-
PDF
- Split View
-
Views
-
Cite
Cite
Inga Voges, Michael Jerosch-Herold, Christopher Hart, Jens Scheewe, Dominik Daniel Gabbert, Eileen Pardun, Hans-Heiner Kramer, Carsten Rickers, Anatomical and functional assessment of the intra-atrial lateral tunnel in the Fontan circulation, European Journal of Cardio-Thoracic Surgery, Volume 44, Issue 3, September 2013, Pages 462–467, https://doi.org/10.1093/ejcts/ezt066
- Share Icon Share
Abstract
In patients after completion of the total cavopulmonary connection (TCPC) with an intra-atrial lateral tunnel, deviations of the tunnel from an ideal straight tubular shape were observed. However, little is known about frequency and adverse effects of such shape deviations. We sought to analyse tunnel anatomy, dimensions and blood flow using cardiac magnetic resonance imaging (CMR).
Fifty-four patients with hypoplastic left heart syndrome (HLHS; mean age 6.0 ± 2.4 years) underwent CMR with gradient-echo cine sequences, 2D- and 3D-phase-contrast imaging. We analysed anatomy, diameters, cross-sectional areas, volumes and blood flow of the tunnel.
Twenty-five patients had a tubular tunnel. In 29 patients, bulging and/or narrowing of the tunnel were present. Cross-sectional areas and volumes of the tunnel were not significantly different between the two groups. There were also no differences for the mean blood flow and the mean and maximal flow velocity (P = 0.05–0.6). In all the patients, the normalized tunnel volume was related to age (r = 0.44; P = 0.002) and body surface area (BSA; r = 0.42; P = 0.005). The mean tunnel blood flow correlated with age (r = 0.73; P = 0.001) and BSA (r = 0.83; P < 0.0001).
A considerable percentage of patients with an intra-atrial lateral tunnel develop mild deviations of the tunnel from ideal tubular shape. The correlation between tunnel volume and mean blood flow with age and BSA suggests that the capacity of the tunnel adjusts to body growth, independent of tunnel shapes that deviate from a fluid-dynamically favourable shape. Follow-up CMRs are needed to detect long-term effects of irregular tunnel shapes on flow dynamics.
INTRODUCTION
Forty years after development of the Fontan procedure, early and intermediate survival has been improved up to >90% [1–3], and thus, long-term outcome is now of growing interest. de Leval et al. [4] suggested that minimizing of energy loss in the Fontan circulation is of crucial importance for long-term outcomes. They hypothesized that in Fontan patients with atriopulmonary connection increased power loss and blood flow turbulences are present and proposed a technical modification [4]. The so-called total cavopulmonary connection (TCPC) has the advantage of reduced energy loss because of a more streamlined flow through a tunnel and consequently reduced central venous pressures.
In patients with a functional single ventricle, a staged palliative surgery resulting in a TCPC has become the preferred treatment option [5]. Although the most used variants of the TCPC, the intra-atrial lateral tunnel and the extracardiac conduit, result in good long-term outcomes [2, 6], morphological deviations of the tunnel, such as (localized) dilatation, have been observed [7]. However, data on frequency, kind and potential clinically relevant effects of such shape deviations are scarce.
Our centre has a large volume of patients with hypoplastic left heart syndrome (HLHS) and a special follow-up programme for these patients. Therefore, we sought to use cardiac magnetic resonance imaging (CMR) as an accurate, non-invasive imaging method that provides 2-, 3- and multi-phase (temporally resolved) 3-dimensional (2D, 3D and 4D) anatomic and functional information [8–11], to evaluate anatomy, dimensions, volumes and flow profiles of the intra-atrial lateral tunnel in patients with HLHS.
MATERIALS AND METHODS
Patients
Fifty-four patients with HLHS (age 6.0 ± 2.4 (3.2–14.2) years), who underwent three-stage surgical palliation with creation of a fenestrated intra-atrial lateral tunnel at the third stage, were studied. All operations were done by the same cardiac surgeon. In all the patients, a hemi-Fontan operation was performed at the second step of the three-staged surgical palliation. During this procedure, a connection of the superior vena cava with the branch pulmonary arteries was constructed without surgical section of the superior vena cava. A side-by-side anastomosis between the right pulmonary artery and the posterior part of the cavo-atrial junction was created in addition to a ventral patchplasty of the pulmonary arteries. The inflow of the superior vena cava into the right atrium was occluded by using a polytetrafluoroethylene (PTFE) patch. The tunnel was constructed by using a PTFE patch which channels the inferior vena cava to the superior vena cava in all the patients.
