-
PDF
- Split View
-
Views
-
Cite
Cite
Mayura Meerang, Andreas Boss, David Kenkel, Angela Broggini-Tenzer, Karima Bérard, Olivia Lauk, Stephan Arni, Walter Weder, Isabelle Opitz, Evaluation of imaging techniques for the assessment of tumour progression in an orthotopic rat model of malignant pleural mesothelioma, European Journal of Cardio-Thoracic Surgery, Volume 47, Issue 1, January 2015, Pages e34–e41, https://doi.org/10.1093/ejcts/ezu393
- Share Icon Share
Abstract
An orthotopic rat tumour recurrence model for malignant pleural mesothelioma (MPM) provides clinical similarity to patients and is useful for drug testing combined with surgical intervention. Importantly, a reliable imaging method is required allowing for noninvasive and repetitive evaluation of the tumour load. We compared the tumour load assessed by bioluminescence and magnetic resonance imaging (MRI) to the macroscopic tumour volume as a reference standard.
A total of 500 000 syngeneic rat MPM cells transfected with luciferase were implanted underneath the parietal pleura of immunocompetent rats (n = 13). From the second day after implantation, bioluminescence measurements of the tumour load expressed as the maximum bioluminescent intensity (photon/second) were performed daily after intraperitoneal injection of the luciferase substrate, d-luciferin, to observe the first occurrence of tumour. Six days after the first detection of tumour, bioluminescence, MRI and macroscopic tumour volume measurement were conducted. For MRI, a 4.7-Tesla small animal imager equipped with a 1H whole-body rat coil was employed using T2-weighted fast spin-echo sequences. Tumour burden (mm3) was quantified from magnetic resonance transverse images by two independent readers by manual segmentation. Finally, the tumour burden assessed by bioluminescence and MRI was correlated (Pearson's correlation) with the macroscopic measurement of tumour (ellipsoid) volume.
In all rats, a single tumour nodule was found at the inoculation site with a median macroscopic volume of 46 mm3 (18–377 mm3). For tumour burden quantification of MRIs, we observed good interobserver correlation (R2 = 0.81, P < 0.0001) as well as significant association with the macroscopic tumour volume (R2 = 0.59, P = 0.002). However, the signal intensity of bioluminescence did not correspond to the macroscopic tumour volume (R2 = 0.01, P = 0.76).
MRI is a reliable and reproducible noninvasive in vivo imaging method for MPM tumour burden assessment for the present MPM model.
INTRODUCTION
Malignant pleural mesothelioma (MPM) is a rare and aggressive tumour. The major cause of MPM is attributed to the inhalation of asbestos fibres into the lung causing chronic inflammation and carcinogenesis. Although the use of asbestos in Europe was banned in the 1980s, the incidence is expected to rise in the next 5–10 years because of long disease latency [1]. Currently, the most widely used treatment approach includes a combination of chemotherapy (cisplatin plus pemetrexed [2]) plus macroscopic complete resection [3] with optional adjuvant radiotherapy [4]. Nonetheless, treatment response and long-term outcome are still very variable (reviewed in [5]). Therefore, it is important to explore novel targets as an alternative treatment option for MPM patients not responding to current protocols.
Besides chemotherapy directed against proliferative tumour cells, molecular targeted treatment and also immune therapy have drawn major interest [6, 7]. Prior to clinical trials, efficiency of new drug candidates have been tested in vitro using MPM primary cells or MPM human cell lines followed by preclinical animal models. However, in vitro conditions cannot fully mimic the tumour microenvironment that includes stromal cells, cytokines, chemokine, blood and oxygen gradient which all together play a major role in the growth, development and therapeutic resistance of MPM. Thus, a reliable in vivo model of MPM that closely resembles the clinical situation is required for testing the efficacy of new drug candidates.
