-
PDF
- Split View
-
Views
-
Cite
Cite
Rouven Kubicki, Jochen Grohmann, Matthias Siepe, Christoph Benk, Frank Humburger, Anne Rensing-Ehl, Brigitte Stiller, Early prediction of capillary leak syndrome in infants after cardiopulmonary bypass, European Journal of Cardio-Thoracic Surgery, Volume 44, Issue 2, August 2013, Pages 275–281, https://doi.org/10.1093/ejcts/ezt028
- Share Icon Share
Abstract
As an inflammatory reaction after cardiac surgery involving cardiopulmonary bypass (CPB), capillary leak syndrome (CLS) is associated with increased morbidity, especially in newborns and infants. We investigated whether different cytokines measured via microdialysis can monitor local inflammation in adipose tissue subcutaneously and predict the development of CLS early, before clinical signs appear. Furthermore, we investigated whether there are age-related differences between the inflammatory responses in newborns and infants.
We performed a prospective study taking serial measurements of the inflammatory response detected in subcutaneous adipose tissue up to 24 h postoperatively. The cohort consisted of 23 neonates and infants (median age 155, range 6–352 days; median body weight 5.4 kg, range 2.6–9.2 kg) who underwent congenital heart surgery with CPB. Microdialysis catheters were introduced in one lateral thigh subcutaneously using a velocity of 1.0 µl/min. Serial microdialysis analyses for cytokines (interleukin [IL]-6, IL-8, IL-10) and complement activation (C3a) were performed. CLS was quantified by X-ray subcutaneous-thoracic ratios.
The median bypass time was 150 min (range 42–432 min) and the aortic cross-clamp time 76 min (range 0–188 min). Six out of 23 infants developed postoperative CLS. Younger age (P = 0.02) and longer bypass time (r = 0.48; P = 0.021) correlated strongly with the development of CLS. Pro- and anti-inflammatory cytokines and complement activation were detected subcutaneously in all patients. The highest levels of IL-6 (55.0 pg/ml) and IL-8 (65.9 pg/ml) were detected 2 h after CPB. During surgery, the C3a level rose dramatically (167.1 ng/ml), followed by a release of IL-10 at the end of CPB. Patients with CLS produced a characteristic and significant second peak of C3a at 8 h postoperatively (CLS 63.8 ng/ml vs non-CLS 23.5 ng/ml; P < 0.01). We detected an aged-related difference in the release of IL-6 and C3a. Longer intubation time (r = 0.63; P = 0.001), higher inotropic demand (r = 0.67; P = 0.001) and higher serological lactate levels (r = 0.65; P = 0.001) correlated closely with the development of CLS.
Diagnostic microdialysis can detect local inflammation and may predict the development of CLS early before severe clinical signs appear.
INTRODUCTION
The prevalence of congenital heart disease (CHD) in Germany is ∼1.1% [1]. Despite all the impressive improvements within recent decades, congenital heart surgery with cardiopulmonary bypass (CPB) in the first year of life is associated with higher mortality and morbidity than in older children [2–4]. Age-dependent differences in the inflammatory response, the greater susceptibility to injury of immature organ systems and the larger extracorporeal circuit to patient size ratio make younger, smaller patients more vulnerable to the damaging effects of CPB [5]. The major problem associated with CPB in newborns and infants is prolonged inflammation leading to capillary leak syndrome (CLS) and organ dysfunction, resulting in higher morbidity and mortality [2, 4, 6, 7].
The inflammatory response is a complex biological and biochemical process involving cellular processes, the contact system, the complement system and cytokines. The latter seem to play a key role in the CPB-mediated inflammatory cascade. Previous studies investigating the inflammatory profile in plasma and bronchoalveolar lavage showed correlations between cytokines and CPB. Elevated levels of tumour necrosis factor-α (TNF-α), and interleukin 6 (IL-6) and IL-8 have been shown to be associated with poorer outcomes after cardiac surgery [8, 9]. Anti-inflammatory cytokines like IL-10 seems to contribute to immune depression by inhibiting the release of pro-inflammatory cytokines [10]. The balance between pro- and anti-inflammatory cytokines is important in determining the level of the inflammatory response. CPB in combination with anaesthesia, medication, vascular injury and surgical stress affects the extent of inflammatory response [4, 10, 11].
