-
PDF
- Split View
-
Views
-
Cite
Cite
Annemieke Smorenberg, Erik J. Lust, Albertus Beishuizen, Jan H. Meijer, Ruud M. Verdaasdonk, A.B. Johan Groeneveld, Systolic time intervals vs invasive predictors of fluid responsiveness after coronary artery bypass surgery, European Journal of Cardio-Thoracic Surgery, Volume 44, Issue 5, November 2013, Pages 891–897, https://doi.org/10.1093/ejcts/ezt108
- Share Icon Share
Abstract
Haemodynamic parameters for predicting fluid responsiveness in intensive care patients are invasive, technically challenging or not universally applicable. We compared the initial systolic time interval (ISTI), a non-invasive measure of the time interval between the electrical and mechanical activities of the heart measured by impedance cardiography, with invasively measured haemodynamic parameters in predicting fluid responsiveness after cardiac surgery.
Thirty-two clinically hypovolemic patients admitted to the intensive care unit after coronary artery bypass surgery received 500 ml of gelatine solution in two volume loading steps of 250 ml at an infusion rate of 1000 ml/h. Haemodynamic and biochemical measurements were done at baseline and 15 min after each volume loading step with continuous recording of the impedance cardiogram and electrocardiogram.
Forty-four percentage (n = 14) of patients showed a stroke volume (SV) index increase >10%. ISTI predicted fluid responsiveness with an optimum threshold of >153 ms (P = 0.023) and a sensitivity of 71% and specificity of 78%. The predictive values of ISTI did not differ from those of arterial pressure or SV at baseline. A decrease of ISTI of ≥8.3 ms predicted fluid responsiveness with the highest positive predictive value (88%, P = 0.004) among the variables, and absence thereof virtually excluded fluid responsiveness (specificity 94%).
Non-invasively measured ISTI is able to predict and monitor fluid responsiveness after cardiac surgery non-inferiorly to invasively measured haemodynamic indices.
INTRODUCTION
Fluid loading is pivotal in the treatment of mechanically ventilated patients with acute circulatory failure after cardiac surgery, yet carries the risk of fluid overloading. After cardiac surgery, fluid overload is associated with an increase in mortality and morbidity such as infection, pulmonary oedema and arrhythmias [1]. Furthermore, only about half of the patients in an intensive care unit (ICU) show an increase in cardiac output (CO) corresponding to fluid infusion and are therefore fluid responsive, so that the prediction of fluid responsiveness could aid proper treatment of circulatory failure and prevent harmful overloading [2–4]. Dynamic parameters such as stroke volume (SV) and pulse pressure variation and haemodynamic responses to passive leg raising or respiratory holds have been shown to be superior in predicting fluid responsiveness to static indices such as central venous pressure (CVP), pulmonary artery occlusion pressure (PAOP) or transpulmonary thermodilution-derived volumes, in mechanically ventilated patients [2, 4–10]. However, both dynamic and static measurements are invasive, technically challenging or not universally applicable.
The pre-ejection period (PEP), the systolic time interval from the onset of ventricular depolarization to the beginning of left ventricular ejection, and its respiratory variation to alterations in intrathoracic pressures during mechanical ventilation, allow the assessment of ventricular function [11–13]. PEP and its respiratory variation have been shown to decrease during effective fluid loading and to predict haemodynamic changes induced by positive end-expiratory pressure and fluid infusion, yet require the presence of a radial artery catheter and are thus invasive [12, 14, 15]. The impedance cardiogram (ICG), obtained from bioimpedance measurements using thoracic electrodes, reflects the cardiac cycle [16]. The time difference between the Q-wave on the electrocardiogram (ECG) and the start of the systolic ejection phase on the ICG can be determined and is thought to reflect PEP [17]. Since the Q-wave can be hard to identify, the R-wave is used instead for the so-called initial systolic time interval (ISTI) [17, 18]. The use of these signals for clinical diagnostics is attractive: registrations can be obtained entirely non-invasively, rapidly, easily and are not restricted to a hospital environment.
We hypothesized that the non-invasively measured ISTI is able to predict and monitor fluid responsiveness in mechanically ventilated ICU patients after cardiac surgery, similarly to more-invasive haemodynamic indices.
Patients and methods
With the approval of the Medical Research Ethics Committee (MREC) of the VU University Medical Center, 35 clinically hypovolemic patients were included following elective coronary artery bypass surgery and admission to the ICU. Written informed consent was obtained prior to surgery. Patients were included when having a radial artery catheter and pulmonary artery (PA) catheter in place, the latter inserted via the jugular or subclavian vein as part of routine care, and when considered to be presumably hypovolaemic. The latter was defined by either systolic blood pressure <110 mmHg, CVP < 10 mmHg, PAOP < 12 mmHg, central or mixed venous O2 saturation (SvO2) <70%, or need of isotropic or vasopressor drugs (or combinations), as done previously [5]. Exclusion criteria were significant loss of blood by bleeding at a rate of ≥100 ml/h via thoracic drains, relevant alterations in inotropic or vasopressor drugs and dosage, presence of an operating artificial pacemaker, absence of sinus rhythm or clinical need to deviate from the research protocol. All patients were sedated and mechanically ventilated during the study, at stationary settings.
Protocol
Measurements were performed within 3 h after admission of the patient to the ICU. Four additional thoracic electrodes were attached for impedance cardiography registration by a single researcher to minimize measurement bias (Fig. 1) [17, 18]. Baseline measurements and blood sampling (baseline, T0) were done. Patients received 500 ml of the Gelofusin® (gelatine 40 g/l, B Braun Medical, Melsungen AG, Germany, in 154/120 mmol/l NaCl), in two-volume loading steps of 250 ml at a rate of 1000 ml/h. After the infusion of each loading step, 15 min were taken into account for haemodynamic stabilization, and measurements and blood sampling were repeated at 30-min intervals (T1 = T0 + 30 min and T2 = T1 + 30 min).
![The placement of the electrodes of the impedance cardiograph. A small electrical current (I) of 0.3 mArms is transmitted through the thorax, applied by the two outer electrodes, having a frequency of 64 kHz. The two inner electrodes passively sense an electrical voltage as a consequence of the small electrical current (V) [18].](https://oup.silverchair-cdn.com/oup/backfile/Content_public/Journal/ejcts/44/5/10.1093_ejcts_ezt108/1/m_ezt10801.gif?Expires=1750301399&Signature=pXeS0U6mqrC~BAsJ88krCylWCmKuKvaXPZEIOemnWBwi-Cm3qcoBWQam~lBRxZJ9bS5BIzZvHDiISS8FJZaKSs9z6kLwmR7JB8fSex2W3ecureZBTb73RXDNTN4yFIKWesP2t5vxABu0NmcykZPcv0HRrtflXK1I9yRymwb4BYrpnspMpeLci6pPfFNlmFunRHaLVQ1onfwo092z6PBhInu4wTXSlq1VbUejEPpMMuy3Z912dtFvpdotWXlQseV3k0RCiPUM2C7V0O2HN5o5yG7O5dDBlCidIGUN7zZPYNKDDigJZ1Hrb41fFboDeoKtalNcijBkfHJEbp6bZHYMgg__&Key-Pair-Id=APKAIE5G5CRDK6RD3PGA)
The placement of the electrodes of the impedance cardiograph. A small electrical current (I) of 0.3 mArms is transmitted through the thorax, applied by the two outer electrodes, having a frequency of 64 kHz. The two inner electrodes passively sense an electrical voltage as a consequence of the small electrical current (V) [18].