Many studies have relied on the use of a subcutaneous or orthotopic xenograft immunodeficient tumour model to test the efficacy of a new compound as well as its pharmacokinetics and toxicities in vivo [8–11]. However, the immune system plays an important role in tumour biology and may influence the regression of an MPM [12, 13]. Thus, an immunodeficient animal model cannot entirely represent the clinical situation.
Our group has established an orthotopic immunocompetent rat model by implanting syngeneic rat mesothelioma cells (IL45) underneath the parietal pleura, which regrows after surgical resection [14, 15]. This model was employed to test the efficacy of a multimodal treatment model combining surgery with intrapleural cisplatin treatment or immunochemotherapy [15, 16]. However, an in vivo, noninvasive imaging method is needed for reliable detection and quantification of the tumour burden. Fluorescence, bioluminescence (Bli), computed tomography (CT) and magnetic resonance (MR) have been employed for orthotopic MPM tumour imaging [10, 11]. We created Bli-expressing clones of an IL45 cell line (IL45-Luc) by stable transfection [17]. The implantation of the IL45-Luc cells in an immune-competent rat generated tumour masses that could be detected by the Bli imager as soon as 3 days after inoculation. The IL45-Luc-derived tumour displayed the same histology as tumours derived from un-transfected IL45 cells [17].
Discordance between Bli and the macroscopic tumour volume measured was observed in some cases; therefore, we implemented an MR imaging protocol in a small animal imager as a third independent technique to assess the tumour burden. Here, we present an approach to quantify tumour burden from MR images. We investigated the correlation between the tumour burden quantified from MR images and Bli signal with the tumour volume measured macroscopically as a reference standard.
MATERIALS AND METHODS
Tumour inoculation
The IL45-Luc cell line was maintained as previously described [17]. Immunocompetent male Fischer 344 rats (n = 13) (F344/NCrHsd; 200–250 g) were purchased from Harlan Laboratories (the Netherland) and housed according to standard guidelines of the Swiss animal health-care law. Experiments were approved by the veterinary office of Zürich. We inoculated 500 000 IL45-Luc cells suspended in 50 µl of Dulbecco's phosphate-buffered saline underneath parietal pleura as previously described [17]. For analgesia, all rats received paracetamol (30 mg/kg) 30 min before and 4 h after surgery.
Bli measurement began two days after inoculation, and continued daily until Bli signal appeared (n = 13). The first Bli signals were detected between 2 and 6 days after inoculation. After the first detection, tumours were grown for 6 days in order to avoid >15% body weight loss. Seven rats received daily treatment of a hedgehog antagonist which inhibits tumour cell proliferation by 10%, generating variation in tumour volume. Bli and MR imaging were conducted prior to euthanization and the macroscopic tumour volume measurement.
Bioluminescence imaging and the analysis
Animal preparation for Bli imaging was performed as previously described [17]. Bli images were sequentially acquired with exposure time of 60 s until the highest Bli signal was detected. We used IVIS 200 living image software (Caliper Life Sciences, Inc., MA, USA) to construct pseudo colour images of Bli signal overlaid on the animal's whole-body picture. A region of Bli [region of interest (ROI)] was selected and quantified for area (mm2) and total Bli signal (photon/second). Total Bli emission was plotted against time after d-luciferin injection. The peak of Bli signal was taken for analysis.
Magnetic resonance imaging and the quantification of tumour volume
Magnetic resonance imaging (MRI) was performed with a Bruker 4.7 T Pharmascan 47/16 US with a gradient strength of 375 mT/m and a slew rate of 3375 T/ms. A linear polarized whole-body mouse 1H radiofrequency coil was applied for spin excitation and signal reception. During the scan, the rats were anaesthetized with isoflurane (Attane; Minrad I, Buffalo, NY, USA). On-line respiration monitoring was performed with an MR-compatible physiology unit (SA Instruments, Inc., NY, USA). After gradient-echo localizers in three spatial directions, the imaging protocol consisted of a 2D encoded T2-weighted fast spin-echo sequence (TR/TE, 3528 ms/11 ms; effective TE, 45 ms; echo train length, 8; matrix, 384 × 384; field of view, 60 × 60 mm2; slice thickness, 1 mm; number of acquisitions, 5; interslice gap, 0.5 mm) in transverse, sagittal and coronal orientation. After data acquisition, data sets were transferred to an off-line computer.
the complete tumour volume being obtained by integrating the volumes of all slice intervals (see Fig. 1).
