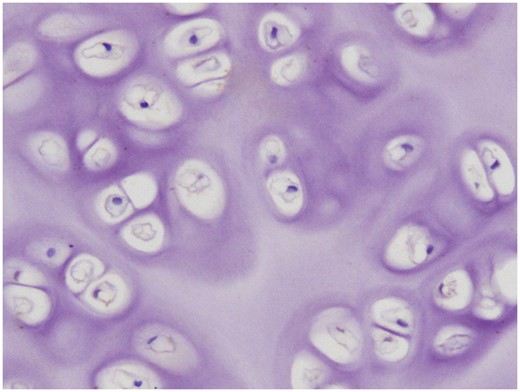
A TUNEL test showed no apoptotic chondrocytes in the de-epithelialized tracheal grafts.
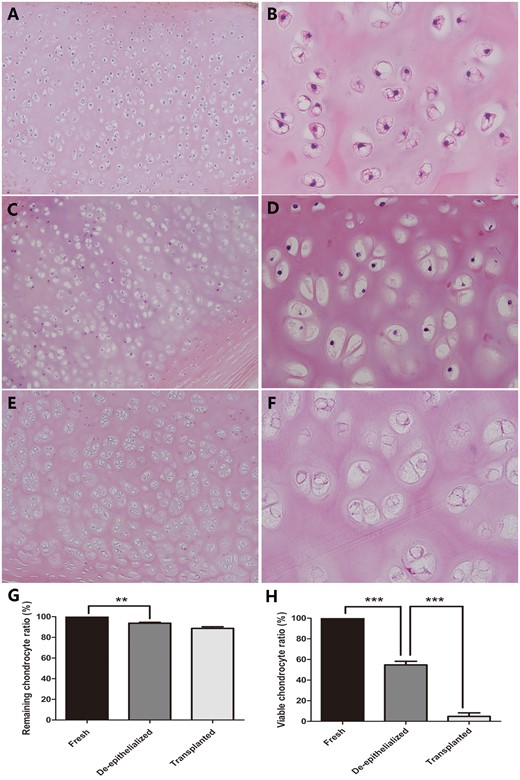
(A and B) Viable chondrocytes were inside the lacunae of the cartilage matrix in the fresh trachea. (C and D) The de-epithelialized tracheal graft showed that some chondrocytes were missing and that evident nuclei loss existed. (E and F) The nuclei in the chondrocytes had almost completely disappeared in the post-transplantation tracheal allograft. (G) The remaining chondrocyte ratio in the de-epithelialized tracheal grafts was significantly decreased, when compared with a fresh trachea. No significant difference was found between the de-epithelialized tracheal grafts and post-transplantation tracheal grafts. (H) The difference in the viable chondrocyte ratio between the fresh tracheas and de-epithelialized tracheal grafts and between the de-epithelialized tracheal grafts and post-transplantation tracheal grafts was obvious. **P < 0.01; ***P < 0.001. (A, C and E original magnification ×100; B, D and F original magnification ×400).
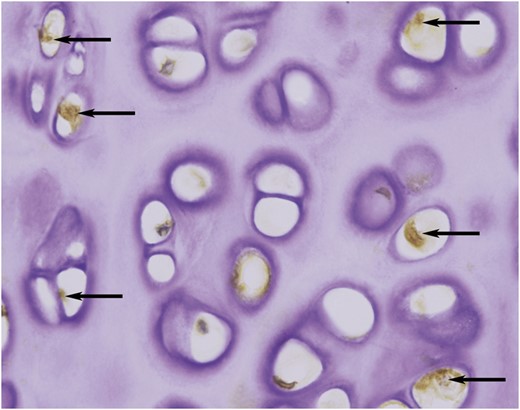
Immunohistochemical staining showed that some matrix metalloproteinase 13-positive chondrocytes (arrows) were scattered in the de-epithelialized tracheal grafts (original magnification ×400).
