-
PDF
- Split View
-
Views
-
Cite
Cite
Marco Ranucci, Valeria Pistuddi, Giulia Pinuccia Pisani, Concetta Carlucci, Giuseppe Isgrò, Alessandro Frigiola, Giuseppe Pomè, Alessandro Giamberti, on behalf of the Surgical and Clinical Outcome Research (SCORE) Group, Retuning mortality risk prediction in paediatric cardiac surgery: the additional role of early postoperative metabolic and respiratory profile, European Journal of Cardio-Thoracic Surgery, Volume 50, Issue 4, October 2016, Pages 642–649, https://doi.org/10.1093/ejcts/ezw102
- Share Icon Share
Abstract
The existing risk stratification scores for paediatric patients undergoing cardiac surgery include the Aristotle Basic Complexity (ABC) Score, the Risk Adjustment in Congenital Heart Surgery-1 (RACHS-1) Score and the Aristotle Comprehensive Complexity (ACC) Score. They are all based on the nature of the surgical operation (ABC and RACHS-1 Scores) with possible adjustment for a number of patient conditions (ACC Score). The present study investigates if the early postoperative parameters may be used to improve the preoperative mortality risk prediction.
A retrospective study on 1392 consecutive patients aged ≤12 years old, undergoing cardiac surgery with cardiopulmonary bypass and without a residual right-to-left shunt was conducted. The ABC Score and metabolic and respiratory postoperative parameters at arrival in the intensive care unit were tested for association and discriminative power for operative mortality.
The ABC yielded a c -statistic of 0.746. Additional independent predictors of operative mortality were postoperative hypoxia and arterial blood lactates. In a multivariable model including the ABC Score, postoperative hypoxia and arterial blood lactates remained independently associated with operative mortality. A modified ABC Score was created, consisting of the ABC Score plus 1.5 points in case of postoperative hypoxia plus 1 point per each 1 mmol/l of arterial blood lactates. The new model was significantly ( P = 0.043) more discriminative than the ABC Score, with a c -statistic of 0.803.
Early postoperative respiratory and metabolic parameters increased the accuracy and discrimination of the ABC Score. An external validation is needed to confirm our results.
INTRODUCTION
Evaluating the quality of care in the management of paediatric patients with congenital heart disease is a challenging issue. The main difficulties include the great heterogeneity of the patient population (both in terms of age and diagnosis), the difficult assessment of comorbid conditions and the many different surgical approaches available [ 1 ].
Historically, the first mortality risk-scoring tools introduced in clinical practice are the Aristotle Basic Complexity (ABC) Score [ 2 ] and the Risk Adjustment in Congenital Heart Surgery-1 (RACHS-1) Score [ 3 ]. Both are based on the complexity of the surgical procedure, without taking into account the preoperative profile of the patient, and are included in the databases of the European Association for Cardio-Thoracic Surgery (EACTS) and the Society of Thoracic Surgeons (STS): RACHS-1 since 2006 and ABC since 2002. Recently, based on their databases, the EACTS and the STS introduced novel risk stratification tools, the Society of Thoracic Surgeons-European Association for Cardio-thoracic Surgery (STAT) mortality score and categories [ 1 ], which will probably become the reference tools in the future.
An attempt to adjust for the additional factors is represented by the Aristotle Comprehensive Complexity (ACC) Score, which adds further discrimination by incorporating two sorts of patient-specific complexity modifiers: procedure-dependent factors (anatomical factors, associated procedures and age at procedure) and procedure-independent factors (weight and prematurity, clinical factors, extracardiac factors and surgical factors) [ 4 ].
Several groups have evaluated the discriminatory power and the accuracy of the existing scores, finding c -statistic values ranging from 0.70 to 0.8 for the ABC Score and the RACHS-1 Score, and slightly higher values for the ACC Score [ 5–8 ].
Intraoperative factors may play an important role in changing the risk profile of paediatric patients undergoing cardiac surgery, resulting in an overall mortality risk that may differ from that assessed based on preoperative factors and the nature of the surgical procedure. This applies to both adult and paediatric patients; however, in the latter population, there is certainly a specific impact of intraoperative factors as determinants of operative mortality. This may be due to the complexity of the procedure, the impact of cardiopulmonary bypass (CPB) on small-sized patients, the need for allogeneic blood transfusions that is greater than in adults and finally, even to the possible changes in the surgical strategy as a consequence of unexpected anatomical problems. It is therefore possible that the paediatric patient, when arriving in the intensive care unit (ICU) at the end of the surgical procedure, may be different (at higher or lower mortality risk) than what expected.
The present study is aimed to verify the hypothesis that a number of metabolic and respiratory parameters collected at arrival in the ICU after cardiac surgery in paediatric patients may be associated with postoperative mortality risk. Based on this hypothesis, we aim to retune the mortality risk prediction based on the ABC Score by incorporating one or more of these early postoperative parameters into a new predictive model.
METHODS
A retrospective study on congenital heart paediatric (≤12 years) patients operated at our institution from October 2008 to December 2014. The local ethics committee approved the study and waived the need for informed consent.
Data were retrieved from our institutional database, which contains preoperative, intraoperative and postoperative data of patients undergoing cardiac surgery. Since October 2008, the database includes haemodynamic, metabolic and respiratory data collected immediately at arrival in the ICU.
Patients
The patient population considered for the present study was restricted to patients receiving cardiac surgery with CPB and without a residual right-to-left shunt. This was decided to analyse a homogeneous patient population in terms of determinants of postoperative oxygenation variables, which are affected by residual cyanotic defects, palliative surgery with a right-to-left shunt and by the deleterious effects of CPB. Other exclusion criteria were the need for extracorporeal membrane oxygenation before ICU admission and intraoperative mortality.
During the period considered, 1894 patients ≤12 years received surgery for correction or palliation of congenital heart defects. Two hundred and thirty-eight patients received surgery without CPB; 228 patients received palliative procedures with a residual right-to-left shunt. Four patients (0.2%) died during surgery and 32 (1.7%) required extracorporeal membrane oxygenation to be weaned from CPB. This patient population was excluded by the present analysis. The final patient population included 1392 subjects.
Anaesthesia, surgery and cardiopulmonary bypass
Anaesthesia was carried out according to our institutional practice. Induction of anaesthesia was achieved with an intravenous infusion of midazolam or propofol. In patients weighing <10 kg, a high-dose opioid anaesthetic (fentanyl 50 µg/kg) was used for the maintenance of anaesthesia and supplemented with midazolam and sevoflurane as tolerated. In patients with larger body weight, the maintenance of anaesthesia was guaranteed with an intravenous infusion of remifentanil and propofol with sevoflurane supplementation as required. Neuromuscular blockade was achieved with vecuronium or atracurium. All patients underwent endotracheal intubation and were mechanically ventilated. Standard monitoring was used, which included a radial or femoral artery catheter for the measurement of systemic arterial blood pressure and intermittent blood sampling, a double-lumen right internal jugular catheter, and oesophageal and rectal temperature probes.
