-
PDF
- Split View
-
Views
-
Cite
Cite
Manuel J Antunes, José Rodríguez-Palomares, Bernard Prendergast, Michele De Bonis, Raphael Rosenhek, Nawwar Al-Attar, Fabio Barili, Filip Casselman, Thierry Folliguet, Bernard Iung, Patrizio Lancellotti, Claudio Muneretto, Jean-François Obadia, Luc Pierard, Piotr Suwalski, Pepe Zamorano, on behalf of the ESC Working Groups of Cardiovascular Surgery and Valvular Heart Disease, Management of tricuspid valve regurgitation: Position statement of the European Society of Cardiology Working Groups of Cardiovascular Surgery and Valvular Heart Disease, European Journal of Cardio-Thoracic Surgery, Volume 52, Issue 6, December 2017, Pages 1022–1030, https://doi.org/10.1093/ejcts/ezx279
- Share Icon Share
Abstract
Tricuspid regurgitation (TR) is a very frequent manifestation of valvular heart disease. It may be due to the primary involvement of the valve or secondary to pulmonary hypertension or to the left-sided heart valve disease (most commonly rheumatic and involving the mitral valve). The pathophysiology of secondary TR is complex and is intrinsically connected to the anatomy and function of the right ventricle. A systematic multimodality approach to diagnosis and assessment (based not only on the severity of the TR but also on the assessment of annular size, RV function and degree of pulmonary hypertension) is, therefore, essential. Once considered non-important, treatment of secondary TR is currently viewed as an essential concomitant procedure at the time of mitral (and, less frequently, aortic valve) surgery. Although the indications for surgical management of severe TR are now generally accepted (Class I), controversy persists concerning the role of intervention for moderate TR. However, there is a trend for intervention in this setting, especially at the time of surgery for left-sided heart valve disease and/or in patients with significant tricuspid annular dilatation (Class IIa). Currently, surgery remains the best approach for the interventional treatment of TR. Percutaneous tricuspid valve intervention (both repair and replacement) is still in its infancy but may become a reliable option in future, especially for high-risk patients with isolated primary TR or with secondary TR related to advanced left-sided heart valve disease.
INTRODUCTION
Tricuspid regurgitation (TR) is one of the most common manifestations of valvular heart disease (VHD) and may affect 65–85% of the population [1, 2]. Although minimal or mild TR may be a normal variant in structurally normal valves, moderate-to-severe TR is usually pathological and arises as a result of leaflet abnormalities and/or annular dilatation [3].
Primary (organic) TR implies pathology of the tricuspid valve (TV) complex and may be of rheumatic, degenerative, congenital, infectious, traumatic or iatrogenic (usually secondary to pacemaker leads) origin. Secondary (or functional) TR is far more common and related to right ventricular (RV) dilatation and/or dysfunction, annular dilatation and leaflet tethering, which are, in turn, usually secondary to left-sided VHD (especially affecting the mitral valve) [4], atrial fibrillation or pulmonary hypertension [5, 6].
Historically, functional TR was usually treated conservatively (i.e. no surgery), based on the misconception that it improved after surgery of the primary left-sided VHD. However, recent studies have shown that TR progresses in a significant number of patients subsequent to and despite successful treatment of left-sided valve lesions [7]. Furthermore, chronic RV overload is associated with the development of irreversible RV dysfunction, whose prognosis is related to the severity of associated TR. As a consequence, the importance of TV repair at the time of left-sided valve surgery has gained acceptance in recent years [2].
The optimal timing of surgery for isolated TR remains controversial, and operation is commonly undertaken at a late stage, because many patients remain asymptomatic (despite impaired RV function) or present high surgical risk as a result of comorbidities and/or older age [2, 3, 5]. A further contentious issue relates to the techniques of TV surgery, because different repair methods have been proposed. Furthermore, various percutaneous approaches have been developed for use in high-risk patients in recent years [6, 7].
Recent international guidelines on VHD [European Society of Cardiology (ESC) and American Heart Association (AHA)/ACC] contain sparse recommendations regarding the diagnosis and treatment of TR [8, 9]. In this position paper, the European Society of Cardiology Working Groups on Cardiovascular Surgery and Valvular Heart Disease review the literature and attempt to address the most frequent questions concerning this condition that has recently drawn increasing attention. Our conclusions are intended to complement the broader recommendations provided by international guidelines (whose content we fully endorse).