Many attempts have been made to mitigate the overwhelming inflammatory response triggered by CPB in paediatrics. The development of modified CPB components and further technical strategies have reduced postoperative morbidity and increased survival rates. To anticipate and prevent CLS, we believe it will soon become necessary to distinguish postoperative complications prior to the appearance of clinical signs.
Microdialysis has recently become established as a widely accepted method to assess tissue metabolism. Numerous studies investigating the pathophysiology of cardiac surgery-related acute phase reactions have analysed blood samples. Microdialysis represents a promising alternative to explore the mediator mechanism of pathophysiological tissue events. CLS is characterized by increased capillary permeability causing fluid and proteins to shift from the intravascular to interstitial space.
The aim of this feasibility study was to subcutaneously monitor local inflammation in adipose tissue to determine the profile of cytokines involved in the inflammatory response to cardiac surgery entailing CPB in newborns and small infants. Using this method, we aim for the early prediction of CLS development, before severe clinical signs appear. We also hoped to ascertain age-dependent differences in complement and cytokine activation.
METHODS
Patients
This prospective single-centre study (February 2009–October 2009) included 23 children within the first year of life (9 males, 14 females), median age 155 (range 6–352) days, median body weight 5.4 (range 2.6–9.2) kg, who underwent cardiac surgery with CPB. Children receiving antibiotics or anti-inflammatory medication or with infection prior to surgery were excluded. Clinical and serum parameters were obtained beginning 1 day before surgery until 24 h post-CPB. All infants were allocated to one of two groups (newborn [n = 6] vs infants [n = 17]). Extracardiac anomalies were Trisomy 21 (n = 4), DiGeorge syndrome (n = 2), Pierre Robin sequence (n = 1), cleft lip and palate (n = 1), oesophageal atresia Type Ib (n = 1) and prothrombin G20210 (n = 1). The patients' diagnoses and their surgical procedures are illustrated in Table 1.
Underlying diagnosis in the patients with congenital heart disease and their surgical procedures
Cardiac defect . | Age (days) . | Procedure . |
---|---|---|
AVSD | 184 127 | Corrective surgery |
AVSD | 265 | BDG |
AVSD + PDA | 227 | Corrective surgery |
ASD + VSD | 164 | Corrective surgery |
VSD | 176 187 | Corrective surgery |
TAPVD + ASD | 7 | Corrective surgery |
PAPVD + ASD | 20 | Corrective surgery |
SV + TAPVD | 261 | BDG |
TOF | 163 177 182 | Corrective surgery |
DORV | 63 289 | Corrective surgery |
DORV + RVOT-stent | 280 | Corrective surgery |
DILV | 159 | BDG |
IAA + VSD + LVOTO | 237 | Corrective surgery |
TAC + IAA | 17 | Corrective surgery (+ECMO) |
TA | 21 | Stage-1 palliation (Norwood I) |
HLHS | 17 | Stage-1 palliation (Norwood I) |
D-TGA + ASD | 6 | ASO |
IAA + VSD + LVOTO | 352 | BDG (+ECMO) |
Cardiac defect . | Age (days) . | Procedure . |
---|---|---|
AVSD | 184 127 | Corrective surgery |
AVSD | 265 | BDG |
AVSD + PDA | 227 | Corrective surgery |
ASD + VSD | 164 | Corrective surgery |
VSD | 176 187 | Corrective surgery |
TAPVD + ASD | 7 | Corrective surgery |
PAPVD + ASD | 20 | Corrective surgery |
SV + TAPVD | 261 | BDG |
TOF | 163 177 182 | Corrective surgery |
DORV | 63 289 | Corrective surgery |
DORV + RVOT-stent | 280 | Corrective surgery |
DILV | 159 | BDG |
IAA + VSD + LVOTO | 237 | Corrective surgery |
TAC + IAA | 17 | Corrective surgery (+ECMO) |
TA | 21 | Stage-1 palliation (Norwood I) |
HLHS | 17 | Stage-1 palliation (Norwood I) |
D-TGA + ASD | 6 | ASO |
IAA + VSD + LVOTO | 352 | BDG (+ECMO) |
ASD: atrial septal defect; ASO: arterial switch operation; AVSD: atrioventricular septal defect; BDG: bidirectional superior cavopulmonary anastomosis (Glenn); DILV: double inlet left ventricle; DORV: double outlet right ventricle; HLHS: hypoplastic left heart syndrome; ECMO: extracorporeal membrane oxygenation; IAA: interruption of aortic arch; LVOTO: left ventricular outflow tract obstruction; PAPVD: partial anomalous pulmonary venous drainage; PDA: patent ductus arteriosus; RVOT-stent: right ventricular outflow tract stent; Stage-1 palliation: modified Norwood I/Damus-Kaye-Stansel; SV: single ventricle; TA: tricuspid atresia; TAC: truncus arteriosus communis; TAPVD: total anomalous pulmonary venous drainage; TGA: transposition of the great arteries; TOF: tetralogy of Fallot; VSD: ventricle septal defect.