Cardiac cannulation was performed after intravenous administration of 300 IU/kg of unfractionated heparin and only after an activated clotting time of longer than 450 s was achieved. Additional heparin boluses were used to maintain an activated clotting time in this range before and during CPB. Double venous cannulation of the superior and inferior vena cava was generally performed. The arterial cannula was placed into the ascending aorta. The CPB circuit included a hollow fibre oxygenator (Dideco D901 or D902, Sorin Group, Mirandola, Italy) with an arterial line filter and a centrifugal pump (Bio-Medicus, Medtronic, Minneapolis, MN, USA). In the blood-primed patients, the CPB circuit was primed with a solution containing red blood cells and a 4% albumin solution. The solution was titrated to reach a haematocrit value of 30% once the patient was connected to the circuit and CPB was initiated. The total priming volume varied between 350 and 450 ml. Therefore, the amount of red blood cells used in the priming solution varied according to the patient's baseline haematocrit, weight and the priming volume used. In all patients, less than a 250-ml volume of red blood cells and only one bag of stored RBCs were used for priming the circuit. Non-blood-primed patients received a 4% albumin solution for priming the CPB circuit, or crystalloid + gelatin solution in patients weighing >20 kg. CPB flow was targeted at 150 ml/kg and subsequently adjusted according to the patient's temperature.
The target patient temperature was chosen by the surgeon based on the type of surgical procedure being performed and personal preferences. All procedures were performed using a regimen of mild (32–34°C), moderate (26–31°C) or deep (20–25°C) hypothermia. Cardiac arrest was obtained and maintained using antegrade intermittent blood cardioplegia or crystalloid cardioplegia. After completion of the CPB and removal of the cannulas, heparin was reversed using protamine sulphate at a 1 : 1 ratio.
Data collection
The following data were available and used for the statistical analysis.
Preoperative data : age (months); weight (kg); height (cm); gender; serum creatinine (mg/dl); serum bilirubin (mg/dl); haematocrit (HCT, %); redo surgery.
Intraoperative data : type of surgery; CPB time (min); aortic cross-clamp time (min); type of priming solution (blood prime or crystalloid/colloid prime); lowest HCT on CPB; lowest temperature (°C) on CPB.
Postoperative data : at the arrival in the ICU, the following data were recorded: inspired oxygen fraction ; arterial oxygen tension ratio; arterial pH; arterial carbon dioxide tension ; bicarbonate ( ); arterial blood lactates (mmol/l); mean arterial pressure (mmHg); central venous pressure; heart rate (beats per minute, bpm); central temperature (°C) and haematocrit (%). The mean arterial pressure and the heart rate were not considered in this analysis, because their normal range is strongly dependent on age. The haematocrit value too was not considered here because of its dependency on the use of blood prime and therefore again on the age of the patients.
Operative mortality was defined as mortality occurring during the hospital stay or after discharge within 30 days from surgery.
Statistics
A preliminary analysis on the discriminative properties of the ABC Score was performed on our patient series, having the operative mortality as the dependent variable and the ABC Score as the predictive test. The patient population was analysed according to the ABC Score distribution, with a stratification of the mortality risk defined according to the categories previously defined in other studies [ 4 ]: low (1–5.9), mild (6–7.9), moderate (8–9.9) and high (10–15) risk. Differences in mortality among the different risk classes were analysed with a Pearson's χ2 test.
The discriminative properties of the ABC Score were explored with a receiver operating characteristic (ROC) analysis, producing the area under the curve ( c -statistics) with 95% confidence interval (CI).
The association of the parameters measured at arrival in the ICU with the operative mortality risk was investigated with a univariate analysis using parametric (Student's t -test) and non-parametric (Mann–Whitney U -test) tests for normally and non-normally distributed variables, with the normality of distribution checked using a Kolmogorov–Smirnov test. A subsequent multivariable analysis (stepwise forward logistic regression) was applied to all the factors having an association with operative mortality at a P- value of 0.1 or less, and the independent risk factors for operative mortality were consequently identified. For each independent risk factor, the discriminative ability was tested with an ROC analysis, producing the areas under the curve (AUC) with 95% CI. Adequate cut-off values were identified according to the sensitivity and specificity properties (Youden's index).
The independent predictors were subsequently included together with the ABC Score in a multivariable model (stepwise forward logistic regression analysis). The odds ratios for mortality in this combined model were used to convert these values into corresponding risk score points for adjustment of the ABC Score. A final modified ABC Score (modABC Score) was therefore created and tested for discriminative properties with an ROC analysis, testing if the modABC Score had significantly higher discriminative power than the ABC Score according to the non-parametric method described by DeLong et al. [ 9 ].
The relationship between the ABC Score, the modABC Score and the observed mortality was graphically expressed as logistic regression functions with 95% CI.
Finally, adequate reclassification analyses were performed, to check how many patients were actually reclassified into the four risk categories previously identified.
The whole analysis is based on a development series, without internal validation as obtainable by splitting the patient population into a development and validation series. Owing to the anticipated few mortality events, the development series would suffer from overfitting in the multivariable model.
All the statistical analyses were performed with computerized packages (SPSS 13.0, IBM, Chicago, IL, USA and MedCalc, MedCalc Software, Ostend, Belgium).
RESULTS
The general characteristics of the patient population and of the surgical procedures are presented in Table 1 . Thirty-two (2.3%) patients fulfilled the criteria for operative mortality. Overall, the ABC Score was significantly ( P = 0.001) associated with the operative mortality risk (Fig. 1 ) and the mortality rate was significantly ( P = 0.001) increased for increasing severity classes of the ABC Score (Table 2 ). However, no significant differences were found in mortality rates between class 1 and classes 2 and 3, and between class 2 and class 3.