A situs solitus and normal venous connection was present in all patients, enabling the construction of a straight tunnel. The age at operation was 2.6 ± 0.8 (1.1–4.9) years. The body weight was 20.2 ± 6.9 (13.5–49.0) kg. 31 (57%) patients had an open fenestration at the time of the study. In 23 patients (42%), percutanous closure of the fenestration was performed previously.
Informed consent was obtained from all the parents, as appropriate, and the study protocol conforms to the ethical guidelines of the 1975 Declaration of Helsinki as reflected in ‘a priori’ approval by the institution's human research committee.
Magnetic resonance imaging
The CMR studies were performed with a 3.0 Tesla scanner (Achieva 3.0T X-series, Philips Medical Systems, Netherlands) using a phased-array coil for cardiac imaging, or in the smallest children, a coil for extremities (SENSE™ Cardiac coil, SENSE™ Flex-L coil, Philips Medical Systems, Netherlands). All the patients required sedation with midazolame and propofol. Heart rate, respiratory motion, oxygen saturation and non-invasive blood pressure were recorded continuously during examination using a CMR compatible monitoring (Invivo Precess™, Invivo Corporation, Orlando, FL, USA).
Retrospective triggered gradient-echo cine imaging was acquired to analyse anatomy and dimensions (cross-sectional areas and volumes) of the intra-atrial lateral tunnel. Sequence parameters were: repetition time (TR)/echo time (TE) 4.4/2.5 ms, FOV 280 × 224 mm, voxel size 1.88 × 1.94 × 6 mm, slice thickness 5 mm and 25 phases per cardiac cycle. CMR images in axial, coronal, sagittal and short axis views were obtained.
ECG-gated 2D phase-contrast cine imaging was applied in patients with a closed fenestration to assess blood flow dynamics in the intra-atrial lateral tunnel; the pulse sequence parameters were: TR/TE = 4.4/2.7 ms, FOV: 270 × 270 mm, voxel size 1.64 × 1.4 × 7 mm and velocity encoding 80 cm/s.
So far, we used 3D phase-contrast imaging to visualize the flow pattern in the tunnel in 6 patients. The imaging parameters were as follows: TR/TE = 3.3/2.2 ms, FOV 320 mm, 12 slices and 25 heart phases.
Imaging processing and analysis
Dedicated CMR software (ViewForum release 6.3, Philips Medical Systems, Netherlands) was used to analyse 2D phase-contrast and gradient-echo cine images.
Diameters of the intra-atrial lateral tunnel were measured on axial, coronal and sagittal gradient-echo cine images at three different locations: (1) above the junction between the inferior vena cava and the tunnel, (2) at the level of the fenestration and (3) below the junction between the tunnel and the pulmonary arteries (Fig. 1). Furthermore, the minimal and maximal diameters and cross-sectional areas of the tunnel were measured. In order to classify the tunnel shape as more tubular or non-tubular, our patient cohort was divided at the median value of the difference between the mean area and the maximal and minimal area. In patients with a tubular tunnel, the difference between the mean area and the maximal and minimal area respectively was <15%, a value >15% defined a non-tubular tunnel. Two patients had a value of exactly 15% and were allocated to the group of patients with a non-tubular tunnel.
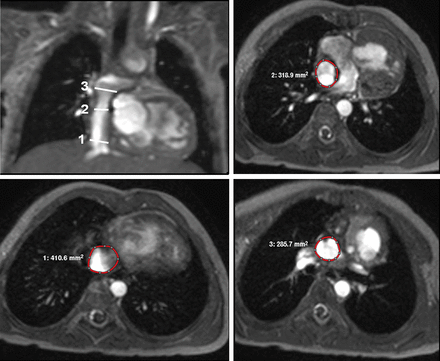
Sites of cross-sectional area measurement: (1) above the junction between the inferior vena cava and the intra-atrial lateral tunnel, (2) at the fenestration and (3) below the junction between the intra-atrial lateral tunnel and the pulmonary arteries.