ANATOMY AND FUNCTION OF THE TRICUSPID VALVE
The TV separates the right atrium and ventricle and is composed of 3 leaflets: anterior, posterior and septal (in decreasing order of size and in a clockwise direction as seen by the surgeon), within a partial fibrous annulus, which is part of the fibrous skeleton of the heart. Occasionally, there may be additional (accessory) leaflets. Each leaflet is connected to the homonymous papillary muscle, although an individual septal papillary muscle is often non-existent and replaced by small muscle heads arising directly from the interventricular septum. Valve function is complex and involves the chordae tendineae, papillary muscles and the right atrial and ventricular myocardium [10].
The tricuspid annulus has a non-planar (3D), elliptical shape in healthy individuals. The posteroseptal part is ‘lowest’ and deviated towards the RV apex, whereas the anteroseptal part is ‘highest’ and closer to the RV outflow tract and aortic valve. The shape and size of the annulus change during the cardiac cycle. In functional TR, the annulus becomes planar, dilating primarily in the direction of the anteroposterior commissure resulting in a more circular shape (the septal annulus is part of the cardiac fibrous skeleton and relatively spared) [11]. The more severe the TR, the more planar and circular the annulus becomes. Progressive TR begets further RV dilatation that, in turn, leads to RV failure (see Imaging the tricuspid valve section).
The TV is closely related to 2 important structures that can be injured at the time of intervention. The bundle of His crosses the septal segment of the tricuspid annulus approximately 5 mm from the anteroseptal commissure. At this point, the bundle penetrates posteriorly beneath the membranous septum to reach the crest of the muscular septum. Of equal importance, the right coronary artery runs in the right atrioventricular groove, a few millimetres from the descending (anteroposterior) segment of the tricuspid annulus.
AETIOLOGY OF TRICUSPID REGURGITATION
TR may be a consequence of primary (organic) pathology of the valve leaflets or, more commonly, a secondary consequence of dilatation of the RV and tricuspid annulus in the context of pulmonary hypertension and RV failure [12].
Primary tricuspid regurgitation
Worldwide, rheumatic fever remains the most frequent cause of primary TV disease, leading to scarring of the valve leaflets and/or chordae with resulting restriction of leaflet mobility. Combined stenotic and regurgitant lesions of varying degrees of severity are not infrequently encountered (often in association with mitral valve disease), whereas isolated tricuspid stenosis or pure isolated TR are rare. The annulus is usually dilated [13].
Primary TR may also be of congenital origin, either as an isolated lesion or in association with atrioventricular canal defects, aneurysms of the ventricular septum or as a component of Ebstein’s anomaly. TV prolapse as a result of myxomatous change of the valve leaflets and chordae tendinae is relatively unusual and often associated with similar changes affecting the mitral valve, and with atrial septal defects.
Primary TR is a common manifestation of carcinoid syndrome, where white fibrous carcinoid plaques develop on the endocardium of valve cusps and cardiac chambers, and on the intima of the great veins and coronary sinus. These have a propensity for the ventricular surfaces of the valve, resulting in adherence of the leaflets to the RV free wall [14].
Another common acquired cause of primary TR is infective endocarditis (most frequently seen in intravenous drug addicts with staphylococcal infection or in patients with pacing or device leads) [15, 16]. Rarer causes include drugs (methysergide and pergolide), cardiac tumours (particularly right atrial myxoma), endomyocardial fibrosis and systemic lupus erythematosus. Finally, direct or non-penetrating trauma is not an infrequent cause of TR.
Secondary tricuspid regurgitation
Secondary TR, the most prevalent tricuspid pathology, may be observed in patients with RV pressure overload secondary to any form of cardiac or pulmonary vascular disease and reflects the presence and severity of RV failure. The most common causes are left-sided VHD (particularly mitral but increasingly also aortic), primary or secondary pulmonary hypertension, persistent atrial fibrillation (with tricuspid annular dilatation), RV ischaemia and cardiomyopathy.
Secondary TR is most commonly associated with rheumatic VHD but may also result from degenerative and ischaemic mitral valve disease as a consequence of pulmonary hypertension and RV volume overload. Long-standing, persisting atrial fibrillation may also lead to TR secondary to annular dilatation [17].
PATHOPHYSIOLOGY OF THE RIGHT VENTRICLE IN ASSOCIATION WITH TRICUSPID REGURGITATION
The relationship between TR and the RV is complex and differs according to the mechanism of TR. Primary TR causes pure volume overload on initially normal right-sided cardiac chambers [3]. Conversely, RV enlargement is the major cause of secondary TR, through tricuspid annular dilatation and valve tenting [18]. The severity of functional TR and RV dilatation is strongly correlated with the presence of leaflet tethering (but less so with annular diameter which reflects leaflet size rather than valve function). Annular dilatation appears to be a secondary consequence of RV dilatation. The interplay of echocardiographic and cardiac magnetic resonance imaging remains poorly defined (see below) and is the subject of ongoing investigation.