Parameter . | Median (IQ range) or number (%) . |
---|---|
Age (months) | 12 (5–60) |
Weight (kg) | 9 (5.1–18) |
Serum creatinine (mg/dl) | 0.3 (0.3–0.4) |
Serum bilirubin (mg/dl) | 0.5 (0.3–0.6) |
Haematocrit (%) | 36 (33–39.5) |
Type of surgical operation | |
Patent foramen ovale, direct closure | 2 (0.1) |
Atrial septal defect, direct closure | 12 (0.9) |
Atrial septal defect, patch closure | 150 (10.8) |
Single atrium patch septum reconstruction | 2 (0.1) |
Ventricular septal defect, direct closure | 25 (1.8) |
Ventricular septal defect, patch closure | 195 (14) |
Multiple ventricular septal defects, correction | 8 (0.6) |
Ventricular septal defect, enlargement | 2 (0.1) |
Complete atrioventricular canal, correction | 56 (4) |
Intermediate atrioventricular canal, correction | 9 (0.6) |
Partial atrioventricular canal, correction | 39 (2.8) |
Aorto-pulmonary window, correction | 3 (0.2) |
Truncus arteriosus, correction | 35 (2.5) |
Partial anomalous pulmonary venous return, correction | 15 (1.1) |
Scimitar syndrome, correction | 1 (0.1) |
Total anomalous pulmonary venous return, correction | 21 (1.5) |
Cor triatriatum, correction | 8 (0.6) |
Pulmonary venous stenosis, repair | 5 (0.4) |
Tetralogy of Fallot, repair, no ventriculotomy | 2 (0.1) |
Tetralogy of Fallot, repair, ventriculotomy, no transannular patch | 39 (2.8) |
Tetralogy of Fallot, repair, ventriculotomy, transannular patch | 76 (5.5) |
Tetralogy of Fallot, repair, right ventricle–pulmonary artery conduit | 3 (0.2) |
Tetralogy of Fallot + atrioventricular canal, correction | 6 (0.4) |
Tetralogy of Fallot, absent pulmonary valve, correction | 4 (0.3) |
Pulmonary atresia with ventricular septal defect, correction | 5 (0.4) |
Pulmonary atresia with ventricular septal defect and major systemic–pulmonary collaterals, correction | 9 (0.6) |
Major systemic–pulmonary collaterals unifocalization | 7 (0.5) |
Tricuspid valve repair | 16 (1.2) |
Tricuspid valve replacement | 3 (0.2) |
Right ventricular outflow obstruction, repair | 19 (1.4) |
Pulmonary artery trunk reconstruction | 9 (0.6) |
Pulmonary artery branches reconstruction | 5 (0.4) |
Double-chamber right ventricle, repair | 1 (0.1) |
Conduit, replacement | 28 (2) |
Pulmonary valve, repair | 7 (0.5) |
Pulmonary valve, replacement | 7 (0.5) |
Conduit placement, right ventricle to pulmonary artery | 22 (1.6) |
Aortic valve repair | 29 (2.1) |
Aortic valve replacement, mechanical prosthesis | 4 (0.3) |
Aortic valve replacement, homograft | 1 (0.1) |
Aortic root replacement, valve sparing | 3 (0.2) |
Ross operation | 17 (1.2) |
Ross-Konno operation | 5 (0.4) |
Aortic stenosis, subvalvar, correction | 76 (5.5) |
Aortic stenosis, supravalvar, correction | 12 (0.9) |
Mitral valve, repair | 41 (2.9) |
Mitral stenosis, supravalvar mitral ring repair | 10 (0.7) |
Mitral valve replacement | 27 (1.9) |
Fontan operation, external conduit, non-fenestrated | 57 (4.1) |
Repair of the congenitally corrected transposition of the great arteries, ventricular septal defect closure | 3 (0.2) |
Arterial switch | 108 (7.8) |
Arterial switch and ventricular septal defect closure | 53 (3.8) |
Senning operation | 4 (0.3) |
Rastelli operation | 20 (1.4) |
Double outlet right ventricle, intraventricular tunnel repair | 22 (1.6) |
Anomalous coronary artery, correction | 7 (0.5) |
Coronary artery fistula ligation | 2 (0.1) |
Coronary artery bypass graft | 4 (0.3) |
Aortic arch repair | 4 (0.3) |
Interrupted aortic arch repair | 16 (1.1) |
Patent ductus arteriosus closure (surgical) | 1 (0.1) |
Pulmonary artery sling repair | 1 (0.1) |
Aortic aneurysm repair | 2 (0.1) |
Cardiac tumour resection | 3 (0.2) |
Pulmonary embolectomy | 2 (0.1) |
Aortic coarctation + ventricular septal defect repair | 2 (0.1) |
Aortic arch + ventricular septal defect repair | 1 (0.1) |
Ebstein's repair | 1 (0.1) |
Redo surgery | 229 (16.4) |
Aristotle Basic Complexity Score | 7.5 (6–9) |
Cardiopulmonary bypass time (min) | 77 (50–123) |
Lowest haematocrit on CPB (%) | 27 (25–29) |
Lowest temperature on CPB (°C) | 32 (30–33) |
Blood cardioplegia | 668 (48) |
Blood prime | 960 (69) |
Parameter . | Median (IQ range) or number (%) . |
---|---|
Age (months) | 12 (5–60) |
Weight (kg) | 9 (5.1–18) |
Serum creatinine (mg/dl) | 0.3 (0.3–0.4) |
Serum bilirubin (mg/dl) | 0.5 (0.3–0.6) |
Haematocrit (%) | 36 (33–39.5) |
Type of surgical operation | |
Patent foramen ovale, direct closure | 2 (0.1) |
Atrial septal defect, direct closure | 12 (0.9) |
Atrial septal defect, patch closure | 150 (10.8) |
Single atrium patch septum reconstruction | 2 (0.1) |
Ventricular septal defect, direct closure | 25 (1.8) |
Ventricular septal defect, patch closure | 195 (14) |
Multiple ventricular septal defects, correction | 8 (0.6) |
Ventricular septal defect, enlargement | 2 (0.1) |
Complete atrioventricular canal, correction | 56 (4) |
Intermediate atrioventricular canal, correction | 9 (0.6) |
Partial atrioventricular canal, correction | 39 (2.8) |
Aorto-pulmonary window, correction | 3 (0.2) |
Truncus arteriosus, correction | 35 (2.5) |
Partial anomalous pulmonary venous return, correction | 15 (1.1) |
Scimitar syndrome, correction | 1 (0.1) |
Total anomalous pulmonary venous return, correction | 21 (1.5) |
Cor triatriatum, correction | 8 (0.6) |
Pulmonary venous stenosis, repair | 5 (0.4) |
Tetralogy of Fallot, repair, no ventriculotomy | 2 (0.1) |
Tetralogy of Fallot, repair, ventriculotomy, no transannular patch | 39 (2.8) |
Tetralogy of Fallot, repair, ventriculotomy, transannular patch | 76 (5.5) |
Tetralogy of Fallot, repair, right ventricle–pulmonary artery conduit | 3 (0.2) |
Tetralogy of Fallot + atrioventricular canal, correction | 6 (0.4) |