Underlying diagnosis in the patients with congenital heart disease and their surgical procedures
Cardiac defect . | Age (days) . | Procedure . |
---|---|---|
AVSD | 184 127 | Corrective surgery |
AVSD | 265 | BDG |
AVSD + PDA | 227 | Corrective surgery |
ASD + VSD | 164 | Corrective surgery |
VSD | 176 187 | Corrective surgery |
TAPVD + ASD | 7 | Corrective surgery |
PAPVD + ASD | 20 | Corrective surgery |
SV + TAPVD | 261 | BDG |
TOF | 163 177 182 | Corrective surgery |
DORV | 63 289 | Corrective surgery |
DORV + RVOT-stent | 280 | Corrective surgery |
DILV | 159 | BDG |
IAA + VSD + LVOTO | 237 | Corrective surgery |
TAC + IAA | 17 | Corrective surgery (+ECMO) |
TA | 21 | Stage-1 palliation (Norwood I) |
HLHS | 17 | Stage-1 palliation (Norwood I) |
D-TGA + ASD | 6 | ASO |
IAA + VSD + LVOTO | 352 | BDG (+ECMO) |
Cardiac defect . | Age (days) . | Procedure . |
---|---|---|
AVSD | 184 127 | Corrective surgery |
AVSD | 265 | BDG |
AVSD + PDA | 227 | Corrective surgery |
ASD + VSD | 164 | Corrective surgery |
VSD | 176 187 | Corrective surgery |
TAPVD + ASD | 7 | Corrective surgery |
PAPVD + ASD | 20 | Corrective surgery |
SV + TAPVD | 261 | BDG |
TOF | 163 177 182 | Corrective surgery |
DORV | 63 289 | Corrective surgery |
DORV + RVOT-stent | 280 | Corrective surgery |
DILV | 159 | BDG |
IAA + VSD + LVOTO | 237 | Corrective surgery |
TAC + IAA | 17 | Corrective surgery (+ECMO) |
TA | 21 | Stage-1 palliation (Norwood I) |
HLHS | 17 | Stage-1 palliation (Norwood I) |
D-TGA + ASD | 6 | ASO |
IAA + VSD + LVOTO | 352 | BDG (+ECMO) |
ASD: atrial septal defect; ASO: arterial switch operation; AVSD: atrioventricular septal defect; BDG: bidirectional superior cavopulmonary anastomosis (Glenn); DILV: double inlet left ventricle; DORV: double outlet right ventricle; HLHS: hypoplastic left heart syndrome; ECMO: extracorporeal membrane oxygenation; IAA: interruption of aortic arch; LVOTO: left ventricular outflow tract obstruction; PAPVD: partial anomalous pulmonary venous drainage; PDA: patent ductus arteriosus; RVOT-stent: right ventricular outflow tract stent; Stage-1 palliation: modified Norwood I/Damus-Kaye-Stansel; SV: single ventricle; TA: tricuspid atresia; TAC: truncus arteriosus communis; TAPVD: total anomalous pulmonary venous drainage; TGA: transposition of the great arteries; TOF: tetralogy of Fallot; VSD: ventricle septal defect.