Cross-sectional areas of the intra-atrial tunnel were measured on axial cine images at the same three locations as for diameters. The degree of deviation of the tunnel from a straight tubular shape was calculated by the following formula: Maximal area − minimal area/mean area.
Tunnel volumes were quantified using Simpson's rule and manual planimetry of axial cine images. The change in volume during the cardiac cycle was calculated by means of the following equation: .
Manual planimetry of short axis cine images was used to quantify right ventricular stroke volume (RVSV).
Tunnel blood flow was calculated from the 2D phase-contrast cine images in patients with a closed fenestration. Tunnel flow was measured below the connection of the tunnel with the pulmonary arteries. Therefore, contours were manually drawn around the regions of interest and subsequently mean flow rates were computed by averaging the flows through all cardiac phases.
The acquired 4D velocity data (3D plus time) were transferred to a commercially available software package (GTFlow release 1.4.13, GyroTools Ltd., Winterthur, Switzerland). Data analysis included blood flow visualization as 3D streamlines (streamlines represent the path of particles at each time point if the velocity field would remain static), 3D path lines (path lines visualize the path of particles in the dynamic flow velocity field) as well as 3D particle traces (particles illustrating spatial and temporal evolution of blood flow).
In some patients, measurements were not possible, because of susceptibility artefacts from implanted devices.
Statistical methods
Statistical analysis was performed using MedCalc (version 11.5.1.0 5, SPSS, Inc., Chicago, IL, USA). All continuous variables were described by mean values ± standard deviation (SD). Correlations were tested by Spearman's rank correlation analysis. Differences between subgroup of patients were analysed using the Mann–Whitney U-test. P values of <0.05 were considered to indicate statistical significance.
The authors had full access to and take full responsibility for the integrity of the data. All the authors have read and agreed to the manuscript as written.
RESULTS
Anatomy and dimensions of the intra-atrial lateral tunnel
Twenty-five (46%) patients had a intra-atrial lateral tunnel. In 29 patients (54%), a deviation of the intra-atrial lateral tunnel from a fluid-dynamically ideal straight tubular shape was found. Such shape deviations were characterized by bulging and/or narrowing of the tunnel at different locations (Fig. 2). In 24 patients (44%), bulging was particularly located in the lower third of the tunnel. There was no difference in age between patients with a tubular tunnel and patients with a non-tubular tunnel (5.85 ± 1.96 vs 6.07 ± 2.79 years, P = 0.58).
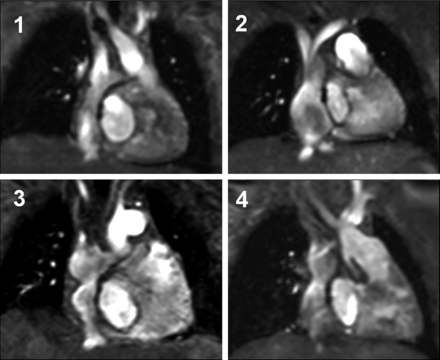
Morphology of the intra-atrial lateral tunnel: (1) tunnel with a tubular shape, (2–4) patients with deviation from a straight tubular tunnel.
Diameters and cross-sectional areas of the tunnel are listed in Table 1. The percentage areas of the tunnel compared with the size of the inferior vena cava were: (1) 122 ± 34% at the fenestration and (2) 112 ± 36% at the junction between tunnel and PA.