RV enlargement is most often the consequence of increased afterload due to pulmonary hypertension, usually post-capillary pulmonary hypertension (defined as pulmonary artery wedge pressure >15 mmHg), reflecting increased left-side filling pressures. Precapillary pulmonary hypertension (defined as pulmonary artery wedge pressure ≥15 mmHg with pulmonary vascular resistance >3 Wood units) is most often due to intrinsic lung diseases or pulmonary thromboembolism [19].
The RV has less muscle mass than the left ventricle, and RV systolic function is therefore more load sensitive. Thus, pulmonary hypertension results in an early fall in RV ejection fraction and associated RV enlargement [20]. Initial pressure overload thereby rapidly translates into mixed pressure and volume overload, progressive RV dilatation and secondary TR—a vicious circle, because TR itself contributes to further RV enlargement, thereby increasing tenting and TR severity [21]. Less frequently, secondary TR may be due to RV enlargement without increased afterload (idiopathic secondary TR) [18].
The severity of secondary TR and its RV consequences may be partly reversible after correction of the primary cause (particularly in the case of left-sided VHD) [22]. However, RV dysfunction may be irreversible and even initial impairment of RV function is a strong independent predictor of survival after left-sided valve surgery [23]. Increased RV volumes are also associated with reduced survival in isolated TR [24]. Although RV volume thresholds have been defined for predicting the recovery of RV impairment due to pulmonary regurgitation in congenital heart disease [25], no comparable thresholds have been defined for secondary TR [6]. RV enlargement is also responsible for complex interactions with the left ventricle, and left ventricular impairment may itself lead to reduced RV stroke volume [26].
Patients with heart failure and preserved ejection fraction may present with diastolic dysfunction, atrial fibrillation and varying degrees of TR, which have independent prognostic impact [27]. Finally, a ‘restriction-dilatation syndrome’ occurs when systolic function ‘fails’; diastolic pressure raises and the interventricular septum moves towards the left ventricle during diastole, which in turn raises the left ventricular diastolic pressure and perpetuates TR [20, 28–30].
CLINICAL PRESENTATION AND NATURAL HISTORY
TR is often clinically silent and symptoms usually relate to concomitant left-sided VHD. General fatigue and reduced exercise capacity may occur as a result of low cardiac output, and upper abdominal pain may accompany hepatic congestion. Peripheral lower limb oedema may be present in advanced cases and cause significant discomfort.
Typical signs on auscultation are a soft pansystolic murmur at the lower sternal border and xiphoid process, typically increasing in intensity during inspiration. However, these signs are non-specific and may be difficult to identify. Signs of right heart failure are present in advanced TR: systolic jugular distension, pulsatile hepatomegaly and peripheral oedema. Ascites, liver failure and cachexia may be observed in end-stage disease [12].
The electrocardiogram frequently shows right bundle branch block and atrial fibrillation reflects disease evolution. The chest X-ray shows cardiomegaly secondary to right atrial and ventricular enlargement.
Isolated primary TR evolves slowly but is associated with excess mortality in comparison with the general population. The 10-year incidence of dyspnoea or congestive heart failure was estimated at 57% in asymptomatic patients and strongly related to RV enlargement [31]. Similarly, RV dysfunction, TR severity and increasing pulmonary artery pressure are factors predicting survival in isolated TR [32].
Secondary TR is frequently associated with left-sided VHD, which is the most important determinant of prognosis. Nevertheless, TR has a prognostic value per se that is proportional to its severity, even after adjustment for left ventricular function and pulmonary artery pressure [33]. Secondary TR may improve after correction of left-sided VHD but may also persist or even worsen [34]. This evolution is unpredictable, and severe late TR is associated with reduced survival [35, 36]. Isolated secondary TR is less frequent and prognosis strongly depends on TR severity (10-year survival 38% vs 70% in those with severe and non-severe TR, respectively) [24].
IMAGING THE TRICUSPID VALVE
The goals of imaging in patients with TR are the assessment of severity, aetiology and consequences (e.g. RV size and function, pulmonary artery dimension and pressure) and the detection of concomitant left-sided VHD (including the assessment of prosthetic valve function, where appropriate).
Echocardiography is the principal imaging modality for the assessment of valve morphology and grading TR (Table 1). Routinely, only 2 leaflets can be seen simultaneously using a standard 2D view. Evaluation with 3D imaging is a useful tool [37], allowing simultaneous imaging of all 3 leaflets and commissures.