One infant was intubated and treated with Milrinon 1 day before surgery due to congestive heart failure. Three patients were treated preoperatively with beta-blockers, 5 received diuretics and 4 prostaglandin E1.
All children survived to discharge home; only one child died on extracorporeal membrane oxygenation 18 days after corrective surgery for truncus arteriosus communis Type 4b with an interrupted aortic arch and unsuccessful weaning from CPB.
Sternal closure was delayed in 8 of 23 patients and was performed between the second and the sixth postoperative days.
Capillary leak syndrome
We defined CLS as the development of either non-cardiac oedema or ascites or pleural effusion and a rise in the subcutaneous-thoracic ratio (S/T) (threshold of >12.6%). None of our patients fulfilled these criteria before the surgical intervention. The S/T was calculated from a chest X-ray like that described by Sonntag et al. [12] on admission on intensive care unit (ICU), after 24 and 48 h.
Anaesthetic technique
All patients underwent balanced general anaesthesia consisting of induction with intravenous rohypnol, fentanyl and pancuronium bromide. Anaesthesia was maintained with inhaled isofluran plus intravenous fentanyl and midazolam. All had a urinary bladder catheter, central venous catheter, nasogastric tube and a radial or femoral arterial-line inserted.
Cardiopulmonary bypass techniques
CPB was performed using a modified children’s device (Stöckert S III, Sorin Group, Mirandola, Italy) that includes the following components: oxygenator and attached hard shell reservoir (D100/ D101 Dideco, Sorin Group, Munic, Germany), roller pumps (Sorin Group, Mirandola, Italy), an arterial filter (D131/130, Sorin Group, Munic, Germany), a cardioplegia heat exchanger (CSC 14, Sorin Group, Mirandola, Italy), a haemofilter (Dideco Hft 0.2, Sorin Group, Mirandola, Munic) and a phosphorylcholin-coated tubing system.
The extracorporeal circuit was primed with FFP, mannitole 20%, heparin, sodium bicarbonate 8.4%, packed red blood cells and ionosterile. The total priming volume was aimed at 250–350 ml. After clamping the aorta, 30 ml/kg blood cardioplegia according to Buckberg [13] was administered and repeated every 20 min during CPB (10 ml/kg). For continuous online blood gas analysis, we used CDI™ (Terumo Corporation, Tokyo, Japan). To optimize the venous blood return, we applied a vacuum-assisted venous drainage (VAVD)-controller (Maquet, Rastatt, Germany). If modest or deep hypothermia was necessary, the INVOS® System (In Vivo Optical Spectroscopy; Somanetics Corporation, Troy, Michigan) was used to measure changes in oxygen levels to detect the risk of brain hypoxia. Routine heparinization was reversed by an equivalent amount of protamine sulphate. At the time of weaning from CPB, inotropic and vasopressor support was instituted as needed. Intra- and postoperative data are given in Tables 2 and 3.
Dataa . | Patients (n = 23) . | Range . |
---|---|---|
Duration of CPB (min) | 150 ± 20 | (42–432) |
Duration of aortic-clamping time (min) | 76 ± 12 | (0–188) |
Lowest temperature during CPB (°C) | 31 ± 1 | (17.8–36.3) |
Flow rate of CPB (l/min/m2 KOF) | 0.93 ± 0.04 | (0.58–1.27) |
Duration of mechanical ventilation (h) | 185 ± 71 | (3–1552) |
12-h fluid balance (ml) | 13 ± 40 | (−428 to +324) |
CLS (yes/no) | 6/17 |
Dataa . | Patients (n = 23) . | Range . |
---|---|---|
Duration of CPB (min) | 150 ± 20 | (42–432) |
Duration of aortic-clamping time (min) | 76 ± 12 | (0–188) |
Lowest temperature during CPB (°C) | 31 ± 1 | (17.8–36.3) |
Flow rate of CPB (l/min/m2 KOF) | 0.93 ± 0.04 | (0.58–1.27) |
Duration of mechanical ventilation (h) | 185 ± 71 | (3–1552) |
12-h fluid balance (ml) | 13 ± 40 | (−428 to +324) |
CLS (yes/no) | 6/17 |
aValues as number (n) or mean ± standard error of the mean, range of mean (−).