Tetralogy of Fallot, absent pulmonary valve, correction | 4 (0.3) |
Pulmonary atresia with ventricular septal defect, correction | 5 (0.4) |
Pulmonary atresia with ventricular septal defect and major systemic–pulmonary collaterals, correction | 9 (0.6) |
Major systemic–pulmonary collaterals unifocalization | 7 (0.5) |
Tricuspid valve repair | 16 (1.2) |
Tricuspid valve replacement | 3 (0.2) |
Right ventricular outflow obstruction, repair | 19 (1.4) |
Pulmonary artery trunk reconstruction | 9 (0.6) |
Pulmonary artery branches reconstruction | 5 (0.4) |
Double-chamber right ventricle, repair | 1 (0.1) |
Conduit, replacement | 28 (2) |
Pulmonary valve, repair | 7 (0.5) |
Pulmonary valve, replacement | 7 (0.5) |
Conduit placement, right ventricle to pulmonary artery | 22 (1.6) |
Aortic valve repair | 29 (2.1) |
Aortic valve replacement, mechanical prosthesis | 4 (0.3) |
Aortic valve replacement, homograft | 1 (0.1) |
Aortic root replacement, valve sparing | 3 (0.2) |
Ross operation | 17 (1.2) |
Ross-Konno operation | 5 (0.4) |
Aortic stenosis, subvalvar, correction | 76 (5.5) |
Aortic stenosis, supravalvar, correction | 12 (0.9) |
Mitral valve, repair | 41 (2.9) |
Mitral stenosis, supravalvar mitral ring repair | 10 (0.7) |
Mitral valve replacement | 27 (1.9) |
Fontan operation, external conduit, non-fenestrated | 57 (4.1) |
Repair of the congenitally corrected transposition of the great arteries, ventricular septal defect closure | 3 (0.2) |
Arterial switch | 108 (7.8) |
Arterial switch and ventricular septal defect closure | 53 (3.8) |
Senning operation | 4 (0.3) |
Rastelli operation | 20 (1.4) |
Double outlet right ventricle, intraventricular tunnel repair | 22 (1.6) |
Anomalous coronary artery, correction | 7 (0.5) |
Coronary artery fistula ligation | 2 (0.1) |
Coronary artery bypass graft | 4 (0.3) |
Aortic arch repair | 4 (0.3) |
Interrupted aortic arch repair | 16 (1.1) |
Patent ductus arteriosus closure (surgical) | 1 (0.1) |
Pulmonary artery sling repair | 1 (0.1) |
Aortic aneurysm repair | 2 (0.1) |
Cardiac tumour resection | 3 (0.2) |
Pulmonary embolectomy | 2 (0.1) |
Aortic coarctation + ventricular septal defect repair | 2 (0.1) |
Aortic arch + ventricular septal defect repair | 1 (0.1) |
Ebstein's repair | 1 (0.1) |
Redo surgery | 229 (16.4) |
Aristotle Basic Complexity Score | 7.5 (6–9) |
Cardiopulmonary bypass time (min) | 77 (50–123) |
Lowest haematocrit on CPB (%) | 27 (25–29) |
Lowest temperature on CPB (°C) | 32 (30–33) |
Blood cardioplegia | 668 (48) |
Blood prime | 960 (69) |
CPB: cardiopulmonary bypass; IQ: interquartile.
Parameter . | Median (IQ range) or number (%) . |
---|---|
Age (months) | 12 (5–60) |
Weight (kg) | 9 (5.1–18) |
Serum creatinine (mg/dl) | 0.3 (0.3–0.4) |
Serum bilirubin (mg/dl) | 0.5 (0.3–0.6) |
Haematocrit (%) | 36 (33–39.5) |
Type of surgical operation | |
Patent foramen ovale, direct closure | 2 (0.1) |
Atrial septal defect, direct closure | 12 (0.9) |
Atrial septal defect, patch closure | 150 (10.8) |
Single atrium patch septum reconstruction | 2 (0.1) |
Ventricular septal defect, direct closure | 25 (1.8) |
Ventricular septal defect, patch closure | 195 (14) |
Multiple ventricular septal defects, correction | 8 (0.6) |
Ventricular septal defect, enlargement | 2 (0.1) |
Complete atrioventricular canal, correction | 56 (4) |
Intermediate atrioventricular canal, correction | 9 (0.6) |
Partial atrioventricular canal, correction | 39 (2.8) |
Aorto-pulmonary window, correction | 3 (0.2) |
Truncus arteriosus, correction | 35 (2.5) |
Partial anomalous pulmonary venous return, correction | 15 (1.1) |
Scimitar syndrome, correction | 1 (0.1) |
Total anomalous pulmonary venous return, correction | 21 (1.5) |
Cor triatriatum, correction | 8 (0.6) |
Pulmonary venous stenosis, repair | 5 (0.4) |
Tetralogy of Fallot, repair, no ventriculotomy | 2 (0.1) |
Tetralogy of Fallot, repair, ventriculotomy, no transannular patch | 39 (2.8) |
Tetralogy of Fallot, repair, ventriculotomy, transannular patch | 76 (5.5) |
Tetralogy of Fallot, repair, right ventricle–pulmonary artery conduit | 3 (0.2) |
Tetralogy of Fallot + atrioventricular canal, correction | 6 (0.4) |
Tetralogy of Fallot, absent pulmonary valve, correction | 4 (0.3) |
Pulmonary atresia with ventricular septal defect, correction | 5 (0.4) |
Pulmonary atresia with ventricular septal defect and major systemic–pulmonary collaterals, correction | 9 (0.6) |
Major systemic–pulmonary collaterals unifocalization | 7 (0.5) |
Tricuspid valve repair | 16 (1.2) |
Tricuspid valve replacement | 3 (0.2) |
Right ventricular outflow obstruction, repair | 19 (1.4) |
Pulmonary artery trunk reconstruction | 9 (0.6) |
Pulmonary artery branches reconstruction | 5 (0.4) |
Double-chamber right ventricle, repair | 1 (0.1) |
Conduit, replacement | 28 (2) |
Pulmonary valve, repair | 7 (0.5) |
Pulmonary valve, replacement | 7 (0.5) |
Conduit placement, right ventricle to pulmonary artery | 22 (1.6) |
Aortic valve repair | 29 (2.1) |
Aortic valve replacement, mechanical prosthesis | 4 (0.3) |
Aortic valve replacement, homograft | 1 (0.1) |
Aortic root replacement, valve sparing | 3 (0.2) |
Ross operation | 17 (1.2) |
Ross-Konno operation | 5 (0.4) |
Aortic stenosis, subvalvar, correction | 76 (5.5) |
Aortic stenosis, supravalvar, correction | 12 (0.9) |
Mitral valve, repair | 41 (2.9) |
Mitral stenosis, supravalvar mitral ring repair | 10 (0.7) |
Mitral valve replacement | 27 (1.9) |
Fontan operation, external conduit, non-fenestrated | 57 (4.1) |
Repair of the congenitally corrected transposition of the great arteries, ventricular septal defect closure | 3 (0.2) |
Arterial switch | 108 (7.8) |
Arterial switch and ventricular septal defect closure | 53 (3.8) |