Parameters . | Mild . | Moderate . | Severe . |
---|---|---|---|
Qualitative | |||
TV morphology | Normal/abnormal | Normal/abnormal | Abnormal/flail/large coaptation defect |
Colour flow TR jet | Small, central | Intermediate | Very large central jet or eccentric wall impinging jet |
CW signal of TR jet | Faint/parabolic | Dense/parabolic | Dense/triangular with early peaking (peak <2 m/s in massive TR) |
Semi-quantitative | |||
VC width (mm)a | Not defined | <7 | >7 |
PISA radius (mm)b | ≤5 | 6–9 | >9 |
Hepatic vein flowc | Systolic dominance | Systolic blunting | Systolic flow reversal |
Tricuspid inflow | Normal | Normal | E-wave dominant (≥1 m/s)d |
Quantitative | |||
EROA (mm2) | Not defined | Not defined | ≥40 |
R Vol (ml)e | Not defined | Not defined | ≥45 |
Parameters . | Mild . | Moderate . | Severe . |
---|---|---|---|
Qualitative | |||
TV morphology | Normal/abnormal | Normal/abnormal | Abnormal/flail/large coaptation defect |
Colour flow TR jet | Small, central | Intermediate | Very large central jet or eccentric wall impinging jet |
CW signal of TR jet | Faint/parabolic | Dense/parabolic | Dense/triangular with early peaking (peak <2 m/s in massive TR) |
Semi-quantitative | |||
VC width (mm)a | Not defined | <7 | >7 |
PISA radius (mm)b | ≤5 | 6–9 | >9 |
Hepatic vein flowc | Systolic dominance | Systolic blunting | Systolic flow reversal |
Tricuspid inflow | Normal | Normal | E-wave dominant (≥1 m/s)d |
Quantitative | |||
EROA (mm2) | Not defined | Not defined | ≥40 |
R Vol (ml)e | Not defined | Not defined | ≥45 |
An IVC diameter <2.1 cm is considered normal.
At a Nyquist limit of 50–60 cm/s.
Baseline Nyquist limit shift of 28 cm/s.
Unless other reasons of systolic blunting (atrial fibrillation or elevated RA pressure).
In the absence of other causes of elevated RA pressure.
The RA, RV size and IVC are usually of normal size in patients with mild TR. An end-systolic RV eccentricity index >2 suggests severe TR. In acute severe TR, the RV size is often normal. In chronic severe TR, the RV is classically dilated. Accepted cut-off values for non-significant right-sided chambers enlargement (measurements obtained from apical 4-chamber view): mid RV cavity diameter ≤33 mm, RV end-diastolic area ≤28 cm2, RV end-systolic area ≤16 cm2, RV fractional area change >32%, maximal 2D RA volume ≤33 ml/m2.
CW: continuous-wave; EROA: effective regurgitant orifice area; IVC: inferior vena cava; PISA: proximal isovelocity surfasse área; RA: right atrium; RV: right ventricle; R Vol: regurgitant volume; TR: tricuspid regurgitation; TV: tricuspid valve; VC: vena contracta.
Parameters . | Mild . | Moderate . | Severe . |
---|---|---|---|
Qualitative | |||
TV morphology | Normal/abnormal | Normal/abnormal | Abnormal/flail/large coaptation defect |
Colour flow TR jet | Small, central | Intermediate | Very large central jet or eccentric wall impinging jet |
CW signal of TR jet | Faint/parabolic | Dense/parabolic | Dense/triangular with early peaking (peak <2 m/s in massive TR) |
Semi-quantitative | |||
VC width (mm)a | Not defined | <7 | >7 |
PISA radius (mm)b | ≤5 | 6–9 | >9 |
Hepatic vein flowc | Systolic dominance | Systolic blunting | Systolic flow reversal |
Tricuspid inflow | Normal | Normal | E-wave dominant (≥1 m/s)d |
Quantitative | |||
EROA (mm2) | Not defined | Not defined | ≥40 |
R Vol (ml)e | Not defined | Not defined | ≥45 |
Parameters . | Mild . | Moderate . | Severe . |
---|---|---|---|
Qualitative | |||
TV morphology | Normal/abnormal | Normal/abnormal | Abnormal/flail/large coaptation defect |
Colour flow TR jet | Small, central | Intermediate | Very large central jet or eccentric wall impinging jet |
CW signal of TR jet | Faint/parabolic | Dense/parabolic | Dense/triangular with early peaking (peak <2 m/s in massive TR) |
Semi-quantitative | |||
VC width (mm)a | Not defined | <7 | >7 |
PISA radius (mm)b | ≤5 | 6–9 | >9 |
Hepatic vein flowc | Systolic dominance | Systolic blunting | Systolic flow reversal |
Tricuspid inflow | Normal | Normal | E-wave dominant (≥1 m/s)d |
Quantitative | |||
EROA (mm2) | Not defined | Not defined | ≥40 |
R Vol (ml)e | Not defined | Not defined | ≥45 |
An IVC diameter <2.1 cm is considered normal.