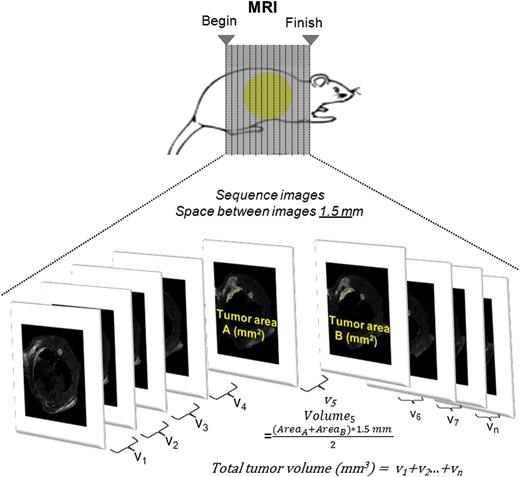
Macroscopic tumour volume quantification
After euthanasia with an overdose of pentobarbital and exsanguination, we excised the chest wall that contained the tumour nodule. The length (a) and width (b) of the tumour were measured with a slide callipers. The tumour area (mm2) was calculated by the ellipsoid formula: area = π × a/2 × b/2. The thoracic wall was cut at the thickest part and fixed in formalin for histological analysis. The thickness of tumour (c) was measured on the H&E section. Finally, the tumour volume was approximated using the ellipsoid formula: V = 4/3 π × a/2 × b/2 × c/2 [15].
Histology and proliferation index
Tissues were fixed in 4% formalin for 24 h and then de-calcified in EDTA solution for 2–3 weeks. Afterwards, they were intensively washed in water, dehydrated in a series of alcohol solutions and embedded in paraffin. Tissues were cut at 3 μm thickness and stained for H&E and collagen using the Sirius red method. The proliferation index with Ki67 (rabbit anti-Ki-67, CMC27531021; Cell Marque) was quantified as the percentage of positive Ki67 tumour nuclei per total tumour nuclei [18].
Statistical analysis
Statistical analysis has been performed by computation of Pearson's correlation coefficient using Prism 5 (GraphPad software, Inc., CA, USA). A P value of less than 0.05 was considered significant. The intraclass correlation coefficient was computed by SPSS 22.
RESULTS
Characteristics of IL45-Luc-derived tumour
At autopsy (6 days after the first tumour detection), a single tumour nodule was visible at the injection site (Fig. 2A) in all rats with a median macroscopic tumour volume of 46 mm3 (range; 18–377 mm3, Supplementary Table 1). As described previously, tumours derived from IL45-luc were of the sarcomatoid mesothelioma subtype (Fig. 2B). The stromal compartment including fibroblasts, immune cells, extracellular matrix and vessels was present (Fig. 2B and C). Tumours were highly proliferative as shown by the over 70% Ki-67 staining index (Fig. 2D), and the tumours were without any necrotic area.
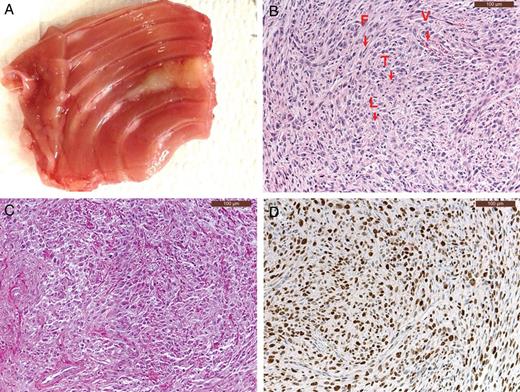
(A) Macroscopic appearance of a tumour derived from IL45-Luc cells. A white tumour nodule is found around the injection site. (B) In an H&E staining and indicated by arrow; tumour cells (T), stromal part containing blood vessels (V), lymphocytes (L) and fibroblasts (F). (C) Collagen staining showing the presence of collagen bundles (red) infiltrates. (D) Ki67 staining (brown) of tumour cells.