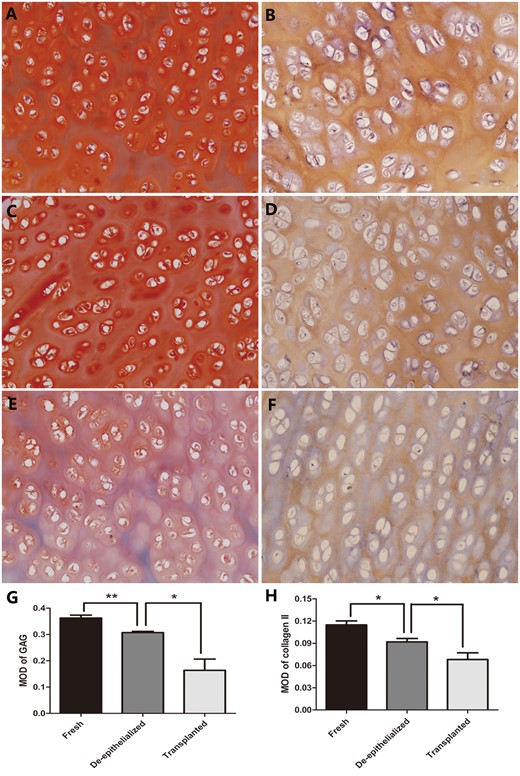
Safranin O/fast green staining for GAG and immunohistochemical staining for collagen II in the fresh tracheas (A and B), de-epithelialized tracheal grafts (C and D) and post-transplantation tracheal grafts (E and F). Optical density analysis showed a significant difference in the MOD of GAG and collagen II between the fresh tracheas and de-epithelialized tracheal grafts and between the de-epithelialized tracheal grafts and post-transplantation tracheal grafts (G and H). *P < 0.05; **P < 0.01. (A–F original magnification ×200). GAG: glycosaminoglycan; MOD: mean optical density.
After transplantation, the 6 animals were observed for the following number of days: 91, 108, 183, 189, 191 and 792 days. Fibre-optic bronchoscopy examination showed that no stenosis had developed in 5 allografts and that the inner surface was shiny (Fig. 6A). In the remaining 1 allograft in which the epithelium and mixed glands were not completely removed, moderate stenosis occurred at the 4th week after transplantation but did not progress any further. This dog was asymptomatic and survived without stent insertion until it was sacrificed at 91 days.
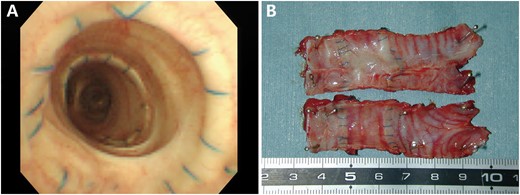
(A) In the allograft from the dog observed for 792 days, the fibre-optic bronchoscopy findings revealed that no stenosis had developed in the shiny inner surface. (B) After harvest, the macroscopic findings revealed that the allograft from the dog observed for 792 days had been incorporated into the host trachea. The luminal surface appeared shiny and patent.
After harvest, macroscopic findings revealed that all allografts had incorporated into the host tracheas (Fig. 6B), including the allograft that developed moderate stenosis. Histological examination showed all allografts were completely covered with regenerated epithelium, which gradually transformed from pseudostratified ciliated columnar epithelium near the anastomotic sites to monolayer epithelium without cilia in the middle portion of the allografts. Evident revascularization was observed in the submucosa of all allografts (Fig. 7A–E). All allografts maintained their structural integrity except for 1 allograft (observation for 91 days), which developed stenosis and showed partial cartilage absorption accompanied by severe lymphocyte and monocyte infiltration (Fig. 7E and F). Compared with the de-epithelialized tracheal graft after the detergent treatment, the remaining chondrocyte ratio in all post-transplantation allografts (Fig. 3E and F) was decreased slightly but was not statistically significant (P = 0.058; Fig. 3G), and the chondrocyte nuclei had almost completely disappeared, indicating hardly any viable chondrocytes existed (P < 0.001; Fig. 3H). In the post-transplantation grafts versus the de-epithelialized tracheal grafts (Fig. 5E and F), optical density image analyses showed that the GAG contents (P = 0.044; Fig. 5G) and collagen II contents (P = 0.022; Fig. 5H) experienced a substantial reduction (Table 1).