At a Nyquist limit of 50–60 cm/s.
Baseline Nyquist limit shift of 28 cm/s.
Unless other reasons of systolic blunting (atrial fibrillation or elevated RA pressure).
In the absence of other causes of elevated RA pressure.
The RA, RV size and IVC are usually of normal size in patients with mild TR. An end-systolic RV eccentricity index >2 suggests severe TR. In acute severe TR, the RV size is often normal. In chronic severe TR, the RV is classically dilated. Accepted cut-off values for non-significant right-sided chambers enlargement (measurements obtained from apical 4-chamber view): mid RV cavity diameter ≤33 mm, RV end-diastolic area ≤28 cm2, RV end-systolic area ≤16 cm2, RV fractional area change >32%, maximal 2D RA volume ≤33 ml/m2.
CW: continuous-wave; EROA: effective regurgitant orifice area; IVC: inferior vena cava; PISA: proximal isovelocity surfasse área; RA: right atrium; RV: right ventricle; R Vol: regurgitant volume; TR: tricuspid regurgitation; TV: tricuspid valve; VC: vena contracta.
Trivial/mild TR is frequently encountered in normal people. This so-called ‘physiological’ TR is characterized by normal leaflet morphology and a thin, central TR colour jet adjacent to valve closure [38]. Secondary TR is a consequence of tricuspid annular dilatation and leaflet tethering [39], and quantitation of these parameters is useful to predict TR. 2D echocardiographic evaluation of the annular diameter underestimates the actual size due to its elliptical shape. Consequently, the annular diameter measured in a 4-chamber (septal–lateral) view or a short-axis view (oblique) is less than the true anteroposterior diameter [37]. In adults, the normal tricuspid annular diameter is 28 ± 5 mm (4-chamber view in diastole). Current ESC/EACTS guidelines suggest a threshold diameter ≥40 mm (>21 mm/m2) as an indicator for surgery [40].
The severity of the TR changes during the respiratory cycle due to inspiratory increase in RV size and change of morphology, resulting in tricuspid annular dilatation and leaflet tethering [41]. A difference in the peak TR velocity between inspiration and expiration ≥0.6 m/s is associated with severe TR (sensitivity of 66% and specificity of 94%) [42].
Increased apical displacement of the tricuspid leaflets (tethering) is evaluated by measuring the tenting area (area between the atrial surface of the leaflets and the annular plane at maximal systolic closure) and coaptation distance (distance between the annular plane and point of coaptation) in the 4-chamber view [38]. A tenting area >1 cm2 and coaptation depth <8 mm are associated with severe TR [39].
Right heart catheterization should be considered to measure pulmonary artery pressures when laminar TR precludes reliable Doppler-derived estimation. Cardiac magnetic resonance (CMR) provides more accurate evaluation of the RV complementary information to echocardiography, including calculation of regurgitant volume and fraction, mean and peak velocity or transvalvular gradient [43]. The regurgitant orifice is estimated by cine imaging through the leaflet tips in systole or by visualization of the flow jet in cross-section through plane phase-contrast velocity mapping. The regurgitant volume is estimated by calculating RV stroke volume and pulmonary forward flow. If TR is the only valve lesion, the difference between right and left ventricular stroke volumes may be used to estimate regurgitant volume [44].
Multislice computed tomography offers detailed anatomical evaluation of the TV due to its excellent spatial resolution and is especially useful in patients with complex congenital heart disease [45]. This technique involves the use of ionizing radiation and should only be used when the same amount of information cannot be obtained using non-radiation techniques.
Intraoperative echocardiographic assessment
The diagnosis of TR and decision to intervene must be made preoperatively. Severity of TR is influenced by fluid loading and the prevailing intraoperative conditions, including the effects of anaesthesia. Although intraoperative transoesophageal echocardiography is essential for the assessment of mitral and/or aortic valve dysfunction, it may also underestimate the severity of TR and lead to incorrect decisions regarding the need for intervention [46]. Hence, the recent trend towards greater reliance on tricuspid annular diameter (>40 mm or >21 mm/m2 in the 4-chamber view) to determine whether a concomitant tricuspid annuloplasty is indicated.