Senning operation | 4 (0.3) |
Rastelli operation | 20 (1.4) |
Double outlet right ventricle, intraventricular tunnel repair | 22 (1.6) |
Anomalous coronary artery, correction | 7 (0.5) |
Coronary artery fistula ligation | 2 (0.1) |
Coronary artery bypass graft | 4 (0.3) |
Aortic arch repair | 4 (0.3) |
Interrupted aortic arch repair | 16 (1.1) |
Patent ductus arteriosus closure (surgical) | 1 (0.1) |
Pulmonary artery sling repair | 1 (0.1) |
Aortic aneurysm repair | 2 (0.1) |
Cardiac tumour resection | 3 (0.2) |
Pulmonary embolectomy | 2 (0.1) |
Aortic coarctation + ventricular septal defect repair | 2 (0.1) |
Aortic arch + ventricular septal defect repair | 1 (0.1) |
Ebstein's repair | 1 (0.1) |
Redo surgery | 229 (16.4) |
Aristotle Basic Complexity Score | 7.5 (6–9) |
Cardiopulmonary bypass time (min) | 77 (50–123) |
Lowest haematocrit on CPB (%) | 27 (25–29) |
Lowest temperature on CPB (°C) | 32 (30–33) |
Blood cardioplegia | 668 (48) |
Blood prime | 960 (69) |
Parameter . | Median (IQ range) or number (%) . |
---|---|
Age (months) | 12 (5–60) |
Weight (kg) | 9 (5.1–18) |
Serum creatinine (mg/dl) | 0.3 (0.3–0.4) |
Serum bilirubin (mg/dl) | 0.5 (0.3–0.6) |
Haematocrit (%) | 36 (33–39.5) |
Type of surgical operation | |
Patent foramen ovale, direct closure | 2 (0.1) |
Atrial septal defect, direct closure | 12 (0.9) |
Atrial septal defect, patch closure | 150 (10.8) |
Single atrium patch septum reconstruction | 2 (0.1) |
Ventricular septal defect, direct closure | 25 (1.8) |
Ventricular septal defect, patch closure | 195 (14) |
Multiple ventricular septal defects, correction | 8 (0.6) |
Ventricular septal defect, enlargement | 2 (0.1) |
Complete atrioventricular canal, correction | 56 (4) |
Intermediate atrioventricular canal, correction | 9 (0.6) |
Partial atrioventricular canal, correction | 39 (2.8) |
Aorto-pulmonary window, correction | 3 (0.2) |
Truncus arteriosus, correction | 35 (2.5) |
Partial anomalous pulmonary venous return, correction | 15 (1.1) |
Scimitar syndrome, correction | 1 (0.1) |
Total anomalous pulmonary venous return, correction | 21 (1.5) |
Cor triatriatum, correction | 8 (0.6) |
Pulmonary venous stenosis, repair | 5 (0.4) |
Tetralogy of Fallot, repair, no ventriculotomy | 2 (0.1) |
Tetralogy of Fallot, repair, ventriculotomy, no transannular patch | 39 (2.8) |
Tetralogy of Fallot, repair, ventriculotomy, transannular patch | 76 (5.5) |
Tetralogy of Fallot, repair, right ventricle–pulmonary artery conduit | 3 (0.2) |
Tetralogy of Fallot + atrioventricular canal, correction | 6 (0.4) |
Tetralogy of Fallot, absent pulmonary valve, correction | 4 (0.3) |
Pulmonary atresia with ventricular septal defect, correction | 5 (0.4) |
Pulmonary atresia with ventricular septal defect and major systemic–pulmonary collaterals, correction | 9 (0.6) |
Major systemic–pulmonary collaterals unifocalization | 7 (0.5) |
Tricuspid valve repair | 16 (1.2) |
Tricuspid valve replacement | 3 (0.2) |
Right ventricular outflow obstruction, repair | 19 (1.4) |
Pulmonary artery trunk reconstruction | 9 (0.6) |
Pulmonary artery branches reconstruction | 5 (0.4) |
Double-chamber right ventricle, repair | 1 (0.1) |
Conduit, replacement | 28 (2) |
Pulmonary valve, repair | 7 (0.5) |
Pulmonary valve, replacement | 7 (0.5) |
Conduit placement, right ventricle to pulmonary artery | 22 (1.6) |
Aortic valve repair | 29 (2.1) |
Aortic valve replacement, mechanical prosthesis | 4 (0.3) |
Aortic valve replacement, homograft | 1 (0.1) |
Aortic root replacement, valve sparing | 3 (0.2) |
Ross operation | 17 (1.2) |
Ross-Konno operation | 5 (0.4) |
Aortic stenosis, subvalvar, correction | 76 (5.5) |
Aortic stenosis, supravalvar, correction | 12 (0.9) |
Mitral valve, repair | 41 (2.9) |
Mitral stenosis, supravalvar mitral ring repair | 10 (0.7) |
Mitral valve replacement | 27 (1.9) |
Fontan operation, external conduit, non-fenestrated | 57 (4.1) |
Repair of the congenitally corrected transposition of the great arteries, ventricular septal defect closure | 3 (0.2) |
Arterial switch | 108 (7.8) |
Arterial switch and ventricular septal defect closure | 53 (3.8) |
Senning operation | 4 (0.3) |
Rastelli operation | 20 (1.4) |
Double outlet right ventricle, intraventricular tunnel repair | 22 (1.6) |
Anomalous coronary artery, correction | 7 (0.5) |
Coronary artery fistula ligation | 2 (0.1) |
Coronary artery bypass graft | 4 (0.3) |
Aortic arch repair | 4 (0.3) |
Interrupted aortic arch repair | 16 (1.1) |
Patent ductus arteriosus closure (surgical) | 1 (0.1) |
Pulmonary artery sling repair | 1 (0.1) |
Aortic aneurysm repair | 2 (0.1) |
Cardiac tumour resection | 3 (0.2) |
Pulmonary embolectomy | 2 (0.1) |
Aortic coarctation + ventricular septal defect repair | 2 (0.1) |
Aortic arch + ventricular septal defect repair | 1 (0.1) |
Ebstein's repair | 1 (0.1) |
Redo surgery | 229 (16.4) |
Aristotle Basic Complexity Score | 7.5 (6–9) |
Cardiopulmonary bypass time (min) | 77 (50–123) |
Lowest haematocrit on CPB (%) | 27 (25–29) |
Lowest temperature on CPB (°C) | 32 (30–33) |
Blood cardioplegia | 668 (48) |
Blood prime | 960 (69) |
CPB: cardiopulmonary bypass; IQ: interquartile.
. | Risk classes . | |||
---|---|---|---|---|
Low ( N = 249) . | Mild ( N = 463) . | Moderate ( N = 354) . | High ( N = 294) . | |
ABC Score | 3–5.9 | 6–7.9 | 8–9.9 | 10–15 |
Mortality rate (%) | 0.4 | 1.1 | 1.9 | 6.1 |
χ2 for between-groups difference: 27.2; P = 0.001 |
. | Risk classes . | |||
---|---|---|---|---|
Low ( N = 249) . | Mild ( N = 463) . | Moderate ( N = 354) . | High ( N = 294) . | |
ABC Score | 3–5.9 | 6–7.9 | 8–9.9 | 10–15 |
Mortality rate (%) | 0.4 | 1.1 | 1.9 | 6.1 |
χ2 for between-groups difference: 27.2; P = 0.001 |
ABC: Aristotle Basic Complexity.