Measuring points . | Diameter (mm/m2) . | Cross-sectional area (mm2/m2) . | |||
---|---|---|---|---|---|
Coronal . | Sagittal . | Axial . | |||
Breadth . | Length . | ||||
At the junction between IVC and tunnel | 23.8 ± 4.0 | 21.0 ± 3.2 | 26.1 ± 4.3 | 24.6 ± 5.1 | 400.7 ± 103.1 |
At the fenestration | 23.5 ± 5.1 | 21.4 ± 7.5 | 24.6 ± 4.9 | 29.6 ± 6.2 | 462.7 ± 115.1 |
At the junction between tunnel and PA | 26.7 ± 4.1 | 22.4 ± 5.5 | 25.5 ± 4.8 | 25.2 ± 3.8 | 423.7 ± 111.6 |
Maximum | 28.2 ± 4.0 | 26.2 ± 4.2 | 28.6 ± 4.6 | 31.1 ± 5.3 | 511.6 ± 125.2 |
Minimum | 20.9 ± 6.2 | 18.7 ± 6.7 | 22.6 ± 3.7 | 22.9 ± 3.1 | 353.1 ± 67.6 |
Measuring points . | Diameter (mm/m2) . | Cross-sectional area (mm2/m2) . | |||
---|---|---|---|---|---|
Coronal . | Sagittal . | Axial . | |||
Breadth . | Length . | ||||
At the junction between IVC and tunnel | 23.8 ± 4.0 | 21.0 ± 3.2 | 26.1 ± 4.3 | 24.6 ± 5.1 | 400.7 ± 103.1 |
At the fenestration | 23.5 ± 5.1 | 21.4 ± 7.5 | 24.6 ± 4.9 | 29.6 ± 6.2 | 462.7 ± 115.1 |
At the junction between tunnel and PA | 26.7 ± 4.1 | 22.4 ± 5.5 | 25.5 ± 4.8 | 25.2 ± 3.8 | 423.7 ± 111.6 |
Maximum | 28.2 ± 4.0 | 26.2 ± 4.2 | 28.6 ± 4.6 | 31.1 ± 5.3 | 511.6 ± 125.2 |
Minimum | 20.9 ± 6.2 | 18.7 ± 6.7 | 22.6 ± 3.7 | 22.9 ± 3.1 | 353.1 ± 67.6 |
Values are presented as mean ± SD.
PA: pulmonary arteries; IVC: inferior vena cava.
Measuring points . | Diameter (mm/m2) . | Cross-sectional area (mm2/m2) . | |||
---|---|---|---|---|---|
Coronal . | Sagittal . | Axial . | |||
Breadth . | Length . | ||||
At the junction between IVC and tunnel | 23.8 ± 4.0 | 21.0 ± 3.2 | 26.1 ± 4.3 | 24.6 ± 5.1 | 400.7 ± 103.1 |
At the fenestration | 23.5 ± 5.1 | 21.4 ± 7.5 | 24.6 ± 4.9 | 29.6 ± 6.2 | 462.7 ± 115.1 |
At the junction between tunnel and PA | 26.7 ± 4.1 | 22.4 ± 5.5 | 25.5 ± 4.8 | 25.2 ± 3.8 | 423.7 ± 111.6 |
Maximum | 28.2 ± 4.0 | 26.2 ± 4.2 | 28.6 ± 4.6 | 31.1 ± 5.3 | 511.6 ± 125.2 |
Minimum | 20.9 ± 6.2 | 18.7 ± 6.7 | 22.6 ± 3.7 | 22.9 ± 3.1 | 353.1 ± 67.6 |
Measuring points . | Diameter (mm/m2) . | Cross-sectional area (mm2/m2) . | |||
---|---|---|---|---|---|
Coronal . | Sagittal . | Axial . | |||
Breadth . | Length . | ||||
At the junction between IVC and tunnel | 23.8 ± 4.0 | 21.0 ± 3.2 | 26.1 ± 4.3 | 24.6 ± 5.1 | 400.7 ± 103.1 |
At the fenestration | 23.5 ± 5.1 | 21.4 ± 7.5 | 24.6 ± 4.9 | 29.6 ± 6.2 | 462.7 ± 115.1 |
At the junction between tunnel and PA | 26.7 ± 4.1 | 22.4 ± 5.5 | 25.5 ± 4.8 | 25.2 ± 3.8 | 423.7 ± 111.6 |
Maximum | 28.2 ± 4.0 | 26.2 ± 4.2 | 28.6 ± 4.6 | 31.1 ± 5.3 | 511.6 ± 125.2 |
Minimum | 20.9 ± 6.2 | 18.7 ± 6.7 | 22.6 ± 3.7 | 22.9 ± 3.1 | 353.1 ± 67.6 |
Values are presented as mean ± SD.
PA: pulmonary arteries; IVC: inferior vena cava.
The comparison between patients with a tubular tunnel and patients with a non-tubular tunnel showed no differences regarding cross-sectional areas, percentage area of the tunnel compared with the area of the inferior vena cava and volume of the tunnel (Table 2). Furthermore, the degree of tunnel shape deviation and the change of the tunnel volume during the heart cycle were not significantly different between these groups (Figs 3 and 4). The tunnel volume correlated significantly with age (Fig. 5), body surface area (BSA) (r = 0.42; P = 0.005) and time after TCPC (r = 0.42; P = 0.001).