EVALUATING RIGHT VENTRICULAR FUNCTION
Despite the limitations of 2D echocardiography in evaluating RV function because of geometric assumptions [47], complete assessment of RV geometry and function is usually achievable using 3D echocardiography and CMR. 3D echocardiography correlates well with CMR and has less propensity to underestimate end-diastolic and end-systolic volumes and superior reproducibility to 2D imaging [48]. However, 3D-derived RV volumes are slightly smaller than those obtained with CMR with a lower reference limit of 44% for RV ejection fraction, and upper limits of 89 ml/m2 and 45 ml/m2 for RV end-diastolic and end-systolic volumes, respectively [49].
In clinical practice, the simplest method used to screen RV dilatation is by measurement of ventricular (end-diastolic and end-systolic) areas and linear dimensions (basal and mid-cavity plus RV outflow tract proximal and distal diameters) in end diastole [49]. Adjustment of the apical 4-chamber view by rotation of the probe over the cardiac apex is important to avoid foreshortening and derive the maximal RV diameter. Basal diameter >42 mm, mid-cavity diameter >33 mm or apex-to-base length >86 mm indicate RV enlargement [49]. Qualitative assessment of RV function may be derived by geometric evaluation of the inter-ventricular septum whose patterns of movement in systole and diastole can help distinguish between volume and pressure overload [50]. In pure volume overload (as in severe TR), the septum flattens in diastole and pushes against the left ventricle as a result of increased RV end-diastolic pressure. In systole, left ventricular pressure remains higher than that in the RV and the septum regains its normal configuration.
The fractional area change is the percentage change in RV chamber area during the cardiac cycle measured from the apical 4-chamber view. Fractional area change (normal >35%) correlates with RV ejection fraction measured using CMR [49]. The rate of RV pressure rise in systole (RV dP/dt) provides an estimate of RV systolic function and can be derived from the descending slope of the TR continuous-wave Doppler signal, a value <400 mmHg/s indicates RV dysfunction [49].
Tricuspid annular plane systolic excursion (TAPSE) assesses the degree of tricuspid annular displacement towards the apex and accurately reflects RV longitudinal function [51]. Current ESC/EACTS guidelines recommend the use of tricuspid annular plane systolic excursion (<15 mm), tricuspid annular systolic velocity (<11 cm/s) and RV end-systolic area (>20 cm2) to identify patients with RV dysfunction [8]. However, these values may underestimate the extent of RV dysfunction in the presence of severe TR with volume overload and should be interpreted with caution.
CMR has been considered the gold standard technique to evaluate the left (LV) and right ventricular (RV) volumes and ejection fraction, and both parameters independently predict significant residual TR after surgery [52]. Although there are no defined thresholds in terms of RV volumes or ejection fraction to indicate surgery in patients with TR, an RV end-diastolic volume ≥64 ml/m2 predicts RV dysfunction at follow-up.
INDICATIONS FOR TRICUSPID VALVE INTERVENTION
Although significant TR with progressive RV dilatation and dysfunction may remain clinically silent for a prolonged period and compromise individual patient outcome, the timing of surgical intervention for TR remains controversial [38]. In a recent meta-analysis, Pagnesi et al. [53] demonstrated that concomitant TV repair at the time of left-sided valve surgery is associated with reduced cardiovascular mortality and improved TR-related outcomes (as assessed by echocardiography) at follow-up.
Both European (ESC/EACTS) and American (AHA/ACC) guidelines state that isolated TV surgery is indicated in symptomatic patients with severe primary TR (Class I) and should be considered in asymptomatic or mildly symptomatic patients with RV enlargement or deteriorating RV function (Class IIa) [8, 9]. Although such patients respond well to diuretic therapy, delayed surgery is likely to result in irreversible RV damage, organ failure and poor results of later surgical intervention.
TV surgery is recommended for patients with severe TR undergoing left-sided valve surgery, irrespective of symptoms (Class I). Although there is no specific evidence to confirm that mild TR progresses to severe TR with or without repair, TV surgery should be considered (Class IIa) in patients with mild/moderate secondary TR and/or significant annular dilatation (≥40 mm or 21 mm/m2) (Class IIa) [8, 9].
Early surgery should also be considered for severe TR occurring late after left-sided valve surgery (with or without original TV intervention) (Class IIa) in symptomatic patients and in those who are asymptomatic but with progressive RV dilatation or dysfunction (unless severe right/left ventricular dysfunction or pulmonary vascular disease preclude surgery). Late referral is associated with poor outcomes, because RV dysfunction is already established in many patients [54].