Measurements
CO and SV were measured using the thermodilution technique by injecting 10 ml of D5W solution at room temperature in triplicate and randomly in the respiratory cycle, and concomitant registration of the thermal shift in the PA, using a PA catheter connected to the bedside monitor (Marquette, WI, USA). Values were averaged and indexed to body surface area (e.g. SV index, SVI). Heart rate (HR), arterial pressures (systolic, SAP; mean, MAP), CVP, PAOP and ventilatory settings were registered. The CVP and PAOP (after proper wedging) were taken from the PA catheter. Pressures were obtained with patients in the supine position at the midchest level at end expiration, after calibration and zeroing to atmospheric pressure in mmHg (Marquette, WI, USA). At baseline and after each volume loading step, mixed venous blood was taken for determination of mixed venous O2 saturation (Sysmex SE-9000, Sysmex Corporation, Kobe, Japan). The arterial blood pressure curve from the radial artery catheter and ECG from the bedside monitor were recorded for PEP measurements. Impedance recordings were made in the supine position using an impedance cardiograph (Department of Physics and Medical Technology, VU University Medical Center, Amsterdam, Netherlands) and signals were AD-converted using an AD Instruments PowerLab 8SP and processed using ADInstruments Chart for Windows (version 5.1.1). The ECG and ICG were used for determination of ISTI. The ECG was recorded separately to eliminate any time-delay differences between the ICG and the ECG recorded by the bedside monitor. Recordings were made continuously throughout the study protocol from which the parameters were retrieved during off-line analysis.
Initial systolic time interval and pre-ejection period
ISTI was defined as the time interval between the R-point on the ECG and C-wave on the ICG (Fig. 2). The ISTI was measured as the mean value >30 s in milliseconds. These 30 s were divided into four periods of each 7.5 s. The respiratory variation in ISTI (%) was calculated as the difference between the maximal and minimal ISTI for each period, divided by mean ISTI in that period times 100. The dISTI was taken from the average variation over the four periods. PEP was defined as the time interval between the Q-point on the ECG of the bedside monitor and the beginning of the upstroke of the invasive radial arterial pressure curve (U-point). PEP and dPEP were determined with similar formulas used for ISTI and dISTI.
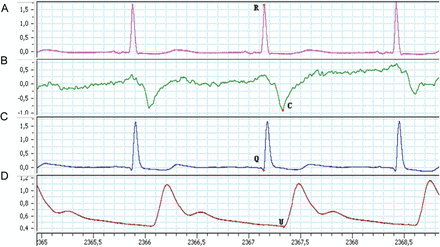
From top to bottom: (A) the ECG and (B) ICG from the impedance cardiograph and (C) ECG and (D) radial arterial pressure curve from the bedside monitor. ISTI was defined as the time interval in milliseconds between the R-point on the ECG and C-wave on the ICG, PEP as the time interval in milliseconds between the Q-point on the ECG and the beginning of the upstroke of the radial arterial pressure curve (U-point).
Statistical analysis
We did not perform a power analysis for this exploratory study. Fluid responsiveness was defined as a cardiac response of an increase in SVI at T = 2 of more than 10% compared with baseline (T = 2–T = 0). The Fisher's exact test for binary categorical variables and the χ2 test for remaining categorical parameters were used to compare groups using SPSS software, version 20. For continuous variables, the Mann–Whitney U-test was used. Spearman's correlation coefficient rs was applied to quantify relations between continuous variables. Receiver operating characteristic (ROC) analysis was used to assess the ability of baseline and changes in invasive and non-invasive haemodynamic variables to predict fluid responsiveness with calculation of the area under the ROC curve (AUC), using the MedCalc software for Windows, version 11.3.3 (Medcalc Software, Mariakerke, Belgium). The optimum cutoff values with highest combined sensitivity and specificity were defined and AUCs were compared where appropriate. Negative predictive values (NPV) and positive predictive values (PPV) were calculated. Binary logistic regression was used to calculate an odds ratio (OR) and 95% confidence intervals (CIs). A P value of <0.05 was considered statistically significant and exact P values are given, in three decimals if <0.1, unless <0.001. Data are presented as number [percentage] or median [interquartile range] where appropriate. Preliminary results have been published previously [18].
RESULTS
Data are presented according to fluid responsiveness (Table 1). Fluid responsiveness was defined as an increase in stroke volume index (SVI) at T = 2 of >10% compared with baseline (T = 2–T = 0), while classification according to an SVI increase of >10% from T = 1−T = 0 did not change the distribution of patients among response groups. Three patients were excluded during the study period because of increased fluid needs on clinical grounds following hypotension. Thirty-two patients were included for final analysis. Forty-four percentage (n = 14) of the patients had an increased SVI >10% and were classified fluid responsive. Groups did not differ in demographic or surgical characteristics, use of vasopressor and inotropic medication (Table 1). Both on- and off-pump cardiac surgery had been applied.