. | Risk classes . | |||
---|---|---|---|---|
Low ( N = 249) . | Mild ( N = 463) . | Moderate ( N = 354) . | High ( N = 294) . | |
ABC Score | 3–5.9 | 6–7.9 | 8–9.9 | 10–15 |
Mortality rate (%) | 0.4 | 1.1 | 1.9 | 6.1 |
χ2 for between-groups difference: 27.2; P = 0.001 |
. | Risk classes . | |||
---|---|---|---|---|
Low ( N = 249) . | Mild ( N = 463) . | Moderate ( N = 354) . | High ( N = 294) . | |
ABC Score | 3–5.9 | 6–7.9 | 8–9.9 | 10–15 |
Mortality rate (%) | 0.4 | 1.1 | 1.9 | 6.1 |
χ2 for between-groups difference: 27.2; P = 0.001 |
ABC: Aristotle Basic Complexity.

Association between Aristotle Basic Complexity (ABC) Score and mortality risk, with receiver operating characteristic curve (data in the text). Dashed lines are the 95% confidence interval.
The discriminative properties of the ABC Score were moderately good (AUC 0.746, 95% CI 0.722–0.768, Fig. 1 ).
The values of metabolic and respiratory variables at arrival in the ICU are reported in Table 3 , for survivors and non-survivors. Among these parameters, only the and the arterial blood lactates were independent predictors of operative mortality. were preliminarily excluded from the multivariable analysis, due to their intercorrelation with the combined index . The had a modest discriminative power (AUC = 0.656), with a cut-off value at 209 (sensitivity 53%, specificity 75%, positive predictive value 6.2%, negative predictive value 98.1% and relative risk for mortality 3.2). A second cut-off at a value of 100 was tested, but the relative risk for mortality (3.3) did not increase.
Variable . | Survivors ( N = 1360) . | Non-survivors ( N = 32) . | P -value . |
---|---|---|---|
pH | 7.4 (7.4–7.5) | 7.4 (7.3–7.47) | 0.081 |
0.51 (0.48–0.59) | 0.56 (0.48–0.67) | 0.062 | |
(mmHg) | 204 (112–258) | 103 (62–192) | 0.001 |
399 (214–510) | 200 (100–360) | 0.001 | |
(mmHg) | 34 (30–38.6) | 36.4 (31.8–41.6) | 0.034 |
23 (21–25) | 24.3 (21.2–27) | 0.134 | |
Central temperature (°C) | 35.8 (34–36.5) | 35 (31–36.7) | 0.541 |
Arterial blood lactates (mmol/l) | 1.4 (1.0–2.1) | 2.3 (1.3–4.7) | 0.001 |
Regression coefficient | OR (95% CI) | P -value | |
Independent predictors of mortality | |||
−0.004 | 0.996 (0.993–0.998) | 0.002 | |
Blood lactates (mmol/l) | 0.323 | 1.382 (1.178–1.621) | 0.001 |
Constant | −3.210 |
Variable . | Survivors ( N = 1360) . | Non-survivors ( N = 32) . | P -value . |
---|---|---|---|
pH | 7.4 (7.4–7.5) | 7.4 (7.3–7.47) | 0.081 |
0.51 (0.48–0.59) | 0.56 (0.48–0.67) | 0.062 | |
(mmHg) | 204 (112–258) | 103 (62–192) | 0.001 |
399 (214–510) | 200 (100–360) | 0.001 | |
(mmHg) | 34 (30–38.6) | 36.4 (31.8–41.6) | 0.034 |
23 (21–25) | 24.3 (21.2–27) | 0.134 | |
Central temperature (°C) | 35.8 (34–36.5) | 35 (31–36.7) | 0.541 |
Arterial blood lactates (mmol/l) | 1.4 (1.0–2.1) | 2.3 (1.3–4.7) | 0.001 |
Regression coefficient | OR (95% CI) | P -value | |
Independent predictors of mortality | |||
−0.004 | 0.996 (0.993–0.998) | 0.002 | |
Blood lactates (mmol/l) | 0.323 | 1.382 (1.178–1.621) | 0.001 |
Constant | −3.210 |
CI: confidence interval; OR: odds ratio.
Variable . | Survivors ( N = 1360) . | Non-survivors ( N = 32) . | P -value . |
---|---|---|---|
pH | 7.4 (7.4–7.5) | 7.4 (7.3–7.47) | 0.081 |
0.51 (0.48–0.59) | 0.56 (0.48–0.67) | 0.062 | |
(mmHg) | 204 (112–258) | 103 (62–192) | 0.001 |
399 (214–510) | 200 (100–360) | 0.001 | |
(mmHg) | 34 (30–38.6) | 36.4 (31.8–41.6) | 0.034 |
23 (21–25) | 24.3 (21.2–27) | 0.134 | |
Central temperature (°C) | 35.8 (34–36.5) | 35 (31–36.7) | 0.541 |
Arterial blood lactates (mmol/l) | 1.4 (1.0–2.1) | 2.3 (1.3–4.7) | 0.001 |
Regression coefficient | OR (95% CI) | P -value | |
Independent predictors of mortality | |||
−0.004 | 0.996 (0.993–0.998) | 0.002 | |
Blood lactates (mmol/l) | 0.323 | 1.382 (1.178–1.621) | 0.001 |
Constant | −3.210 |
Variable . | Survivors ( N = 1360) . | Non-survivors ( N = 32) . | P -value . |
---|---|---|---|
pH | 7.4 (7.4–7.5) | 7.4 (7.3–7.47) | 0.081 |
0.51 (0.48–0.59) | 0.56 (0.48–0.67) | 0.062 | |
(mmHg) | 204 (112–258) | 103 (62–192) | 0.001 |
399 (214–510) | 200 (100–360) | 0.001 | |
(mmHg) | 34 (30–38.6) | 36.4 (31.8–41.6) | 0.034 |
23 (21–25) | 24.3 (21.2–27) | 0.134 | |
Central temperature (°C) | 35.8 (34–36.5) | 35 (31–36.7) | 0.541 |
Arterial blood lactates (mmol/l) | 1.4 (1.0–2.1) | 2.3 (1.3–4.7) | 0.001 |
Regression coefficient | OR (95% CI) | P -value | |
Independent predictors of mortality | |||
−0.004 | 0.996 (0.993–0.998) | 0.002 | |
Blood lactates (mmol/l) | 0.323 | 1.382 (1.178–1.621) | 0.001 |
Constant | −3.210 |
CI: confidence interval; OR: odds ratio.
The arterial blood lactates yielded a moderately good discriminative power (AUC = 0.740), with a cut-off value at 2.8 mmol/l (sensitivity 66.5%, specificity 77.7%, positive predictive value 8.3%, negative predictive value 98.6% and odds ratio for mortality 5.7). Additional cut-off values were identified at a level of 5 mmol/l (odds ratio for mortality 10.6) and 8 mmol/l (odds ratio for mortality 14.0).
The ABC Score was included in a multivariable model with additional early postoperative parameters ( and arterial blood lactates as continuous variables), and based on the odds ratios, a corresponded to 1.5 additional points, and each increase of 1 mmol/l of arterial blood lactates corresponded to 1 additional point (Table 4 ). The resulting modified ABC Score (modABC Score) was therefore structured as the ABC Score plus the arterial blood lactate values plus 1.5 points if the was <200.