SURGICAL TECHNIQUES
The principles of TV reconstruction are identical to those for mitral valve repair—restoration of full leaflet mobility, correction of prolapse, provision of a large leaflet coaptation surface and annular stabilization.
Access to the TV is usually via median sternotomy (although right thoracotomy may be better for redo surgery and some groups routinely employ minimally invasive right thoracotomy) [55]. TV surgery is usually performed under cardioplegia, although beating-heart surgery (even for first-time operations) may prevent atrioventricular block and reduce ischaemic time (both of which may be of particular value in high-risk patients undergoing TV replacement late after left-sided valve surgery).
Tricuspid annuloplasty techniques: sutures, rings and bands
Annuloplasty techniques are well established and share the common goal of narrowing the valve orifice to achieve leaflet coaptation [56, 57]. The De Vega annuloplasty is still used by many surgeons and consists of a double circular suture of the anterior and posterior annulus to narrow the orifice to the desired dimension, and several modifications of the technique are in use [58, 59]. Avoidance of the use of prosthetic material is an advantage, particularly in cases of active endocarditis, although the technique does not restore normal annular shape. Recently, the concept of TV orifice index to optimize TV annular reduction has been developed [60].
A variety of prosthetic oval-shaped rings have therefore been developed to replicate the systolic configuration of the normal tricuspid orifice. The prosthetic ring should be carefully sized to correspond to leaflet and body surface area, but it should be further downsized if there is severe leaflet tethering.
Sutures must be placed in the annulus, 1–2 mm beyond the leaflet hinge line. Care should be taken to avoid the adjacent aortic valve and right coronary artery, and the last sutures should be placed in the anteroseptal commissure (right fibrous trigone) and in the middle of the septal annulus to avoid injury to the bundle of His.
Additional procedures (e.g. anterior leaflet enlargement and artificial polytetrafluoroethylene chordae) may be useful in specific settings and when the valve is significantly deformed [61]. All techniques should be adapted in the presence of pacemaker leads according to anatomy, the patient’s condition and the surgeon’s experience.
Tricuspid valve replacement
The gold standard for the surgical treatment of secondary TR is TV annuloplasty. However, the results are very poor in patients with severe RV dysfunction, very large annuli and significant leaflet tenting (precise measurements poorly defined) who are probably better served with valve replacement.
Accurate prosthesis sizing is essential to not distort the right coronary artery. TV replacement is usually performed using multiple pledgeted annular sutures. Sutures in the septal portion (between the middle of the septal leaflet and the anteroseptal commissure) should be placed through leaflet tissue (with preservation of the septal leaflet) to avoid injury to the bundle of His. The use of non-everting pledgeted sutures (pledgets placed on the ventricular aspect of the leaflet tissue) is recommended.
Results
TV repair has lower perioperative mortality than valve replacement and is generally the preferred option. Mortality is low after annuloplasty for uncomplicated primary TR, but outcomes depend on aetiology, RV function and patient risk profile. Repair for secondary TR at the time of left-sided valve surgery has no impact on mortality, unless there is severe heart failure and/or significant RV dilatation or dysfunction.
In contrast, repeat surgery for TR late after initial mitral (or aortic) valve surgery is associated with higher morbidity and mortality, reflecting the overall clinical condition of the patient (including age and the number of previous cardiac interventions) [54]. Perioperative mortality rates up to 30% have been reported, even from experienced centres [62], and long-term results may also be poor due to associate irreversible RV or left ventricular dysfunction.
Suture and ring annuloplasty are associated with good long-term outcomes. Rates of late recurrent TR are higher following the original De Vega procedure [55], with conflicting evidence concerning long-term survival [7], although modifications of the original suture annuloplasty technique have been associated with encouraging results [5]. In a recent comparison, long-term mortality was equivalent following the ring or the De Vega suture annuloplasty in patients undergoing TV repair at the time of mitral valve surgery and predicted by the presence of diabetes mellitus, baseline RV dysfunction and older age. The rates of durable repair and recurrent TR were similar over long-term follow-up [63]. Rigid ring dehiscence is also a potential late complication [64].
Outcomes with mechanical and bioprosthetic valves are similar if TV replacement is necessary [65], although the use of a bioprosthetic valve is currently preferred (perhaps reflecting the emerging possibility of percutaneous valve-in-valve implantation for late prosthetic valve degeneration) [66].