. | No response, n = 18 . | Response, n = 14 . | P . |
---|---|---|---|
Gender, female | 2 [11] | 2 [14] | 1.00 |
Age (years) | 66 [10] | 64 [19] | 0.48 |
Body weight (kg) | 90 [22] | 85 [15] | 0.76 |
Body mass index (kg m−2) | 27.4 [4.6] | 25.9 [7.7] | 0.73 |
Premorbidity | |||
Diabetes mellitus | 6 [33] | 4 [29] | 1.00 |
Pre-existent arterial hypertension | 12 [67] | 10 [71] | 1.00 |
Dyslipidaemia | 9 [50] | 10 [71] | 0.46 |
Smoker/non-smoker <1 year/non-smoker | 2/3/13 | 1/3/10 | 0.89 |
Surgery | |||
Off-pump | 4 [22] | 8 [57] | 0.068 |
Bypasses (number) | 4 [3–5] | 4 [2–7] | 0.48 |
Duration of surgery (min) | 280 [95] | 238 [80] | 0.14 |
Pump time (min) | 111 [86] | 0 [120] | 0.13 |
Aorta cross clamp time (min) | 62 [89] | 0 [93] | 0.38 |
Intensive care unit (ICU) | |||
Duration ICU admission (h) | 20.7 [5.5] | 22.5 [5.7] | 0.30 |
Mechanical ventilation (min) | 370 [211] | 249 [228] | 0.47 |
Dopamine (n) | 16 [89] | 11 [79] | 0.63 |
(mg h−1) | 10 [7] | 8 [8] | 0.70 |
Respiratory rate (b. min−1) | 14 [7] | 14 [3] | 0.92 |
Positive end-expiratory pressure (cmH2O) | 5 [3] | 5 [2] | 0.37 |
Peak airway pressure (cmH2O) | 17 [4] | 18 [7] | 0.38 |
Mean pressure (cmH2O) | 9 [3] | 9 [2] | 0.86 |
Tidal volume (ml) | 493 [65] | 467 [96] | 0.52 |
. | No response, n = 18 . | Response, n = 14 . | P . |
---|---|---|---|
Gender, female | 2 [11] | 2 [14] | 1.00 |
Age (years) | 66 [10] | 64 [19] | 0.48 |
Body weight (kg) | 90 [22] | 85 [15] | 0.76 |
Body mass index (kg m−2) | 27.4 [4.6] | 25.9 [7.7] | 0.73 |
Premorbidity | |||
Diabetes mellitus | 6 [33] | 4 [29] | 1.00 |
Pre-existent arterial hypertension | 12 [67] | 10 [71] | 1.00 |
Dyslipidaemia | 9 [50] | 10 [71] | 0.46 |
Smoker/non-smoker <1 year/non-smoker | 2/3/13 | 1/3/10 | 0.89 |
Surgery | |||
Off-pump | 4 [22] | 8 [57] | 0.068 |
Bypasses (number) | 4 [3–5] | 4 [2–7] | 0.48 |
Duration of surgery (min) | 280 [95] | 238 [80] | 0.14 |
Pump time (min) | 111 [86] | 0 [120] | 0.13 |
Aorta cross clamp time (min) | 62 [89] | 0 [93] | 0.38 |
Intensive care unit (ICU) | |||
Duration ICU admission (h) | 20.7 [5.5] | 22.5 [5.7] | 0.30 |
Mechanical ventilation (min) | 370 [211] | 249 [228] | 0.47 |
Dopamine (n) | 16 [89] | 11 [79] | 0.63 |
(mg h−1) | 10 [7] | 8 [8] | 0.70 |
Respiratory rate (b. min−1) | 14 [7] | 14 [3] | 0.92 |
Positive end-expiratory pressure (cmH2O) | 5 [3] | 5 [2] | 0.37 |
Peak airway pressure (cmH2O) | 17 [4] | 18 [7] | 0.38 |
Mean pressure (cmH2O) | 9 [3] | 9 [2] | 0.86 |
Tidal volume (ml) | 493 [65] | 467 [96] | 0.52 |
Values are median [interquartile range] or number [percentage], where appropriate.
. | No response, n = 18 . | Response, n = 14 . | P . |
---|---|---|---|
Gender, female | 2 [11] | 2 [14] | 1.00 |
Age (years) | 66 [10] | 64 [19] | 0.48 |
Body weight (kg) | 90 [22] | 85 [15] | 0.76 |
Body mass index (kg m−2) | 27.4 [4.6] | 25.9 [7.7] | 0.73 |
Premorbidity | |||
Diabetes mellitus | 6 [33] | 4 [29] | 1.00 |
Pre-existent arterial hypertension | 12 [67] | 10 [71] | 1.00 |
Dyslipidaemia | 9 [50] | 10 [71] | 0.46 |
Smoker/non-smoker <1 year/non-smoker | 2/3/13 | 1/3/10 | 0.89 |
Surgery | |||
Off-pump | 4 [22] | 8 [57] | 0.068 |
Bypasses (number) | 4 [3–5] | 4 [2–7] | 0.48 |
Duration of surgery (min) | 280 [95] | 238 [80] | 0.14 |
Pump time (min) | 111 [86] | 0 [120] | 0.13 |
Aorta cross clamp time (min) | 62 [89] | 0 [93] | 0.38 |
Intensive care unit (ICU) | |||
Duration ICU admission (h) | 20.7 [5.5] | 22.5 [5.7] | 0.30 |
Mechanical ventilation (min) | 370 [211] | 249 [228] | 0.47 |
Dopamine (n) | 16 [89] | 11 [79] | 0.63 |
(mg h−1) | 10 [7] | 8 [8] | 0.70 |
Respiratory rate (b. min−1) | 14 [7] | 14 [3] | 0.92 |
Positive end-expiratory pressure (cmH2O) | 5 [3] | 5 [2] | 0.37 |
Peak airway pressure (cmH2O) | 17 [4] | 18 [7] | 0.38 |
Mean pressure (cmH2O) | 9 [3] | 9 [2] | 0.86 |
Tidal volume (ml) | 493 [65] | 467 [96] | 0.52 |
. | No response, n = 18 . | Response, n = 14 . | P . |
---|---|---|---|
Gender, female | 2 [11] | 2 [14] | 1.00 |
Age (years) | 66 [10] | 64 [19] | 0.48 |
Body weight (kg) | 90 [22] | 85 [15] | 0.76 |
Body mass index (kg m−2) | 27.4 [4.6] | 25.9 [7.7] | 0.73 |
Premorbidity | |||
Diabetes mellitus | 6 [33] | 4 [29] | 1.00 |
Pre-existent arterial hypertension | 12 [67] | 10 [71] | 1.00 |
Dyslipidaemia | 9 [50] | 10 [71] | 0.46 |
Smoker/non-smoker <1 year/non-smoker | 2/3/13 | 1/3/10 | 0.89 |
Surgery | |||
Off-pump | 4 [22] | 8 [57] | 0.068 |
Bypasses (number) | 4 [3–5] | 4 [2–7] | 0.48 |
Duration of surgery (min) | 280 [95] | 238 [80] | 0.14 |
Pump time (min) | 111 [86] | 0 [120] | 0.13 |
Aorta cross clamp time (min) | 62 [89] | 0 [93] | 0.38 |
Intensive care unit (ICU) | |||
Duration ICU admission (h) | 20.7 [5.5] | 22.5 [5.7] | 0.30 |
Mechanical ventilation (min) | 370 [211] | 249 [228] | 0.47 |
Dopamine (n) | 16 [89] | 11 [79] | 0.63 |
(mg h−1) | 10 [7] | 8 [8] | 0.70 |
Respiratory rate (b. min−1) | 14 [7] | 14 [3] | 0.92 |
Positive end-expiratory pressure (cmH2O) | 5 [3] | 5 [2] | 0.37 |
Peak airway pressure (cmH2O) | 17 [4] | 18 [7] | 0.38 |
Mean pressure (cmH2O) | 9 [3] | 9 [2] | 0.86 |
Tidal volume (ml) | 493 [65] | 467 [96] | 0.52 |
Values are median [interquartile range] or number [percentage], where appropriate.
Haemodynamic parameters
The HR decreased more in responders than non-responders (P < 0.001) (Table 2). At baseline SAP, MAP and SV were lower in responders. A decrease in HR during fluid infusion correlated with an increase in SVI, and baseline values for SAP and MAP negatively correlated with the SVI increase (Table 3). SvO2 showed an increase in responders, while decreasing in non-responders, correlating with the concomitant change in SVI (Tables 2 and 3).