Variable . | Regression coefficient . | OR (95% CI) . | P -value . |
---|---|---|---|
ABC Score | 0.305 | 1.357 (1.104–1.668) | 0.001 |
0.768 | 2.155 (1.014–4.580) | 0.046 | |
Blood lactates (mmol/l) | 0.383 | 1.467 (1.248–1.724) | 0.001 |
Constant | −7.540 |
Variable . | Regression coefficient . | OR (95% CI) . | P -value . |
---|---|---|---|
ABC Score | 0.305 | 1.357 (1.104–1.668) | 0.001 |
0.768 | 2.155 (1.014–4.580) | 0.046 | |
Blood lactates (mmol/l) | 0.383 | 1.467 (1.248–1.724) | 0.001 |
Constant | −7.540 |
ABC: Aristotle Basic Complexity; CI: confidence interval; OR: odds ratio.
Variable . | Regression coefficient . | OR (95% CI) . | P -value . |
---|---|---|---|
ABC Score | 0.305 | 1.357 (1.104–1.668) | 0.001 |
0.768 | 2.155 (1.014–4.580) | 0.046 | |
Blood lactates (mmol/l) | 0.383 | 1.467 (1.248–1.724) | 0.001 |
Constant | −7.540 |
Variable . | Regression coefficient . | OR (95% CI) . | P -value . |
---|---|---|---|
ABC Score | 0.305 | 1.357 (1.104–1.668) | 0.001 |
0.768 | 2.155 (1.014–4.580) | 0.046 | |
Blood lactates (mmol/l) | 0.383 | 1.467 (1.248–1.724) | 0.001 |
Constant | −7.540 |
ABC: Aristotle Basic Complexity; CI: confidence interval; OR: odds ratio.
The relationship between the modABC Score and the mortality rate is shown in Fig. 2 . The model was tested with a Hosmer–Lemeshow test, showing a good accuracy ( χ2 7.22, P = 0.511). Discrimination was good, with an AUC of 0.803 (95% CI 0.781–0.824). The discriminative properties of the ABC Score and the modABC Score were compared (Fig. 3 ), demonstrating that the discrimination power was significantly ( P = 0.043) higher for the modABC Score.
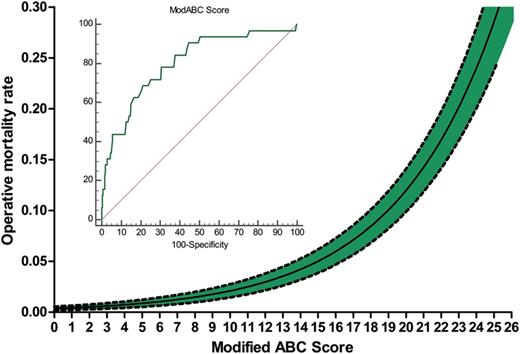
Association between modified Aristotle Basic Complexity (modABC) Score and mortality risk, with receiver operating characteristic curve (data in the text). Dashed lines are the 95% confidence interval.
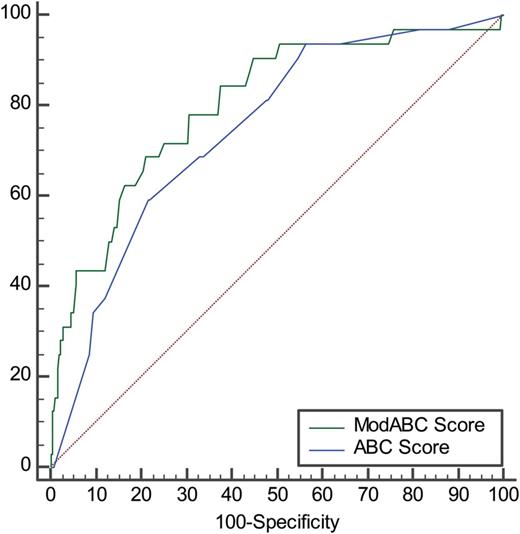
Receiver operating characteristic curve comparison between the Aristotle Basic Complexity (ABC) Score and the modified Aristotle Basic Complexity (modABC) Score. Data in the text.
A reclassification analysis (Fig. 4 ) was structured, analysing the number and rates of the patients having their risk category reclassified by the modABC with respect to the ABC Score. Six hundred and twenty-four (44.8%) patients did not change their risk class; 564 (40.5%) patients decreased their risk class by one level and 103 (7.4%) by two levels; 68 (4.9%) patients increased their risk class by one level, 31 (2.2%) by two levels and 2 (0.2%) by three levels.
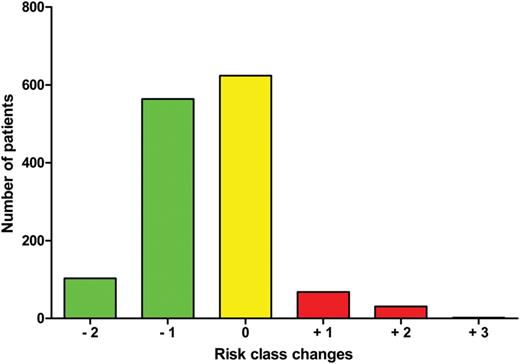
Risk class changes from the Aristotle Basic Complexity Score and the modified Aristotle Basic Complexity Score.
Within the patients who were reclassified into lower risk classes ( N = 667), the observed mortality rate was 0.9%. The ABC Score-predicted mortality risk was 1.8% (95% CI 1.6–1.9%), significantly ( P < 0.05) higher than the observed, whereas the modABC Score produced a more appropriate estimate of the mortality risk at 1.1% (95% CI 1.0–1.2%). Within the patients who were reclassified into higher-risk classes ( N = 101), the observed mortality rate was 4.0%. The ABC Score-predicted mortality rate was 2.0% (95% CI 1.8–2.2%), significantly ( P < 0.05) underestimating the mortality risk, whereas the modABC Score produced a predicted mortality risk of 4.5% (95% CI 3.7–5.3%) closer to the observed value.
DISCUSSION
Main findings
Our study includes a large patient population of paediatric (0–12 years) patients undergoing corrective cardiac surgery with CPB. Within this setting, we could demonstrate that (i) the preoperative assessment of mortality risk with the ABC Score provides an acceptable accuracy and discrimination; (ii) early postoperative hypoxaemia is an independent risk factor for mortality; (iii) early postoperative arterial blood lactate values are independent predictors of mortality and (iv) a modified ABC Score, inclusive of these two variables, significantly increases the accuracy and discrimination for operative mortality risk prediction, allowing a reclassification into different risk categories in more than 50% of the patient population.
Post-cardiopulmonary bypass hypoxia
Many studies addressed the determinants and clinical consequences of post-CPB hypoxia in adult cardiac surgery patients [ 10–13 ]. Within this setting, recognized mechanisms are atelectasis, ventilation/perfusion mismatch, fluid overload, increased alveolo-capillary permeability, alveolar endothelium injury due to the release of inflammatory mediators and impaired nitric oxide release. Additional factors are related to left ventricular dysfunction with increased left atrial pressures, and the effects of massive transfusions of allogeneic blood products.