Dataa . | Patients (n = 23) . | Range . |
---|---|---|
Duration of CPB (min) | 150 ± 20 | (42–432) |
Duration of aortic-clamping time (min) | 76 ± 12 | (0–188) |
Lowest temperature during CPB (°C) | 31 ± 1 | (17.8–36.3) |
Flow rate of CPB (l/min/m2 KOF) | 0.93 ± 0.04 | (0.58–1.27) |
Duration of mechanical ventilation (h) | 185 ± 71 | (3–1552) |
12-h fluid balance (ml) | 13 ± 40 | (−428 to +324) |
CLS (yes/no) | 6/17 |
Dataa . | Patients (n = 23) . | Range . |
---|---|---|
Duration of CPB (min) | 150 ± 20 | (42–432) |
Duration of aortic-clamping time (min) | 76 ± 12 | (0–188) |
Lowest temperature during CPB (°C) | 31 ± 1 | (17.8–36.3) |
Flow rate of CPB (l/min/m2 KOF) | 0.93 ± 0.04 | (0.58–1.27) |
Duration of mechanical ventilation (h) | 185 ± 71 | (3–1552) |
12-h fluid balance (ml) | 13 ± 40 | (−428 to +324) |
CLS (yes/no) | 6/17 |
aValues as number (n) or mean ± standard error of the mean, range of mean (−).
Variablesa . | Non-CLS (n = 17) . | CLS (n = 6) . | P-value . | |
---|---|---|---|---|
Age (days) | 182 [88] | 17 [97] | 0.019 | |
Weight (kg) | 6.3 [1.8] | 3.3 [1.5] | 0.001 | |
Duration of mechanical ventilation (h) | 26.2 [77] | 291.3 [716] | 0.002 | |
Duration of CPB (min) | 109 [106] | 194 [181] | 0.024 | |
Duration of aortic-clamping time (min) | 61 [61] | 113.5 [125] | 0.233 | ns |
Lowest temperature during CPB (°C) | 34.8 [3.8] | 27.0 [11.3] | 0.003 | |
Flow rate of CPB (l/min/m2 KOF) | 1.1 [0.2] | 0.6 [0.29] | 0.001 | |
Lactate (mmol/l) | ||||
Preoperative | 0.8 [0.6] | 1.4 [1.3] | 0.038 | |
8-h postoperative | 1.1 [0.8] | 3.0 [1.7] | 0.003 | |
24-h postoperative | 1.3 [0.7] | 3.1 [1.6] | 0.001 | |
Leucocytes (G/l) | ||||
preoperative | 9.7 [3.8] | 12.5 [7.4] | 0.050 | |
8-h postoperative | 9.0 [4.9] | 6.6 [6.9] | 0.050 | |
Fluid balance 12 h after surgery (ml) | (−53) [294] | 124 [142] | 0.036 | |
Inotropic value | ||||
End of CPB | 12.5 [11] | 28.5 [19] | 0.015 | |
8-h postoperative | 7.6 [8] | 14.3 [14] | 0.088 | ns |
24-h postoperative | 7.4 [4.8] | 12.6 [14.6] | 0.194 | ns |
Variablesa . | Non-CLS (n = 17) . | CLS (n = 6) . | P-value . | |
---|---|---|---|---|
Age (days) | 182 [88] | 17 [97] | 0.019 | |
Weight (kg) | 6.3 [1.8] | 3.3 [1.5] | 0.001 | |
Duration of mechanical ventilation (h) | 26.2 [77] | 291.3 [716] | 0.002 | |
Duration of CPB (min) | 109 [106] | 194 [181] | 0.024 | |
Duration of aortic-clamping time (min) | 61 [61] | 113.5 [125] | 0.233 | ns |
Lowest temperature during CPB (°C) | 34.8 [3.8] | 27.0 [11.3] | 0.003 | |
Flow rate of CPB (l/min/m2 KOF) | 1.1 [0.2] | 0.6 [0.29] | 0.001 | |
Lactate (mmol/l) | ||||
Preoperative | 0.8 [0.6] | 1.4 [1.3] | 0.038 | |
8-h postoperative | 1.1 [0.8] | 3.0 [1.7] | 0.003 | |
24-h postoperative | 1.3 [0.7] | 3.1 [1.6] | 0.001 | |
Leucocytes (G/l) | ||||
preoperative | 9.7 [3.8] | 12.5 [7.4] | 0.050 | |
8-h postoperative | 9.0 [4.9] | 6.6 [6.9] | 0.050 | |
Fluid balance 12 h after surgery (ml) | (−53) [294] | 124 [142] | 0.036 | |
Inotropic value | ||||
End of CPB | 12.5 [11] | 28.5 [19] | 0.015 | |
8-h postoperative | 7.6 [8] | 14.3 [14] | 0.088 | ns |
24-h postoperative | 7.4 [4.8] | 12.6 [14.6] | 0.194 | ns |
aValues as number (n) or median [inter-quartile range].