. | No response (n = 18) . | Response (n = 14) . | P . |
---|---|---|---|
HR (b min−1) | |||
T0 | 75 [21] | 82 [12] | 0.23 |
T1 | 74 [20] | 80 [13] | 0.57 |
T2 | 73 [19] | 77 [47] | 0.80 |
T2–T0 | −1 [5] | −4 [4] | <0.001 |
SAP (mmHg) | |||
T0 | 112 [18] | 91 [10] | <0.001 |
T1 | 117 [29] | 107 [23] | 0.16 |
T2 | 127 [29] | 111 [19] | 0.091 |
T2–T0 | 16 [31] | 19 [18] | 0.25 |
MAP (mmHg) | |||
T0 | 74 [14] | 63 [8] | 0.001 |
T1 | 76 [15] | 72 [11] | 0.19 |
T2 | 82 [13] | 75 [9] | 0.091 |
T2–T0 | 9 [20] | 11 [12] | 0.32 |
CVP (cmH2O) | |||
T0 | 6 [3] | 6 [5] | 0.46 |
T1 | 6 [3] | 7 [4] | 0.38 |
T2 | 6 [2] | 7 [4] | 0.60 |
T2–T0 | 1 [2] | 0 [2] | 0.45 |
PAOP (mmHg) | |||
T0 | 8 [6] | 9 [7] | 0.89 |
T1 | 11 [6] | 10 [3] | 0.35 |
T2 | 10 [5] | 9 [4] | 0.55 |
T2–T0 | 1 [5] | 2 [4] | 0.77 |
CO (ml min−1) | |||
T0 | 5.4 [2.3] | 4.8 [2.4] | 0.17 |
T1 | 5.3 [2.0] | 5.3 [1.9] | 0.88 |
T2 | 5.2 [2.1] | 5.6 [2.8] | 0.62 |
T2–T0 | −0.3 [0.42] | 0.9 [0.6] | <0.001 |
SV (ml) | |||
T0 | 71.2 [34.3] | 58.7 [25.8] | 0.04 |
T1 | 69.3 [24.9] | 66.9 [35.5] | 0.91 |
T2 | 63.9 [28.0] | 73.5 [44.1] | 0.54 |
T2–T0 | −4.7 [8.1] | 13.3 [8.5] | <0.001 |
SvO2 | |||
T0 | 0.76 [0.09] | 0.71 [0.09] | 0.34 |
T1 | 0.72 [0.12] | 0.71 [0.11] | 0.66 |
T2 | 0.68 [0.09] | 0.72 [0.06] | 0.04 |
T2–T0 | −0.03 [0.08] | 0.01 [0.06] | 0.014 |
PEP (ms) | |||
T0 | 185 [14] | 198 [25] | 0.08 |
T1 | 182 [19] | 189 [18] | 0.21 |
T2 | 174 [22] | 186 [26] | 0.068 |
T2–T0 | −8 [16] | −4 [23] | 0.73 |
dPEP (%) | |||
T0 | 12.8 [6.2] | 15.0 [7.4] | 0.29 |
T1 | 7.2 [2.9] | 8.7 [4.6] | 0.29 |
T2 | 7.0 [4.8] | 7.9 [3.6] | 0.97 |
T2–T0 | −5.6 [7.2] | −6.5 [5.7] | 0.38 |
ISTI (ms) | |||
T0 | 145 [24] | 158 [25] | 0.04 |
T1 | 147 [29] | 153 [36] | 0.49 |
T2 | 147 [29] | 152 [34] | 0.60 |
T2–T0 | 5 [13] | −7 [17] | 0.012 |
dISTI (%) | |||
T0 | 22.4 [17.8] | 21.2 [27.4] | 0.70 |
T1 | 15.2 [13.1] | 15.2 [6.5] | 0.82 |
T2 | 17.4 [7.4] | 15.6 [10.9] | 0.79 |
T2–T0 | −5.4 [10.5] | −4.7 [20.6] | 0.91 |
. | No response (n = 18) . | Response (n = 14) . | P . |
---|---|---|---|
HR (b min−1) | |||
T0 | 75 [21] | 82 [12] | 0.23 |
T1 | 74 [20] | 80 [13] | 0.57 |
T2 | 73 [19] | 77 [47] | 0.80 |
T2–T0 | −1 [5] | −4 [4] | <0.001 |
SAP (mmHg) | |||
T0 | 112 [18] | 91 [10] | <0.001 |
T1 | 117 [29] | 107 [23] | 0.16 |
T2 | 127 [29] | 111 [19] | 0.091 |
T2–T0 | 16 [31] | 19 [18] | 0.25 |
MAP (mmHg) | |||
T0 | 74 [14] | 63 [8] | 0.001 |
T1 | 76 [15] | 72 [11] | 0.19 |
T2 | 82 [13] | 75 [9] | 0.091 |
T2–T0 | 9 [20] | 11 [12] | 0.32 |
CVP (cmH2O) | |||
T0 | 6 [3] | 6 [5] | 0.46 |
T1 | 6 [3] | 7 [4] | 0.38 |
T2 | 6 [2] | 7 [4] | 0.60 |
T2–T0 | 1 [2] | 0 [2] | 0.45 |
PAOP (mmHg) | |||
T0 | 8 [6] | 9 [7] | 0.89 |
T1 | 11 [6] | 10 [3] | 0.35 |
T2 | 10 [5] | 9 [4] | 0.55 |
T2–T0 | 1 [5] | 2 [4] | 0.77 |
CO (ml min−1) | |||
T0 | 5.4 [2.3] | 4.8 [2.4] | 0.17 |
T1 | 5.3 [2.0] | 5.3 [1.9] | 0.88 |
T2 | 5.2 [2.1] | 5.6 [2.8] | 0.62 |
T2–T0 | −0.3 [0.42] | 0.9 [0.6] | <0.001 |
SV (ml) | |||
T0 | 71.2 [34.3] | 58.7 [25.8] | 0.04 |
T1 | 69.3 [24.9] | 66.9 [35.5] | 0.91 |
T2 | 63.9 [28.0] | 73.5 [44.1] | 0.54 |
T2–T0 | −4.7 [8.1] | 13.3 [8.5] | <0.001 |
SvO2 | |||
T0 | 0.76 [0.09] | 0.71 [0.09] | 0.34 |
T1 | 0.72 [0.12] | 0.71 [0.11] | 0.66 |
T2 | 0.68 [0.09] | 0.72 [0.06] | 0.04 |
T2–T0 | −0.03 [0.08] | 0.01 [0.06] | 0.014 |
PEP (ms) | |||
T0 | 185 [14] | 198 [25] | 0.08 |
T1 | 182 [19] | 189 [18] | 0.21 |
T2 | 174 [22] | 186 [26] | 0.068 |
T2–T0 | −8 [16] | −4 [23] | 0.73 |
dPEP (%) | |||
T0 | 12.8 [6.2] | 15.0 [7.4] | 0.29 |
T1 | 7.2 [2.9] | 8.7 [4.6] | 0.29 |
T2 | 7.0 [4.8] | 7.9 [3.6] | 0.97 |
T2–T0 | −5.6 [7.2] | −6.5 [5.7] | 0.38 |
ISTI (ms) | |||
T0 | 145 [24] | 158 [25] | 0.04 |
T1 | 147 [29] | 153 [36] | 0.49 |
T2 | 147 [29] | 152 [34] | 0.60 |
T2–T0 | 5 [13] | −7 [17] | 0.012 |
dISTI (%) | |||
T0 | 22.4 [17.8] | 21.2 [27.4] | 0.70 |
T1 | 15.2 [13.1] | 15.2 [6.5] | 0.82 |
T2 | 17.4 [7.4] | 15.6 [10.9] | 0.79 |
T2–T0 | −5.4 [10.5] | −4.7 [20.6] | 0.91 |
Values are median [interquartile range]. HR: heart rate; SAP: systolic arterial pressure; MAP: mean arterial pressure; CVP: central venous pressure; PAOP: pulmonary artery occlusion pressure; CO: cardiac output; SV: stroke volume; SvO2: mixed venous O2 saturation; PEP: mean pre-ejection period; dPEP: respiratory variation in PEP; ISTI: mean initial systolic time interval; dISTI: respiratory variation in ISTI; T0: baseline measurement; T1: after the first volume loading step (VLS) of 250 ml Gelofusin®; T2: after the second VLS.