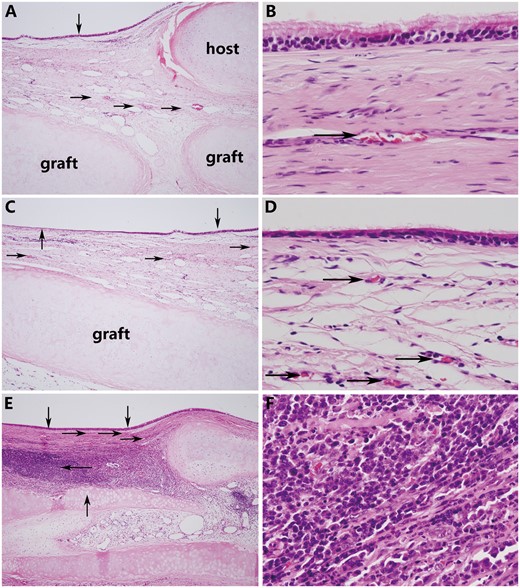
(A) The graft was covered with pseudostratified ciliated columnar epithelium (down arrow), and evident revascularization (right arrow) was observed near the anastomotic sites (the graft was sutured to the host trachea using a telescopic method). (B) Magnification view of regenerative epithelium. (C) The regenerated epithelium gradually transformed from a pseudostratified ciliated columnar epithelium near the anastomotic sites (down arrow) to a monolayer epithelium without cilia in the middle portion of the graft (up arrow). Regenerated small blood vessels (right arrow) were confirmed in the submucosa of the graft. (D) Magnification view of regenerative epithelium. (E) Although the graft was covered with regenerated epithelium (down arrow), the cartilage was partially absorbed and destroyed (up arrow) and accompanied by severe lymphocyte and monocyte infiltration (left arrow). (F) Magnification view. (A, C, and E original magnification ×40; B, D and F original magnification ×400).
DISCUSSION
Consideration was given to the fact that viable cartilage seems to achieve greater post-transplantation success rates than nonviable cartilage; in 2 studies, cartilage transplantation results were not satisfactory because of poor chondrocyte viability [11, 12]. In a related study [10], we used various procedures among 3 groups to deal with tracheal grafts to determine whether cartilage viability should be carefully maintained during treatment. In the 2 groups in which most of the cartilage chondrocytes and nuclei were removed, all animals had died of severe graft stenosis within 40 days. In the other group in which a number of chondrocytes remained viable, the animals were able to survive for a long period of time. Therefore, tracheal cartilage viability was necessary to maintain lumen patency in immunosuppressant-free allotransplantation [10]. We deemed it important to check the cartilage viability of the tracheal grafts before transplantation. Thus, in this study, we attempted to quantify the viability of the tracheal grafts after the detergent treatment by objectively calculating the ratio of chondrocytes possessing viable nuclei in the cartilage. The results showed that about one-half of the viable chondrocytes were retained after the treatment procedures. We also evaluated the influence of the detergent treatment on ECM components. The results indicated that although the main ECM components of the de-epithelialized tracheal graft were damaged by the detergent treatment, a result that seemed unavoidable, chondrocyte viability was still maintained to some extent.
Thus far, some methods have been attempted to produce a decellularization scaffold for tracheal reconstruction. Currently, the clinically applied method is DEM based on deoxycholate and DNase, which provide a nearly acellular airway matrix that is non-immunogenic and has appropriate mechanical properties [5]. Some reports suggested that DEM could remove the tracheal epithelium and that the decellularized scaffold maintained mechanical function and tissue structure [13–16]. However, this method also has negative effects on tracheal grafts. Macchiarini et al. [5] prepared the tracheal scaffold with 25 DEM cycles, after which a few visible chondrocytes were distorted and mostly anuclear. Sun et al. [17] demonstrated a few nucleated cells, with indistinct cell borders that were visible in the decellularized tracheal cartilage after 8 DEM cycles, accompanied by a significant loss of GAG in the decellularized tracheal cartilage. Therefore, it is believed that DEM can remove the epithelium and then eliminate antigenicity more thoroughly from the tracheal grafts than the detergent treatment we used, but this intense decellularization process could also damage the cartilage and result in almost all chondrocytes becoming anuclear, making the tracheal grafts only a biological scaffold without viability. Two subjects who had undergone clinical application demonstrated that the tracheal scaffold previously decellularized by DEM developed stenosis or malacia after transplantation [6, 8]. These findings demonstrate that the non-viable tracheal scaffold would not meet the demands of a tissue-engineered matrix and likely would not yield a suitable graft for lifelong implantation. In our study, the method we used was different from that of DEM. We removed the epithelium and mixed glands using a detergent treatment. This method was relatively mild, characterized by a low temperature (4 °C), few cycles (repeated 4 times) and no enzyme use.