ns: non-significant.
Variablesa . | Non-CLS (n = 17) . | CLS (n = 6) . | P-value . | |
---|---|---|---|---|
Age (days) | 182 [88] | 17 [97] | 0.019 | |
Weight (kg) | 6.3 [1.8] | 3.3 [1.5] | 0.001 | |
Duration of mechanical ventilation (h) | 26.2 [77] | 291.3 [716] | 0.002 | |
Duration of CPB (min) | 109 [106] | 194 [181] | 0.024 | |
Duration of aortic-clamping time (min) | 61 [61] | 113.5 [125] | 0.233 | ns |
Lowest temperature during CPB (°C) | 34.8 [3.8] | 27.0 [11.3] | 0.003 | |
Flow rate of CPB (l/min/m2 KOF) | 1.1 [0.2] | 0.6 [0.29] | 0.001 | |
Lactate (mmol/l) | ||||
Preoperative | 0.8 [0.6] | 1.4 [1.3] | 0.038 | |
8-h postoperative | 1.1 [0.8] | 3.0 [1.7] | 0.003 | |
24-h postoperative | 1.3 [0.7] | 3.1 [1.6] | 0.001 | |
Leucocytes (G/l) | ||||
preoperative | 9.7 [3.8] | 12.5 [7.4] | 0.050 | |
8-h postoperative | 9.0 [4.9] | 6.6 [6.9] | 0.050 | |
Fluid balance 12 h after surgery (ml) | (−53) [294] | 124 [142] | 0.036 | |
Inotropic value | ||||
End of CPB | 12.5 [11] | 28.5 [19] | 0.015 | |
8-h postoperative | 7.6 [8] | 14.3 [14] | 0.088 | ns |
24-h postoperative | 7.4 [4.8] | 12.6 [14.6] | 0.194 | ns |
Variablesa . | Non-CLS (n = 17) . | CLS (n = 6) . | P-value . | |
---|---|---|---|---|
Age (days) | 182 [88] | 17 [97] | 0.019 | |
Weight (kg) | 6.3 [1.8] | 3.3 [1.5] | 0.001 | |
Duration of mechanical ventilation (h) | 26.2 [77] | 291.3 [716] | 0.002 | |
Duration of CPB (min) | 109 [106] | 194 [181] | 0.024 | |
Duration of aortic-clamping time (min) | 61 [61] | 113.5 [125] | 0.233 | ns |
Lowest temperature during CPB (°C) | 34.8 [3.8] | 27.0 [11.3] | 0.003 | |
Flow rate of CPB (l/min/m2 KOF) | 1.1 [0.2] | 0.6 [0.29] | 0.001 | |
Lactate (mmol/l) | ||||
Preoperative | 0.8 [0.6] | 1.4 [1.3] | 0.038 | |
8-h postoperative | 1.1 [0.8] | 3.0 [1.7] | 0.003 | |
24-h postoperative | 1.3 [0.7] | 3.1 [1.6] | 0.001 | |
Leucocytes (G/l) | ||||
preoperative | 9.7 [3.8] | 12.5 [7.4] | 0.050 | |
8-h postoperative | 9.0 [4.9] | 6.6 [6.9] | 0.050 | |
Fluid balance 12 h after surgery (ml) | (−53) [294] | 124 [142] | 0.036 | |
Inotropic value | ||||
End of CPB | 12.5 [11] | 28.5 [19] | 0.015 | |
8-h postoperative | 7.6 [8] | 14.3 [14] | 0.088 | ns |
24-h postoperative | 7.4 [4.8] | 12.6 [14.6] | 0.194 | ns |
aValues as number (n) or median [inter-quartile range].
ns: non-significant.