. | No response (n = 18) . | Response (n = 14) . | P . |
---|---|---|---|
HR (b min−1) | |||
T0 | 75 [21] | 82 [12] | 0.23 |
T1 | 74 [20] | 80 [13] | 0.57 |
T2 | 73 [19] | 77 [47] | 0.80 |
T2–T0 | −1 [5] | −4 [4] | <0.001 |
SAP (mmHg) | |||
T0 | 112 [18] | 91 [10] | <0.001 |
T1 | 117 [29] | 107 [23] | 0.16 |
T2 | 127 [29] | 111 [19] | 0.091 |
T2–T0 | 16 [31] | 19 [18] | 0.25 |
MAP (mmHg) | |||
T0 | 74 [14] | 63 [8] | 0.001 |
T1 | 76 [15] | 72 [11] | 0.19 |
T2 | 82 [13] | 75 [9] | 0.091 |
T2–T0 | 9 [20] | 11 [12] | 0.32 |
CVP (cmH2O) | |||
T0 | 6 [3] | 6 [5] | 0.46 |
T1 | 6 [3] | 7 [4] | 0.38 |
T2 | 6 [2] | 7 [4] | 0.60 |
T2–T0 | 1 [2] | 0 [2] | 0.45 |
PAOP (mmHg) | |||
T0 | 8 [6] | 9 [7] | 0.89 |
T1 | 11 [6] | 10 [3] | 0.35 |
T2 | 10 [5] | 9 [4] | 0.55 |
T2–T0 | 1 [5] | 2 [4] | 0.77 |
CO (ml min−1) | |||
T0 | 5.4 [2.3] | 4.8 [2.4] | 0.17 |
T1 | 5.3 [2.0] | 5.3 [1.9] | 0.88 |
T2 | 5.2 [2.1] | 5.6 [2.8] | 0.62 |
T2–T0 | −0.3 [0.42] | 0.9 [0.6] | <0.001 |
SV (ml) | |||
T0 | 71.2 [34.3] | 58.7 [25.8] | 0.04 |
T1 | 69.3 [24.9] | 66.9 [35.5] | 0.91 |
T2 | 63.9 [28.0] | 73.5 [44.1] | 0.54 |
T2–T0 | −4.7 [8.1] | 13.3 [8.5] | <0.001 |
SvO2 | |||
T0 | 0.76 [0.09] | 0.71 [0.09] | 0.34 |
T1 | 0.72 [0.12] | 0.71 [0.11] | 0.66 |
T2 | 0.68 [0.09] | 0.72 [0.06] | 0.04 |
T2–T0 | −0.03 [0.08] | 0.01 [0.06] | 0.014 |
PEP (ms) | |||
T0 | 185 [14] | 198 [25] | 0.08 |
T1 | 182 [19] | 189 [18] | 0.21 |
T2 | 174 [22] | 186 [26] | 0.068 |
T2–T0 | −8 [16] | −4 [23] | 0.73 |
dPEP (%) | |||
T0 | 12.8 [6.2] | 15.0 [7.4] | 0.29 |
T1 | 7.2 [2.9] | 8.7 [4.6] | 0.29 |
T2 | 7.0 [4.8] | 7.9 [3.6] | 0.97 |
T2–T0 | −5.6 [7.2] | −6.5 [5.7] | 0.38 |
ISTI (ms) | |||
T0 | 145 [24] | 158 [25] | 0.04 |
T1 | 147 [29] | 153 [36] | 0.49 |
T2 | 147 [29] | 152 [34] | 0.60 |
T2–T0 | 5 [13] | −7 [17] | 0.012 |
dISTI (%) | |||
T0 | 22.4 [17.8] | 21.2 [27.4] | 0.70 |
T1 | 15.2 [13.1] | 15.2 [6.5] | 0.82 |
T2 | 17.4 [7.4] | 15.6 [10.9] | 0.79 |
T2–T0 | −5.4 [10.5] | −4.7 [20.6] | 0.91 |
. | No response (n = 18) . | Response (n = 14) . | P . |
---|---|---|---|
HR (b min−1) | |||
T0 | 75 [21] | 82 [12] | 0.23 |
T1 | 74 [20] | 80 [13] | 0.57 |
T2 | 73 [19] | 77 [47] | 0.80 |
T2–T0 | −1 [5] | −4 [4] | <0.001 |
SAP (mmHg) | |||
T0 | 112 [18] | 91 [10] | <0.001 |
T1 | 117 [29] | 107 [23] | 0.16 |
T2 | 127 [29] | 111 [19] | 0.091 |
T2–T0 | 16 [31] | 19 [18] | 0.25 |
MAP (mmHg) | |||
T0 | 74 [14] | 63 [8] | 0.001 |
T1 | 76 [15] | 72 [11] | 0.19 |
T2 | 82 [13] | 75 [9] | 0.091 |
T2–T0 | 9 [20] | 11 [12] | 0.32 |
CVP (cmH2O) | |||
T0 | 6 [3] | 6 [5] | 0.46 |
T1 | 6 [3] | 7 [4] | 0.38 |
T2 | 6 [2] | 7 [4] | 0.60 |
T2–T0 | 1 [2] | 0 [2] | 0.45 |
PAOP (mmHg) | |||
T0 | 8 [6] | 9 [7] | 0.89 |
T1 | 11 [6] | 10 [3] | 0.35 |
T2 | 10 [5] | 9 [4] | 0.55 |
T2–T0 | 1 [5] | 2 [4] | 0.77 |
CO (ml min−1) | |||
T0 | 5.4 [2.3] | 4.8 [2.4] | 0.17 |
T1 | 5.3 [2.0] | 5.3 [1.9] | 0.88 |
T2 | 5.2 [2.1] | 5.6 [2.8] | 0.62 |
T2–T0 | −0.3 [0.42] | 0.9 [0.6] | <0.001 |
SV (ml) | |||
T0 | 71.2 [34.3] | 58.7 [25.8] | 0.04 |
T1 | 69.3 [24.9] | 66.9 [35.5] | 0.91 |
T2 | 63.9 [28.0] | 73.5 [44.1] | 0.54 |
T2–T0 | −4.7 [8.1] | 13.3 [8.5] | <0.001 |
SvO2 | |||
T0 | 0.76 [0.09] | 0.71 [0.09] | 0.34 |
T1 | 0.72 [0.12] | 0.71 [0.11] | 0.66 |
T2 | 0.68 [0.09] | 0.72 [0.06] | 0.04 |
T2–T0 | −0.03 [0.08] | 0.01 [0.06] | 0.014 |
PEP (ms) | |||
T0 | 185 [14] | 198 [25] | 0.08 |
T1 | 182 [19] | 189 [18] | 0.21 |
T2 | 174 [22] | 186 [26] | 0.068 |
T2–T0 | −8 [16] | −4 [23] | 0.73 |
dPEP (%) | |||
T0 | 12.8 [6.2] | 15.0 [7.4] | 0.29 |
T1 | 7.2 [2.9] | 8.7 [4.6] | 0.29 |
T2 | 7.0 [4.8] | 7.9 [3.6] | 0.97 |
T2–T0 | −5.6 [7.2] | −6.5 [5.7] | 0.38 |
ISTI (ms) | |||
T0 | 145 [24] | 158 [25] | 0.04 |
T1 | 147 [29] | 153 [36] | 0.49 |
T2 | 147 [29] | 152 [34] | 0.60 |
T2–T0 | 5 [13] | −7 [17] | 0.012 |
dISTI (%) | |||
T0 | 22.4 [17.8] | 21.2 [27.4] | 0.70 |
T1 | 15.2 [13.1] | 15.2 [6.5] | 0.82 |
T2 | 17.4 [7.4] | 15.6 [10.9] | 0.79 |
T2–T0 | −5.4 [10.5] | −4.7 [20.6] | 0.91 |
Values are median [interquartile range]. HR: heart rate; SAP: systolic arterial pressure; MAP: mean arterial pressure; CVP: central venous pressure; PAOP: pulmonary artery occlusion pressure; CO: cardiac output; SV: stroke volume; SvO2: mixed venous O2 saturation; PEP: mean pre-ejection period; dPEP: respiratory variation in PEP; ISTI: mean initial systolic time interval; dISTI: respiratory variation in ISTI; T0: baseline measurement; T1: after the first volume loading step (VLS) of 250 ml Gelofusin®; T2: after the second VLS.