Bioluminescence imaging
The median maximum Bli signal was observed at 21.5 min (range; 16–32 min) after injection of d-luciferin with the median bioluminescent value of 1.21 × 107 p/s (range; 3.6 × 106–4.9 × 107 p/s, Supplementary Table 1). We observed postoperative inflammation localized at the thoracotomy site in some rats and reduced bioluminescence signal within this area (data not shown). Tumour areas (2D) quantified from Bli were 30 times (range; 12–45 times) larger than the actual macroscopic tumour area but showed a trend of positive correlation (R2 = 0.22, P = 0.1) (Fig. 3A). However, the total tumour burden quantified from the Bli image (photon/second) did not correlate with macroscopic tumour volume (Fig. 6B).
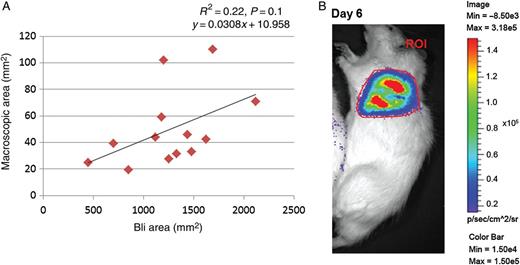
(A) A trend of positive correlation between tumour area quantified in Bli images and macroscopically (n = 13). (B) Example of Bli image of a rat (ROI: region of interest).
Magnetic resonance images
Tumour nodules appeared within 5–14 image sections, showing irregular shape. Tumour and postoperative scar were visible as slightly hyperintense signals compared with the chest wall, whereas strong hyperintensity represents areas of inflammation or fluid collections (Fig. 4A). The correlation of tumour volume measured from MR images by the two observers was highly significant with a Pearson correlation coefficient of 0.81 (Fig. 4B) and intraclass correlation coefficient of 0.903. For the further analyses, the average of tumour volumes quantified by the two observers was used. The median tumour burden quantified from MR images was 145.4 mm3 (range; 77.7–609.8 mm3, Supplementary Table 1) and was 3.5 (median) times higher than the one measured macroscopically (range; 0.7–7.6 times). We could not observe any correlation between tumour burden quantified from MR images and total Bli signal (Fig. 5A and B). However, tumour volume measured macroscopically and from MRI images correlated significantly (Fig. 6A).
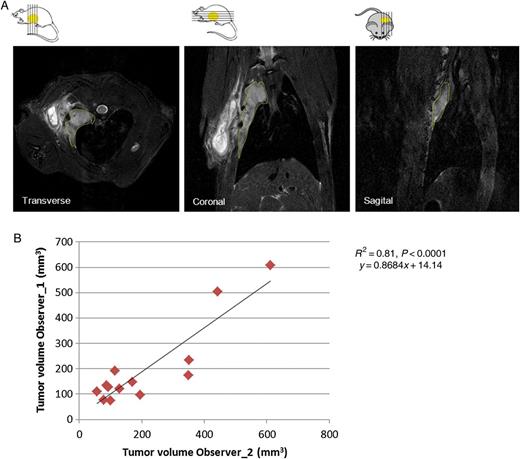
(A) Example of MR images (transverse/coronal/sagittal); the margins of tumour area are indicated with yellow lines. (B) Significant correlation of tumour volumes quantified in transverse images by two independent observers (n = 13).