Immunorejection and re-epithelization are important factors for successful tracheal allotransplantation. In this study, the tracheas were treated with detergent and used in an immunosuppressant-free allotransplantation. After transplantation, histological examination verified no evident lymphocyte or monocyte infiltration in any of the allografts, except for 1 allograft. This illustrated that the detergent treatment could eliminate the antigenicity of the tracheal grafts by removing the epithelium and mixed glands. However, the absence of epithelium was considered an important factor for granulation tissue proliferation and lumen stenosis [18]. Epithelial regeneration could prevent fibroblast proliferation and play an essential role in the patency of tracheal allografts [19]. We demonstrated that re-epithelization occurred without extensive infection or granulation tissue formation following tracheal transplantation. It was believed that the regenerated epithelium migrated from the adjacent mucosa to the tracheal graft to form functional respiratory epithelium [20, 21].
Graft ischaemia is another problem in tracheal transplantation. Some evidence has indicated that the blood supply would be critical if successful tracheal transplantation were ever possible. Macchiarini et al. [5, 6] and Elliott et al. [7, 8] had not taken any measures to provide blood to the tracheal grafts. Lack of revascularization may be one reason why graft stenosis or malacia was demonstrated in their clinical application. Successful transplantation requires proper promotion of revascularization. Only a living substitute, therefore vascularized, can fulfil the anatomical, mechanical and anti-infectious functions of the trachea [22]. Thus, we used the omental pedicle to support the blood supply. Histological findings revealed the rich regeneration of blood vessels in the submucosa that could promote epithelial regeneration. Regenerative epithelium could prevent the inner surface of the grafts from having granulation tissue proliferation. Therefore, revascularization was a necessary prerequisite to maintain graft survival and function [23]. All the abovementioned points indicated that the detergent-treated tracheal grafts were capable of preventing immunorejection, inducing re-epithelization and revascularization, thus maintaining lumen patency in vivo.
After transplantation, the nuclei of the chondrocytes had almost completely disappeared, signifying that the chondrocytes had died. The graft had become a bioscaffold without viability to maintain airway patency only. Although omental wrapping allowed the tracheal grafts to recover from early ischaemic changes as new vessels connected to the grafts [24], it could not sustain chondrocyte viability in long-segment tracheal grafts [25]. Therefore, cartilage ECM would no longer be generated. An MOD analysis demonstrated that the GAG and collagen II were substantially degraded after transplantation. In our opinion, maintaining chondrocyte viability to some degree could prevent tracheal grafts from developing granulation tissue during the initial transplantation period, and it may be conducive to promote the process of re-epithelization and revascularization. Beyond the early stages of transplantation, the chondrocytes in the grafts would die because of a lack of nutrition and weak immunorejection. The tracheal grafts became a scaffold without viability to maintain an open airway only. Furthermore, it was considered that with increasing time for transplantation, the tracheal graft would eventually be replaced with fibrotic tissue [22].
Limitations
One limitation of this study was the small number of experimental animals. Second, in our view, an ideal approach for tracheal grafting would be to remove the epithelium and mixed glands from the graft but retain the chondrocyte viability completely. However, the detergent treatment we used could not achieve the desired results. While removing the epithelium and mixed glands, damage to cartilage is an inevitable consequence. In this study, 50% of the viable chondrocytes were retained in the cartilage after the detergent treatment that could prevent the development of a lethal stenosis in the tracheal grafts, but the optimum percentage of viable chondrocytes retained after treatment needs to be further explored. Third, in this study, incomplete removal of the epithelium was observed in 1 of the 6 treated grafts, so repeating the detergent treatment 4 times cannot ensure consistent results. We need to strike a balance between removing the epithelium and retaining the viable chondrocytes.
CONCLUSION
In conclusion, although the GAG and collagen II in the ECM could be damaged during the detergent treatment, chondrocyte viability would be maintained to some extent. About 50% of the viable chondrocytes in the cartilage remained after the de-epithelialization process, which could prevent stenosis and malacia development in the tracheal grafts, providing a compatible and biological tracheal graft to increase the chances of a successful tracheal allotransplantation.
Conflict of interest: none declared.
REFERENCES
Author notes
Tao Lu and Yiwei Huang contributed equally to this work.