. | rs . | P . |
---|---|---|
HR (b min−1) | ||
T0 | 0.37 | 0.04 |
T2−T0 | −0.79 | <0.001 |
SAP (mmHg) | ||
T0 | −0.56 | 0.001 |
T2–T0 | 0.17 | 0.35 |
MAP (mmHg) | ||
T0 | −0.49 | 0.004 |
T2–T0 | 0.16 | 0.39 |
CVP (cmH2O) | ||
T0 | −0.18 | 0.34 |
T2–T0 | 0.08 | 0.66 |
SV (ml) | ||
T0 | −0.43 | 0.014 |
SvO2 | ||
T0 | −0.09 | 0.64 |
T2–T0 | 0.46 | 0.017 |
PEP (ms) | ||
T0 | 0.29 | 0.11 |
T2–T0 | 0.05 | 0.79 |
dPEP (%) | ||
T0 | 0.06 | 0.76 |
T2–T0 | −0.05 | 0.81 |
ISTI (ms) | ||
T0 | 0.27 | 0.14 |
T2–T0 | −0.57 | 0.001 |
dISTI (%) | ||
T0 | 0.05 | 0.79 |
T2–T0 | −0.05 | 0.81 |
. | rs . | P . |
---|---|---|
HR (b min−1) | ||
T0 | 0.37 | 0.04 |
T2−T0 | −0.79 | <0.001 |
SAP (mmHg) | ||
T0 | −0.56 | 0.001 |
T2–T0 | 0.17 | 0.35 |
MAP (mmHg) | ||
T0 | −0.49 | 0.004 |
T2–T0 | 0.16 | 0.39 |
CVP (cmH2O) | ||
T0 | −0.18 | 0.34 |
T2–T0 | 0.08 | 0.66 |
SV (ml) | ||
T0 | −0.43 | 0.014 |
SvO2 | ||
T0 | −0.09 | 0.64 |
T2–T0 | 0.46 | 0.017 |
PEP (ms) | ||
T0 | 0.29 | 0.11 |
T2–T0 | 0.05 | 0.79 |
dPEP (%) | ||
T0 | 0.06 | 0.76 |
T2–T0 | −0.05 | 0.81 |
ISTI (ms) | ||
T0 | 0.27 | 0.14 |
T2–T0 | −0.57 | 0.001 |
dISTI (%) | ||
T0 | 0.05 | 0.79 |
T2–T0 | −0.05 | 0.81 |
rs: Spearman's rho correlation; SVI: stroke volume index; HR: heart rate; SAP: systolic arterial pressure; MAP: mean arterial pressure; CVP: central venous pressure; SV: stroke volume; SvO2: mixed venous O2 saturation; PEP: mean pre-ejection period; dPEP: respiratory variation of PEP; ISTI: mean initial systolic time interval; dISTI: respiratory variation of ISTI; T0: baseline measurement; T2: after 500 ml of Gelofusin® infusion.
. | rs . | P . |
---|---|---|
HR (b min−1) | ||
T0 | 0.37 | 0.04 |
T2−T0 | −0.79 | <0.001 |
SAP (mmHg) | ||
T0 | −0.56 | 0.001 |
T2–T0 | 0.17 | 0.35 |
MAP (mmHg) | ||
T0 | −0.49 | 0.004 |
T2–T0 | 0.16 | 0.39 |
CVP (cmH2O) | ||
T0 | −0.18 | 0.34 |
T2–T0 | 0.08 | 0.66 |
SV (ml) | ||
T0 | −0.43 | 0.014 |
SvO2 | ||
T0 | −0.09 | 0.64 |
T2–T0 | 0.46 | 0.017 |
PEP (ms) | ||
T0 | 0.29 | 0.11 |
T2–T0 | 0.05 | 0.79 |
dPEP (%) | ||
T0 | 0.06 | 0.76 |
T2–T0 | −0.05 | 0.81 |
ISTI (ms) | ||
T0 | 0.27 | 0.14 |
T2–T0 | −0.57 | 0.001 |
dISTI (%) | ||
T0 | 0.05 | 0.79 |
T2–T0 | −0.05 | 0.81 |
. | rs . | P . |
---|---|---|
HR (b min−1) | ||
T0 | 0.37 | 0.04 |
T2−T0 | −0.79 | <0.001 |
SAP (mmHg) | ||
T0 | −0.56 | 0.001 |
T2–T0 | 0.17 | 0.35 |
MAP (mmHg) | ||
T0 | −0.49 | 0.004 |
T2–T0 | 0.16 | 0.39 |
CVP (cmH2O) | ||
T0 | −0.18 | 0.34 |
T2–T0 | 0.08 | 0.66 |
SV (ml) | ||
T0 | −0.43 | 0.014 |
SvO2 | ||
T0 | −0.09 | 0.64 |
T2–T0 | 0.46 | 0.017 |
PEP (ms) | ||
T0 | 0.29 | 0.11 |
T2–T0 | 0.05 | 0.79 |
dPEP (%) | ||
T0 | 0.06 | 0.76 |
T2–T0 | −0.05 | 0.81 |
ISTI (ms) | ||
T0 | 0.27 | 0.14 |
T2–T0 | −0.57 | 0.001 |
dISTI (%) | ||
T0 | 0.05 | 0.79 |
T2–T0 | −0.05 | 0.81 |
rs: Spearman's rho correlation; SVI: stroke volume index; HR: heart rate; SAP: systolic arterial pressure; MAP: mean arterial pressure; CVP: central venous pressure; SV: stroke volume; SvO2: mixed venous O2 saturation; PEP: mean pre-ejection period; dPEP: respiratory variation of PEP; ISTI: mean initial systolic time interval; dISTI: respiratory variation of ISTI; T0: baseline measurement; T2: after 500 ml of Gelofusin® infusion.