Comparison of patients with a tubular tunnel vs patients with a non-tubular tunnel
Parameter . | Tubular tunnel . | Non-tubular tunnel . | P-value . |
---|---|---|---|
Cross-sectional area of the tunnel (mm2/m2) | |||
At the junction between IVC and tunnel | 385.3 ± 60.3 | 418.8 ± 133.3 | 0.92 |
At the fenestration | 440.0 ± 99.2 | 494.9 ± 199.6 | 0.60 |
At the junction between tunnel and PA | 432.3 ± 116.8 | 425.8 ± 107.1 | 0.73 |
Percentage area at the fenestration compared with IVC area (%) | 113 ± 32 | 112 ± 41 | 0.53 |
Percentage area at the junction between tunnel and PA compared with IVC area (%) | 116 ± 26 | 125 ± 41 | 0.67 |
Volume of the tunnel (ml/m2) | 24.9 ± 9.5 | 26.1 ± 7.1 | 0.30 |
Mean blood flow (l/min/m2) | 1.0 ± 1.2 | 1.2 ± 0.4 | 0.05 |
Mean velocity (cm/s) | 5.6 ± 3.3 | 6.6 ± 2.6 | 0.60 |
Maximal velocity (cm/s) | 25.5 ± 10.1 | 35.1 ± 12.5 | 0.10 |
Parameter . | Tubular tunnel . | Non-tubular tunnel . | P-value . |
---|---|---|---|
Cross-sectional area of the tunnel (mm2/m2) | |||
At the junction between IVC and tunnel | 385.3 ± 60.3 | 418.8 ± 133.3 | 0.92 |
At the fenestration | 440.0 ± 99.2 | 494.9 ± 199.6 | 0.60 |
At the junction between tunnel and PA | 432.3 ± 116.8 | 425.8 ± 107.1 | 0.73 |
Percentage area at the fenestration compared with IVC area (%) | 113 ± 32 | 112 ± 41 | 0.53 |
Percentage area at the junction between tunnel and PA compared with IVC area (%) | 116 ± 26 | 125 ± 41 | 0.67 |
Volume of the tunnel (ml/m2) | 24.9 ± 9.5 | 26.1 ± 7.1 | 0.30 |
Mean blood flow (l/min/m2) | 1.0 ± 1.2 | 1.2 ± 0.4 | 0.05 |
Mean velocity (cm/s) | 5.6 ± 3.3 | 6.6 ± 2.6 | 0.60 |
Maximal velocity (cm/s) | 25.5 ± 10.1 | 35.1 ± 12.5 | 0.10 |
Values are presented as mean ± SD. P-values are from the Mann–Whitney U-test.
IVC: inferior vena cava; PA: pulmonary arteries.
Comparison of patients with a tubular tunnel vs patients with a non-tubular tunnel
Parameter . | Tubular tunnel . | Non-tubular tunnel . | P-value . |
---|---|---|---|
Cross-sectional area of the tunnel (mm2/m2) | |||
At the junction between IVC and tunnel | 385.3 ± 60.3 | 418.8 ± 133.3 | 0.92 |
At the fenestration | 440.0 ± 99.2 | 494.9 ± 199.6 | 0.60 |
At the junction between tunnel and PA | 432.3 ± 116.8 | 425.8 ± 107.1 | 0.73 |
Percentage area at the fenestration compared with IVC area (%) | 113 ± 32 | 112 ± 41 | 0.53 |
Percentage area at the junction between tunnel and PA compared with IVC area (%) | 116 ± 26 | 125 ± 41 | 0.67 |
Volume of the tunnel (ml/m2) | 24.9 ± 9.5 | 26.1 ± 7.1 | 0.30 |
Mean blood flow (l/min/m2) | 1.0 ± 1.2 | 1.2 ± 0.4 | 0.05 |
Mean velocity (cm/s) | 5.6 ± 3.3 | 6.6 ± 2.6 | 0.60 |
Maximal velocity (cm/s) | 25.5 ± 10.1 | 35.1 ± 12.5 | 0.10 |
Parameter . | Tubular tunnel . | Non-tubular tunnel . | P-value . |
---|---|---|---|
Cross-sectional area of the tunnel (mm2/m2) | |||
At the junction between IVC and tunnel | 385.3 ± 60.3 | 418.8 ± 133.3 | 0.92 |