CMA microdialysis system (Stockholm, Sweden)
In our study we appropriated a CMA 71 high cut-off brain microdialysis catheter (cut-off 100 kDa, membrane length 10 mm). After anaesthesia, we inserted subcutaneously a microdialysis catheter in one lateral thigh using an intravenous needle (Vasofix® Braunüle G18, B. Braun Melsungen AG, Melsungen, Germany). We carved the outer plastic cylinder 2.5 cm proximal to the needle’s tip to mobilize it. We introduced the intravenous needle and withdrew the metal cannula. After careful observation to exclude any bleeding from the insertion, the microdialysis catheter was inserted in the plastic cylinder that was subsequently withdrawn, leaving the microdialysis catheter in the subcutaneous tissue. Immediately after insertion, the infusion was started by connecting the CMA 107 microinjection pump (Microdialysis, Stockholm, Sweden) at 1.0 µl/min. The microdialysis syringes were filled with perfusion fluid, applied to the pump and connected to the catheter. We perfused the catheter with Voluven (osmolality of 308 mosmol/l) to prevent ultrafiltration of the membrane. The outlet tube was extended to a holder for a microvial that collects the dialysis sample. The dialysates were stored in a freezer at −20°C for subsequent analysis of inflammatory markers.
Microdialysis samples
We employed a 120-min sampling interval for our dialysis. Microvials were changed at seven time points as follows: (S0) after induction of anaesthesia, (H0) directly after CPB, (H1) 2 h, (H2) 4 h, (H3) 6 h and (H4) 8 h after CPB. The final measurement (H5) was taken 24 h after the CPB procedure.
Analysis of cytokines and anaphylatoxin
All cytokines were analysed using cytometric bead array (CBA). The Human Inflammatory Cytokines Kit was used (BD™ Biosciences, San Jose, CA) to quantify the cytokines IL-6, IL-8, IL-10, IL-1β, IL-12p70 and TNF-α according to the manufacturer's instructions.
Anaphylatoxin C3a was measured by using double antibody sandwich enzyme-linked immunosorbent assay (ELISA). We used Human C3a ELISA Kit (BD OptEIA™, BD Biosciences, San Jose, CA) according to the manufacturer’s instructions.
Statistics
Data were analysed using SPSS 17.0 (SPSS, Inc., Washington, DC). Data are shown as means (±SD) and median (range). We used the non-parametric Mann–Whitney U-test and Wilcoxon signed-rank test to assess statistical significance. Strength of correlation between cytokine levels and clinical parameters was assessed using the Spearman correlation test. Probability values <0.05 were considered statistically significant. All results are shown as box-and-whiskers plots.
RESULTS
The median bypass time was 150 (range 42–432) min and the aortic cross-clamp was 76 (range 0–188) min. Younger age (P = 0.02) and longer bypass time (r = 0.48; P = 0.021) correlated strongly with the development of CLS.
Cytokine and complement activation
Pro- and anti-inflammatory cytokines and complement activation were subcutaneously measurable in all patients (Fig. 1A–C). Two hours after the end of CPB, the median IL-6 concentration rose to a maximum (20 vs 55 pg/ml) and decreased continuously to baseline values in the following hours. We only detected IL-8 in 14 patients during surgery. Two hours after CPB, we noted a significant increase (10 vs 66 pg/ml) in IL-8 concentrations, which remained high on the first postoperative day (60 pg/ml). IL-10 concentrations rose immediately after CPB in 9 patients—up to 5 pg/ml. IL-12p70, IL-1β and TNF-α revealed no significant changes over time. We detected a high release of C3a—up to 167 ng/ml—during surgery. In the first 2 h after CPB, we observed a rapid drop to 11 ng/ml on the first postoperative day.