Initial systolic time interval and pre-ejection period
In responders, ISTI at baseline was higher and decreased during fluid infusion while it tended to increase in non-responders (Fig. 3). A decrease in ISTI with fluid infusion negatively correlated with an increase in SVI (Table 3). PEP did not differ between response groups. PEP and ISTI correlated at baseline (rs = 0.35, P = 0.049). For pooled measurements per volume-loading step, the change in PEP and ISTI correlated (rs = 0.39, P = 0.002, respectively). The dISTI and dPEP did not differ between responders and non-responders, yet correlated at baseline (rs = 0.38, P = 0.034).
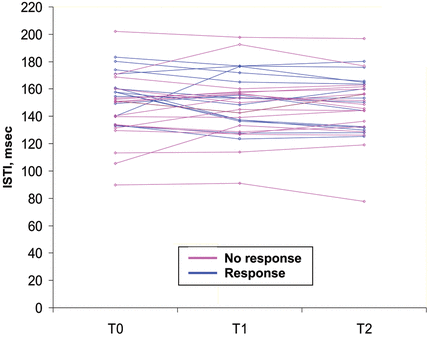
The ISTI in responders and non-responders at baseline (T0) and after each volume loading step of 250 ml of Gelofusin® (T1 and T2). A response was defined as an increase in SVI >10% after 500 ml of fluid infusion.
Predicting fluid responsiveness
Baseline SAP and MAP predicted fluid responsiveness (Table 4). A SV of ≤67.5 ml also predicted it. Baseline ISTI predicted fluid responsiveness with an optimum threshold of >153 ms. The AUC of the ROC curve for ISTI (Fig. 4) did not differ from those of SAP, MAP or SV at baseline (P = 0.097, P = 0.28 and P = 0.99, respectively). A decrease in ISTI of ≥8.3 ms predicted fluid responsiveness, yielding the highest specificity and PPV among the variables. The OR for fluid responsiveness was 12.7 (95% CIs 1.3–124, P = 0.028). The AUC of the ROC curve of change in ISTI did not differ from those of SAP or MAP (P = 0.18 and P = 0.44, respectively). The AUCs of the ROC curves of changes in HR and SvO2 during fluid loading were 0.87 and 0.78 (P < 0.001 for both), respectively, and also did not differ from that of changes in ISTI (P = 0.13 and P = 0.15, respectively).
Receiver operating characteristic curve analyses of variables prior to and after fluid loading for subsequent increases in stroke volume
. | AUC . | P . | Optimum cut-off . | Sens. (%) . | Spec. (%) . | PPV (%) . | NPV (%) . |
---|---|---|---|---|---|---|---|
SAP T0 (mmHg) | 0.90 | <0.001 | ≤102 | 93 | 83 | 81 | 94 |
HR T2–T0 (b min−1) | 0.87 | <0.001 | ≤−3 | 86 | 78 | 75 | 88 |
MAP T0 (mmHg) | 0.85 | <0.001 | ≤69 | 93 | 72 | 72 | 93 |
SvO2T2–T0 | 0.78 | 0.003 | >0 | 75 | 80 | 75 | 80 |
ISTI T2–T0 (ms) | 0.76 | 0.004 | ≤−8.3 | 50 | 94 | 88 | 71 |
SV T0 (ml) | 0.71 | 0.022 | ≤67.5 | 79 | 61 | 62 | 79 |
ISTI T0 (ms) | 0.71 | 0.023 | >153 | 71 | 78 | 71 | 78 |
PEP T0 (ms) | 0.68 | 0.081 | >195 | 57 | 89 | 80 | 73 |
dPEP T0 (%) | 0.61 | 0.29 | >14.0 | 57 | 72 | 62 | 68 |
dISTI T0 (%) | 0.54 | 0.71 | >39.1 | 29 | 89 | 67 | 62 |
PEP T2–T0 (ms) | 0.54 | 0.74 | >0.73 | 36 | 83 | 63 | 63 |
. | AUC . | P . | Optimum cut-off . | Sens. (%) . | Spec. (%) . | PPV (%) . | NPV (%) . |
---|---|---|---|---|---|---|---|
SAP T0 (mmHg) | 0.90 | <0.001 | ≤102 | 93 | 83 | 81 | 94 |
HR T2–T0 (b min−1) | 0.87 | <0.001 | ≤−3 | 86 | 78 | 75 | 88 |
MAP T0 (mmHg) | 0.85 | <0.001 | ≤69 | 93 | 72 | 72 | 93 |
SvO2T2–T0 | 0.78 | 0.003 | >0 | 75 | 80 | 75 | 80 |
ISTI T2–T0 (ms) | 0.76 | 0.004 | ≤−8.3 | 50 | 94 | 88 | 71 |
SV T0 (ml) | 0.71 | 0.022 | ≤67.5 | 79 | 61 | 62 | 79 |
ISTI T0 (ms) | 0.71 | 0.023 | >153 | 71 | 78 | 71 | 78 |
PEP T0 (ms) | 0.68 | 0.081 | >195 | 57 | 89 | 80 | 73 |
dPEP T0 (%) | 0.61 | 0.29 | >14.0 | 57 | 72 | 62 | 68 |
dISTI T0 (%) | 0.54 | 0.71 | >39.1 | 29 | 89 | 67 | 62 |
PEP T2–T0 (ms) | 0.54 | 0.74 | >0.73 | 36 | 83 | 63 | 63 |
AUC: area under the ROC curve; Sens.: sensitivity; Spec.: specificity; PPV: positive predictive value; NPV: negative predictive value; SAP: systolic arterial pressure; HR: heart rate; MAP: mean arterial pressure; SvO2: mixed venous oxygen saturation; ISTI: mean initial systolic time interval; SV: stroke volume; PEP: mean pre-ejection period; dPEP: respiratory variation of PEP; dISTI: respiratory variation of ISTI. T0 = baseline. T2 = after fluid infusion.