At the fenestration | 440.0 ± 99.2 | 494.9 ± 199.6 | 0.60 |
At the junction between tunnel and PA | 432.3 ± 116.8 | 425.8 ± 107.1 | 0.73 |
Percentage area at the fenestration compared with IVC area (%) | 113 ± 32 | 112 ± 41 | 0.53 |
Percentage area at the junction between tunnel and PA compared with IVC area (%) | 116 ± 26 | 125 ± 41 | 0.67 |
Volume of the tunnel (ml/m2) | 24.9 ± 9.5 | 26.1 ± 7.1 | 0.30 |
Mean blood flow (l/min/m2) | 1.0 ± 1.2 | 1.2 ± 0.4 | 0.05 |
Mean velocity (cm/s) | 5.6 ± 3.3 | 6.6 ± 2.6 | 0.60 |
Maximal velocity (cm/s) | 25.5 ± 10.1 | 35.1 ± 12.5 | 0.10 |
Values are presented as mean ± SD. P-values are from the Mann–Whitney U-test.
IVC: inferior vena cava; PA: pulmonary arteries.
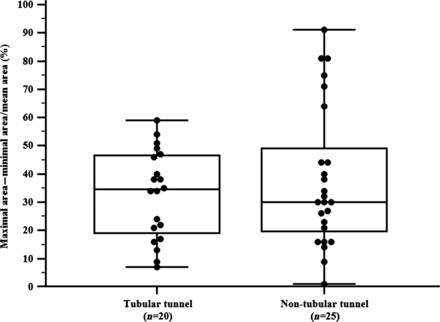
Box–Whisker–Plot showing the mean and dispersion (%) of the degree of area deviation according to the tunnel type.
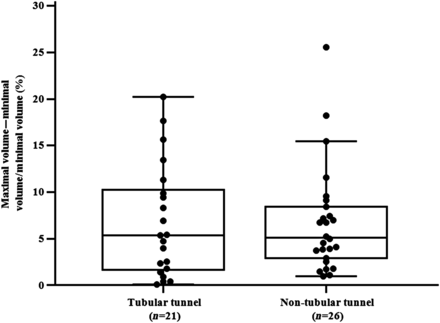
Box–Whisker–Plot showing the mean and dispersion (%) of the degree of volume change according to the tunnel type.

Correlation between age and normalized volume of the intra-atrial lateral tunnel.
Flow measurements
2D phase-contrast cine imaging
The mean blood flow in the tunnel correlated significantly with age (r = 0.73; P = 0.001; Fig. 6), BSA (r = 0.83; P < 0.0001) and RVSV (r = 0.55; P = 0.02). There was a trend towards a higher mean blood flow in patients with a non-tubular tunnel. No differences were found between patients with a tubular tunnel and patients with a non-tubular tunnel regarding the mean and maximal flow velocity in the tunnel (Table 2).

Flow-sensitive 3D phase-contrast cine imaging
Four patients had a tubular and 2 patients a non-tubular tunnel. Visual analyses showed retrograde flow at the junction between the inferior vena cava and the intra-atrial tunnel in 1 patient with a tubular tunnel, and this finding was confirmed by 2D phase-contrast imaging. Additionally, the tunnel blood flow below the fenestration was disordered (e.g. swirling), whereas in the upper part of the tunnel (above the fenestration) the blood flow was more streamlined. Major flow disturbances caused by a device used for the closure of the fenestration were not present.
DISCUSSION
In the present study, shape deviations of the intra-atrial lateral tunnel from a tubular shape were observed in 54% of patients. However, these morphological changes do not lead to significant alterations in tunnel blood flow and dimensions. This study further demonstrated that the volume and the mean tunnel blood flow correlated with age and BSA, suggesting adequate growth of the tunnel.
Anatomy and dimensions of the intra-atrial lateral tunnel
To our knowledge, this is the first study that systematically examined the shape and volumes of the intra-atrial lateral tunnel in a large series of patients with a functional single ventricle.