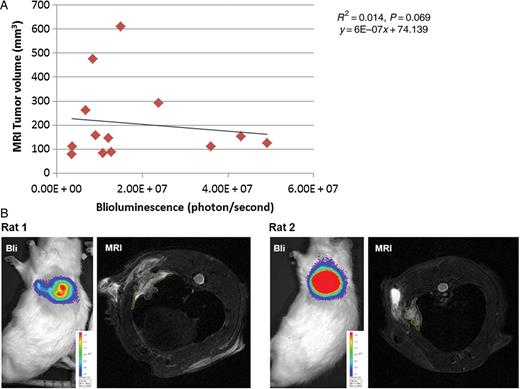
(A) Scatter plot between tumour volume quantified from MR images and Bli signal showing no correlation (n = 13). (B) Images showing typical discrepancies between tumour volume visualized by MR and Bli signal. Rat 2 shows strong Bli signal (red); however, the tumour size visible by MRI is smaller than that of Rat 1, which exhibited lower Bli intensity.
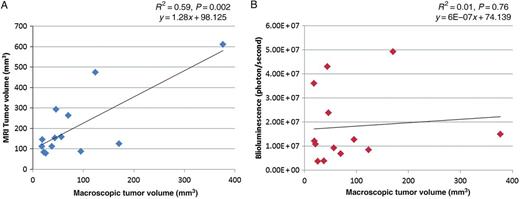
Correlation between macroscopic tumour volume versus MRI and Bli: (A) Positive correlation between tumour volume quantified from MR images (mm3) (average of two observers) and macroscopic tumour volume. (B) Bli signal (photon/second) does not correlate with macroscopic tumour volume.
Discussion
Our orthotopic immune-competent rat MPM model provides a tumour microenvironment that closely reflects the clinical situation. It was proved useful for testing new drug candidates as well as surgical intervention and will provide greater benefit if the tumour can be monitored repetitively and noninvasively. MR imaging and Bli were assessed for their reliability in tumour burden quantification by comparing with the macroscopic tumour volume. In this study, we found that MR imaging provides more reliable tumour burden assessment than Bli as it correlates strongly with the macroscopic tumour volume. Quantification of tumour burden from MR images is a reliable approach as the measurements can be reproduced independently by two observers. MR imaging has been successfully applied for the visualization of MPM in the orthotopic mouse model of MPM as well as other models of solid tumours [10, 19].
The quantification of tumour volume from MR images was feasible and reproducible (good interobserver agreement). Nevertheless, we observed that fibrous postoperative scar interferes with the quantification of tumour volume from MR images. A possible solution for the discrimination between tumour and the postoperative changes including inflammation and fibrosis might be the use of ‘diffusion-weighted imaging’ (DWI). ‘Apparent diffusion coefficient’ values, which are commonly used to quantify the results of DWI, are described as the specific parameter for the differentiation of recurrence of tumour and scar in breast cancer [20]. However, the role of DWI in the differentiation of mesothelioma from postoperative changes is hitherto unknown. Furthermore, an impeded diffusion that is seen in tumours can also be seen in inflammatory or infected areas due to the increased cellular density in those areas [21]. Therefore, DWI might be an interesting tool for the differentiation of tumours and postoperative changes in the future, but the potential application for mesothelioma needs to be evaluated. Furthermore, a semi-automated approach has been successfully applied for the measurement of MPM tumour thickness in CT scan images [22]. A computer interface requires users to indicate an initial end-point; thereafter, it determines the corresponding terminal end-point automatically using a greyscale threshold. It showed high agreement with manual quantification and thus may also be applicable for tumour volume quantification from MR images that we acquired.
The tumour volume obtained from MR images was about two times bigger than the volume quantified macroscopically. This systemic positive bias could be explained by continuous underestimation of tumour thickness measured from H&E sections due to shrinkage of tissues after a fixation and dehydration procedure. Indeed the thickness of the tumour measured from MR images was larger than the thickness measured from H&E sections (data not shown).