A few studies investigated post-CPB hypoxia in paediatric patients, mainly focusing on fluid overload [ 14 ]. In paediatric patients, and mainly in newborns, the same mechanisms leading to post-CPB hypoxia in adults may be identified; additionally, fluid overload is usually more relevant, and the impact of transfusions (blood prime and/or additional allogeneic blood products used during surgery) is more relevant in terms of the ratio of the transfused volume to the circulating blood volume. Additionally, other factors, specific for the congenital heart disease scenario, may play a role as determinants of postoperative hypoxia. Among this, hypoxia may be related to a poor pulmonary blood flow due to (i) pulmonary hypertension (i.e. after truncus arteriosus or complete atrioventricular canal repair), (ii) right ventricular systolic or diastolic dysfunction (i.e. after tetralogy of Fallot repair) and (iii) malfunction of a direct total cavopulmonary connection (i.e. after Fontan operation).
Despite this, the lack of specific studies investigating post-CPB hypoxia in paediatric patients may be justified by the difficult experimental setting, where residual postoperative right-to-left shunts induce a lung function-independent hypoxia.
In our patient population, we have excluded the patients with a residual right-to-left shunt, avoiding this confounder. A poor ratio (<200) was identified as an independent risk factor for mortality, corresponding to an additional 1.5 points of ABC Score. However, the impact of this factor as a determinant of operative mortality appears limited, and no additional mortality rate increase was found when testing more severe patterns of hypoxia.
As a matter of fact, there are a number of possible interventions that the clinicians in charge for postoperative care may apply in order to correct early postoperative hypoxia (change in ventilator pattern, use of diuretics, use of inotropic drugs to support the right ventricular function and use of inhaled nitric oxide). It is possible to hypothesize that for this reason, the role of early postoperative hypoxaemia as a determinant of mortality, albeit significant, appears limited in clinical terms.
Increased arterial blood lactate levels
Contrary to postoperative hypoxaemia, the postoperative increase of arterial blood lactates has been extensively investigated in paediatric patients undergoing heart surgery.
Recently, Schumacher et al. [ 15 ] investigated the time course of postoperative arterial blood lactates in 231 infants after heart surgery, demonstrating that an increase in the rate of 0.6 mmol/l/h had an excellent discriminatory power for bad outcomes, and the same group identified peak serum lactate as a prognostic factor for the need of extracorporeal membrane oxygenation support after the Norwood procedure [ 16 ].
Previously, Maarslet et al. [ 17 ] studied 206 children undergoing heart surgery and found that the arterial blood lactate value at arrival in the ICU was predictive for mortality and need for renal replacement therapy. An arterial blood lactate value of ≥4.5 mmol/l yielded an odds ratio for mortality of 8.4. This value is very similar to our findings, where an arterial blood lactate value of >5 mmol/l had an odds ratio for mortality of 10.6.
In our series, arterial blood lactates at arrival in the ICU are strongly associated with operative mortality. Differently from postoperative hypoxia, no clear cut-off value could be identified, and the relative mortality risk appears to linearly increase with increasing arterial blood lactate levels above 2 mmol/l. Arterial blood lactates at arrival in the ICU actually reflect an intraoperative production, generally attributable to an inadequate oxygen delivery with respect to the oxygen needs during and/or after CPB (defined as type A hyperlactataemia, expression of end-organ(s) ischaemia). Other intraoperative factors leading to hyperlactataemia are a reduction of lactate catabolism due to low hepatic flow and increased lactate production by β-adrenergic stimulation [ 18 ]. Other studies have linked hyperlactataemia intraoperatively [ 19 ] or during CPB [ 20 ] to mortality and bad outcomes in paediatric heart surgery.
Implementation of mortality risk prediction using early postoperative parameters
In adult cardiac surgery, mortality risk prediction is generally based on preoperative risk scores, like the STS Score, The European System for Cardiac Operative Risk Evaluation (EuroSCORE) and the Age, Creatinine and Ejection Fraction (ACEF) Score [ 21 , 22 ], even if attempts to include early postoperative parameters have been published [ 23 , 24 ].
In paediatric heart surgery, the standard of risk stratification is represented by the ABC Score, the ACC Score, the RACHS-1 Score and the STAT Mortality Score and Mortality Categories, all based on preoperative parameters. Even if all these scores have been tested and validated in many series [ 1 , 5–8 ], the attempt to improve their accuracy and discriminative power appears reasonable. As a matter of fact, the complexity of cardiac surgery in the congenital heart scenario involves many intraoperative factors which may considerably change a risk prediction based on preoperative data. Among these, important roles are played by changes from the planned surgery to different approaches (based on unexpected findings, anatomical and technical problems), by the impact of ischaemia–reperfusion injury in cyanotic hearts, by the deleterious effects of CPB that are more evident with greater discrepancy between oxygenator and circuit size and patient size and others. All these factors are difficult to be included into a risk stratification based on preoperative factors and the type of surgery only, and the fact that the paediatric patient may reach the ICU with a mortality risk very different from the preoperative one.
Our modified ABC Score incorporates two simple early postoperative factors that reflect a number of possible intraoperative mechanisms related to lung function and haemodynamics. In our series, the inclusion of these factors significantly improved the accuracy and discriminative power of the ABC Score. A similar approach was followed by Molina Hazan et al. [ 25 ], who incorporated early postoperative arterial blood lactates into the RACHS-1 Score in a series of 255 children receiving heart surgery. Even if the preoperative scoring system was different from the ABC Score, the data presented by these authors allow some comparative analyses.
The authors used the arterial blood lactate value at arrival in the ICU to correct the RACHS-1 Score mortality prediction. As in our study, they did not set on a cut-off value, and the arterial blood lactate value was used as a continuous variable. They found that the relative mortality risk increased by 3.8% per each increase of 1 mg/dl of arterial blood lactate (corresponding to 0.11 mmol/l). Our data support this finding, with a relative risk for mortality increasing by 5.6% for every 1 mg/dl increase of arterial blood lactate.
The main strength of our study is the large patient population included, greatly exceeding the series published until now. There are, of course, limitations in our study. The improvement in discriminative power obtained by the modABC Score with respect to the ABC Score is modest, albeit significant; however, it allowed a reclassification into different risk categories in more than 50% of our patient population. The study is limited to corrective surgery without a residual right-to-left shunt, and includes a heterogeneous patient population ranging from newborns to 12-year-old children. Finally, it is based on a retrospective single-centre experience, without internal and external validation. Given the low mortality rate actually registered for this kind of surgery, a large, multicentre, prospective validation study comparing our modified ABC Score with the other testing scores (ABC, RACHS-1 and STAT) is certainly needed.
Funding
This study was funded by local research funds of the IRCCS Policlinico San Donato.
Conflict of interest: none declared.
REFERENCES