Although shape deviations of the tunnel in our series were present in 54% of the evaluated patients, no significant differences regarding tunnel dimensions and volumes between patients with a tubular tunnel and patients with alterations of tunnel shape were found. This suggests that the observed morphological changes in our group of HLHS patients were only moderate. None of the evaluated patients needed reoperation for tunnel modification.
In this MRI study, we determined the increase of tunnel volume with both age and BSA as a surrogate parameter of growth. In contrast, previous studies using angiocardiography and echocardiography determined only the maximal diameter of the tunnel and calculated the respective area [7, 12]. However, volume seems to be a more reliable parameter when investigating this question. In addition, accuracy of MRI is obviously superior to that of echo when examining tunnel shape and the direct measurement of areas avoids the assumption of a circular shape of the tunnel.
In contrast to the intra-atrial lateral tunnel, the increasingly preferred extracardiac conduit has no growth potential [13], so that particularly during growth the intra-atrial lateral tunnel can be advantageous. The size of the extracardiac tunnel do not perfectly fit to patient size during growth as at the time of surgery an oversized conduit is used to have appropriate tunnel dimensions in adult life. Furthermore, our measurements suggest that the volumes of the intra-atrial lateral tunnel change dynamically during the cardiac cycle, which may be haemodynamically beneficial.
Flow measurements
Our blood flow measurements in the tunnel (in patients with closed fenestration) demonstrate that at the mid-term follow-up the observed alterations from a tubular tunnel shape have no negative impact on the mean and maximal blood flow velocities in the tunnel. Surprisingly, there was a trend towards a higher mean blood flow rate in patients with a non-tubular tunnel compared with patients with a tubular tunnel. As we observed an increase in the indexed mean blood flow rate in the tunnel with age, the slightly older age of patients with a non-tubular tunnel in the group of patients who underwent 2D phase-contrast imaging (6.3 ± 3.4 vs 4.1 ± 2.0 years, P = 0.16) may be the most likely explanation. To our knowledge, there is no other study that evaluated the influence of tunnel shape on blood flow and flow velocities. The increase in mean blood flow with both age and BSA is compatible with data from the literature [14].
Additionally, in a subgroup of patients we performed 3D phase-contrast-flow measurements. Visual analyses showed a disordered blood flow (swirling and reflux) at the junction between the inferior vena cava and the intra-atrial lateral tunnel. These observations were in agreement with the findings of Sharma et al. [15]. They also found a disordered blood flow in the lower part of the tunnel in patients with an intra-atrial lateral tunnel and an extracardiac conduit. In contrast, Fogel et al. [16] have no indications for disturbances of blood flow through the tunnel in patients after TCPC.
The observed blood flow phenomena (swirling and reflux) might be produced by several factors such as respiration, the cardiac cycle and pulmonary vascular resistance, which were not analysed in the present study. Major flow disturbances caused by the device used for the closure of the fenestration were not present. As a prospect for future studies, flow-sensitive 3D phase-contrast CMR may be an accurate tool to analyse venous flow patterns systematically. This technique may give more information on fluid dynamics and potential energy loss in different types of TCPC.
Study limitations
With CMR it was possible to assess anatomy, dimensions and blood flow of the intra-atrial lateral tunnel in the majority of patients. However, a small group of patients could not be enrolled in the study, since CMR imaging was impaired by metal artefacts from implanted devices. Growth of the intra-atrial lateral tunnel is not shown by longitudinal measurements because of the cross-sectional design of our study.
CONCLUSIONS
In the mid-term follow-up a large group of patients after completion of the TCPC develop mild deviations of the intra-atrial lateral tunnel from a tubular shape. These shape variations have no impact on the maximum or mean blood flow within the tunnel. Blood flow and tunnel volume increases with age and BSA, suggesting that the conduit capacity of the tunnel adjusts to body growth. Further studies are needed to monitor the long-term follow-up.
Funding
This study was supported by the Deutsche Gesellschaft für Pädiatrische Kardiologie, Germany (www.kinderkardiologie.org).
Conflict of interest: none declared.
ACKNOWLEDGEMENTS
We thank Traudel Hansen (CMR technologist) for her assistance in patient and study management.
REFERENCES
Author notes
Presented at the 46th Annual Meeting of the Association of European Pediatric Cardiology, Istanbul, Turkey, 25 May 2012.