Ten-year survival rate ranges from 30% to 50%, and late mortality is predicted by preoperative functional class, the LV and RV function and prosthesis-related complications [62, 67, 68].
Transcatheter interventions
New transcatheter solutions for the treatment of TR are now emerging, most of which are still in development or in the initial phase of clinical application. Some devices mimic leaflet edge-to-edge repair (MitraClip), whereas others replicate partial suture annuloplasty (Mitralign, 4-Tech).
Experience with these devices is extremely preliminary, and the safety and efficacy of the devices are yet to be fully demonstrated. A role in patients with less-than-severe secondary TR due to left-sided valve disease therefore seems unlikely for the foreseeable future. Most patients will remain surgical candidates, because both left- and right-sided VHD will require treatment using the most effective surgical approaches. On the other hand, transcatheter options are likely to be highly appropriate in high-risk or inoperable patients with functional mitral and TR, where percutaneous treatment to both valves could be considered simultaneously or using a staged approach [69].
Late TR following previous left-sided valve surgery in high-risk patients (e.g. with renal and/or hepatic impairment, and severe RV dysfunction) might potentially benefit from less invasive transcatheter therapy [24, 70].
Finally, it should be recognized that current transcatheter devices are unable to provide complete durable correction of TR in its advanced stages with severe annular dilatation and leaflet tethering due to significant RV dilatation and dysfunction.
CONCLUSIONS
Primary TR is rare and caused by the abnormalities of the TV apparatus. Secondary TR is far more frequent and related to RV pressure and/or volume overload and dysfunction, resulting in annular dilatation and/or leaflet tethering.
The pathophysiology of TR is complex. A systematic multimodality approach to diagnosis and assessment (based not only on TR severity but also on assessing annular size and RV function) is, therefore, essential.
Clinical presentation ranges from subtle manifestations in the asymptomatic patient to overt right heart failure. Diuretic therapy rapidly decreases volume overload but may mask symptoms and signs of RV dysfunction.
Physical examination is a key to diagnosis, and echocardiography remains the principal imaging tool. CMR and invasive haemodynamic assessment provide essential supplementary information.
The severity of TR is influenced by loading conditions and usually underestimated by transoesophageal echocardiography performed under general anaesthesia—the decision to intervene should therefore be made during preoperative assessment. If this is not possible then greater reliance should be placed upon intraoperative measurement of tricuspid annular diameter rather than intraoperative assessment of the degree of regurgitation.
TV surgery is recommended in patients with severe primary or secondary TR. Surgery should also be considered for patients with mild or moderate secondary TR and a dilated tricuspid annulus (diameter ≥40 mm in the echocardiographic 4-chamber view), who are undergoing surgery for left-sided VHD.
At this stage, TV surgery is not recommended in patients with annular dilatation but no (or minimal) TR.
Patients with severe TR after previous left-sided valve surgery need careful follow-up to detect the need for early redo surgery (or percutaneous intervention) before the development of significant RV dilatation and/or dysfunction.
Future developments in percutaneous TV intervention may offer new treatment options in selected cases.
RECOMMENDATIONS
The ESC Working Groups of Cardiovascular Surgery and Valvular Heart Disease recommend the diagnostic and management algorithms outlined in Figs 1 and 2 for patients with primary and secondary TR, respectively.
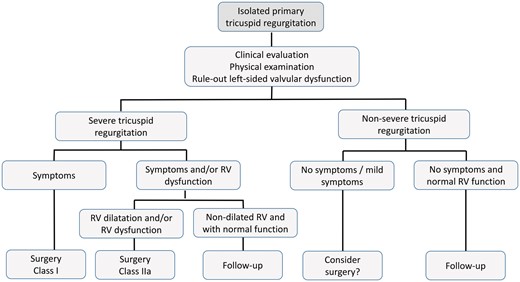
Management of primary tricuspid regurgitation. RV: right ventricular.
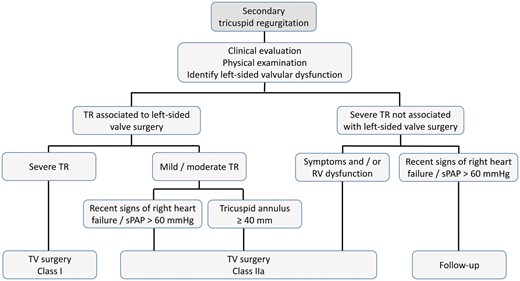
Management of secondary TR. sPAP: systolic pulmonary arterial pressure; TR: tricuspid regurgitation; TV: tricuspid valve.
Conflict of interest: none declared.