Several studies in subcutaneous or intraperitoneal tumour models showed strong correlation between Bli and actual tumour volume [23, 24]. In our study, 2D analyses (tumour area) of both Bli and macroscopic observation show only a trend of positive correlation; this may be explained by the small sample size. However, tumour areas visualized in Bli images were overestimated probably due to the inclusion of light radiance. Total bioluminescent emission (3D) correlates with neither the macroscopic tumour volume nor MRI tumour burden. This discordance between Bli and tumour volume can be explained by several reasons. The emission of Bli signal depends on the extent of luciferase expression and the penetration depth of light [25]. In our model, tumour nodules were deeply embedded in the thoracic cavity. In some cases, Bli can be hindered near the operation site by tissue thickening that results from inflammation and postoperative scar. Accurate injection of d-luciferin into the intraperitoneal cavity is crucial as, in some cases, no Bli signal was detected after injection. Upon re-injection of d-luciferin into the same rat, Bli signal appeared immediately. Intravenous administration may be a better method for applying the correct dosage of d-luciferin; however, it is practically difficult to inject sequentially large volumes of substrate intravenously in rats (authors’ own experience). There are different types of information obtained from Bli signal versus total tumour volume. Bli signal can only be emitted from living tumour cells and therefore indicates the burden of tumour cells alone, excluding apoptotic and necrotic cells. It is a sensitive detection method and is suitable for early detection of tumour growth after testing a compound that causes necrosis and apoptosis. However, Bli signal does not include the stromal fraction. Total tumour volume can be represented by MR images and macroscopic observations. Thus, the combination of the two methods may provide an advantage and distinguish the effect of drugs on the stromal compartment.
Disadvantages of the MR examination are that they are relatively costly examinations due to the purchasing costs and the maintenance of the MR imager. The duration of MR image acquisition varied from 45 min to 1 h per rat, hence increasing anaesthesia and experimental duration. In principle, metals could be a problem, e.g. implanted material after surgery, due to the propensity of these materials to cause distortions of the magnetic field. However, the material used in this study did not cause relevant image artefacts. On the other hand, Bli provides a faster imaging method as two rats can be placed in the Bli imaging chamber and measured simultaneously for a maximum of 35 min. However, a big volume of d-luciferin is required for a rat weighting between 250 and 300 g and the injection caused stress especially in repetitive measurements.
In our specific orthotopic rat MPM model, MR imaging provided more reliable tumour burden quantification. However, the measurements in rats were hindered by the postoperative scar and inflammation, which are common problems that impede tumour mass detection in humans. This model will be further defined to better discriminate between tumour and postoperative scar. In our study, a small thoracotomy was performed for tumour inoculation and we observed that the scar tissue and inflammation was diminishing over the recovery time of 6 days. By inoculating fewer tumour cells, the duration of the experiment may be extended to allow healing and recovery from inflammation before the assessment. These imaging techniques may be applied for tumour load assessment following major surgery such as radical pleurectomy and extrapleural pneumonectomy; however, proper recovery time needs to be validated. Moreover, contrast medium for MR imaging will be tested for its potential application to discriminate tumour from scar. Advances in the development of imaging methods for such orthotopic preclinical animal models will represent great progress in the field of cancer research for therapeutic development.
SUPPLEMENTARY MATERIAL
Supplementary material is available at EJCTS online.
Funding
This work was supported by a Swiss National Science Foundation professorship to Isabelle Opitz.
Conflict of interest: none declared.
ACKNOWLEDGEMENTS
We thank Martin Pruschy for providing the Bli imager and support with the preliminary assessment. In addition, we thank Rolf Stahel and Emanuela Felley-Bosco for providing helpful and insightful comments for the project. P. Vogt is acknowledged for providing assistance for interpretation of tumour histology. We are also indebted to Chloé Spichiger for her editorial assistance in the preparation of the manuscript.
REFERENCES
Author notes
Presented at the 22nd European Conference on General Thoracic Surgery, Copenhagen, Denmark, 15–18 June 2014.