Receiver operating characteristic curve analyses of variables prior to and after fluid loading for subsequent increases in stroke volume
. | AUC . | P . | Optimum cut-off . | Sens. (%) . | Spec. (%) . | PPV (%) . | NPV (%) . |
---|---|---|---|---|---|---|---|
SAP T0 (mmHg) | 0.90 | <0.001 | ≤102 | 93 | 83 | 81 | 94 |
HR T2–T0 (b min−1) | 0.87 | <0.001 | ≤−3 | 86 | 78 | 75 | 88 |
MAP T0 (mmHg) | 0.85 | <0.001 | ≤69 | 93 | 72 | 72 | 93 |
SvO2T2–T0 | 0.78 | 0.003 | >0 | 75 | 80 | 75 | 80 |
ISTI T2–T0 (ms) | 0.76 | 0.004 | ≤−8.3 | 50 | 94 | 88 | 71 |
SV T0 (ml) | 0.71 | 0.022 | ≤67.5 | 79 | 61 | 62 | 79 |
ISTI T0 (ms) | 0.71 | 0.023 | >153 | 71 | 78 | 71 | 78 |
PEP T0 (ms) | 0.68 | 0.081 | >195 | 57 | 89 | 80 | 73 |
dPEP T0 (%) | 0.61 | 0.29 | >14.0 | 57 | 72 | 62 | 68 |
dISTI T0 (%) | 0.54 | 0.71 | >39.1 | 29 | 89 | 67 | 62 |
PEP T2–T0 (ms) | 0.54 | 0.74 | >0.73 | 36 | 83 | 63 | 63 |
. | AUC . | P . | Optimum cut-off . | Sens. (%) . | Spec. (%) . | PPV (%) . | NPV (%) . |
---|---|---|---|---|---|---|---|
SAP T0 (mmHg) | 0.90 | <0.001 | ≤102 | 93 | 83 | 81 | 94 |
HR T2–T0 (b min−1) | 0.87 | <0.001 | ≤−3 | 86 | 78 | 75 | 88 |
MAP T0 (mmHg) | 0.85 | <0.001 | ≤69 | 93 | 72 | 72 | 93 |
SvO2T2–T0 | 0.78 | 0.003 | >0 | 75 | 80 | 75 | 80 |
ISTI T2–T0 (ms) | 0.76 | 0.004 | ≤−8.3 | 50 | 94 | 88 | 71 |
SV T0 (ml) | 0.71 | 0.022 | ≤67.5 | 79 | 61 | 62 | 79 |
ISTI T0 (ms) | 0.71 | 0.023 | >153 | 71 | 78 | 71 | 78 |
PEP T0 (ms) | 0.68 | 0.081 | >195 | 57 | 89 | 80 | 73 |
dPEP T0 (%) | 0.61 | 0.29 | >14.0 | 57 | 72 | 62 | 68 |
dISTI T0 (%) | 0.54 | 0.71 | >39.1 | 29 | 89 | 67 | 62 |
PEP T2–T0 (ms) | 0.54 | 0.74 | >0.73 | 36 | 83 | 63 | 63 |
AUC: area under the ROC curve; Sens.: sensitivity; Spec.: specificity; PPV: positive predictive value; NPV: negative predictive value; SAP: systolic arterial pressure; HR: heart rate; MAP: mean arterial pressure; SvO2: mixed venous oxygen saturation; ISTI: mean initial systolic time interval; SV: stroke volume; PEP: mean pre-ejection period; dPEP: respiratory variation of PEP; dISTI: respiratory variation of ISTI. T0 = baseline. T2 = after fluid infusion.
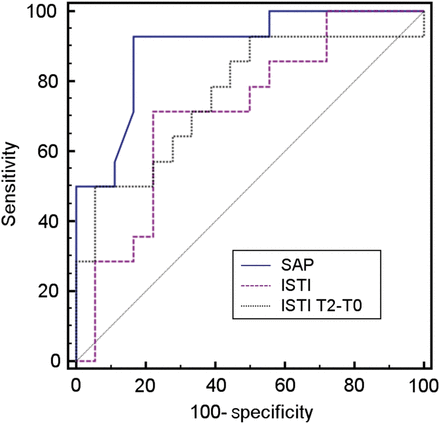
Receiver operating characteristic curves for cardiac response according to the systolic blood pressure (SAP; AUC = 0.90, P < 0.001) and baseline systolic time interval (ISTI; AUC = 0.71, P = 0.02) and increase in ISTI (T2–T0; AUC = 0.76, P = 0.004) during fluid infusion.
DISCUSSION
This study suggests that fluid responsiveness in patients after coronary artery surgery can be predicted and monitored non-invasively by ISTI, non-inferiorly to invasive measurements of blood pressures and flow.
In the present study, 44% of the patients were able to increase SVI >10%, defining fluid responsiveness upon infusion of 500 ml of gelatin solution, in line with the literature [2–4, 6–10]. This confirms that patients who seem hypovolaemic on clinical grounds are not always fluid responsive and are at risk of fluid overloading [3, 4]. To prevent harmful fluid overloading, various haemodynamic parameters have been studied, yet are invasive, technically challenging or not universally applicable, whereas variations in dynamic indices may not be caused by variations in SV alone, during mechanical ventilation [3–5, 6, 19]. Invasively measured SAP, MAP and SV prior to fluid loading were able to predict fluid responsiveness not significantly differently from non-invasive ISTI. Invasively measured low SAP and SV have been demonstrated previously to predict fluid responsiveness after cardiac surgery [10], but these values are not always available. Enlarging preload in the heart operating on the ascending limb of the Frank–Starling curve increases SV and thus SAP and MAP and decreases PEP [7, 8, 11]. PEP and dPEP have been shown to predict haemodynamic changes induced by positive end-expiratory pressure (PEEP) and fluid infusion, whereas indexing to tidal volumes or airway pressures has been shown to increase their predictive values in mechanically ventilated patients [12, 14, 15]. The present study could not reproduce these findings, since PEP did not vary among groups and could not predict fluid responsiveness, acknowledging differences in patient populations and research protocols. ISTI is seen as a surrogate for PEP and, indeed, the variables correlated, in concordance with the literature [17, 18]. PEP is affected by the time for pulse pressure to travel from the left ventricle to the intra-arterial catheter, depending on blood flow and vascular dimensions, whereas the ISTI is a more direct measure of electromechanical coupling of the heart. Fluid responsive patients showed a relatively high ISTI at baseline and a decrease during fluid administration (Fig. 3), associated with preload responsiveness of the heart. If ISTI declined >8.3 ms during fluid loading, a fluid response was highly likely (specificity 94%). This makes the ISTI not only suitable for predicting fluid responsiveness but also for non-invasive monitoring of a response to fluid infusion. At baseline, dISTI correlated to dPEP, yet did not vary among (non-responders). The low correlation coefficient suggests that other factors may be at play. Variations in pleural pressure and thus in venous return may have been too small to considerably help predicting fluid responsiveness by dISTI or dPEP, in the presence of relatively low tidal volumes and dynamic compliance of the respiratory system [4, 19].
Some limitations of the present study must be acknowledged. The systolic time intervals were determined manually during off-line analysis. However, ISTI could be automatically determined in the future by continuous on-line measurements at the bedside. Secondly, we do not know the applicability in other conditions and during spontaneous breathing. Future research may concentrate on the application in various clinical settings and patient groups and the supplementation of non-invasive echocardiography to evaluate the relationship of ISTI to volumetric indices. Future studies may also address use of real-time ISTI operated by nurses to prevent harmful overloading after cardiac surgery.
In conclusion, the present study suggests that non-invasively measured ISTI, a systolic time interval determined by ICG and ECG, can fairly accurately predict and monitor a response to fluid loading in clinically hypovolaemic patients after coronary surgery.
Conflict of interest: none declared.
REFERENCES
Author notes
Presented at the 14th Congress of the European Shock Society Congress in Sicily, Italy, September 2011, where it was granted the best poster award.