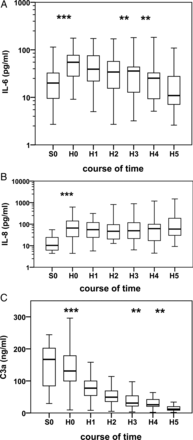
(A–C) Box–whisker plot illustrating median, quartiles and ranges at seven time points as follows: (S0) after induction of anaesthesia; (H0) directly after CPB; (H1) 2–4 h, (H2) 4–6 h, (H3) 6–8 h and (H4) 8–10 h after CPB and (H5) 24 h after CPB. Wilcoxon signed-rank test. (A) IL-6, ***P < 0.001, **P < 0.01. (B) IL-8, ***P < 0.001. (C) C3a, ***P < 0.001, **P < 0.01.
Subcutaneous-thoracic ratio
We demonstrated a significant increase in S/T after surgery in all patients in comparison with baseline (7.4 vs 9.4%). The majority of our patients, especially the younger ones, developed mild pleural effusion or minor ascites within the first postoperative hours without clinical restriction. Later, 6 of 23 children (5 of whom were newborns) developed CLS according to the defined criteria. In comparison with infants, the newborns presented significantly higher S/T values on postoperative days 1 and 2 (Fig. 2).
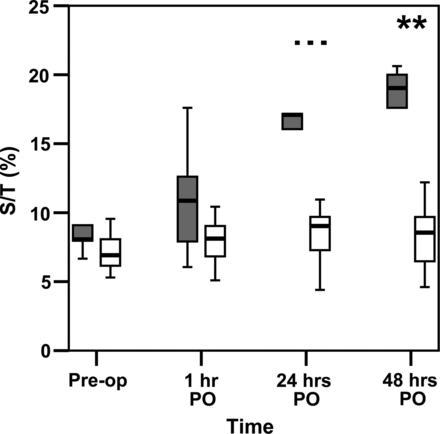
Comparison of S/T-ratios between neonates (black box) and infants (white box) before and after surgery. ***P < 0.001, **P < 0.01, Mann–Whitney U-test.
Correlation between cytokines and complement factors and clinical variables
We noted an age-related difference in the release of IL-6 and C3a. Longer intubation time (r = 0.63; P = 0.001), greater inotrope demand (r = 0.67; P = 0.001) and higher serological lactate levels (r = 0.65; P = 0.001) correlated strongly with CLS. Patients who developed CLS showed lower IL-6 levels 2 h after CPB (r = −0.52, P = 0.01). Moreover, low IL-6 concentrations correlated strongly with high S/T values. We also detected a positive correlation between S/T and C3a. Patients with CLS demonstrated a significant second peak of complement 3a (CLS 64 vs 24 ng/ml; P < 0.01) during the study (Fig. 3) compared with patients without CLS. The release of IL-10 depends on age. We identified no correlations regarding IL-8 concentrations.
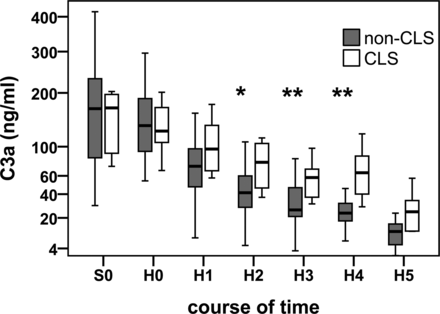
Changes in subcutaneous C3a levels between patients with CLS (white box) and without CLS (black box) during and after CPB. **P < 0.01, *P < 0.05, Mann–Whitney U-test.
DISCUSSION
In this study, we discovered that diagnostic microdialysis enables us to diagnose the inflammatory response in adipose tissue—the target area where CLS pathophysiology takes place. Our results illustrate the inflammatory mediator profile in neonates and infants undergoing heart surgery with CPB. Furthermore, diagnostic microdialysis seems to suggest that CLS may be predicted before its severe clinical signs appear.
Effect of cardiopulmonary bypass on cytokines and the complement system
This study demonstrates that both newborns and infants exhibit a local inflammatory response in adipose tissue after CPB. We confirm the pro-inflammatory response to paediatric CPB with rising levels of IL-6, IL-8 and the S/T. Previous studies showed the essential involvement of IL-6 and IL-8 in acute inflammation of diverse organs. IL-6 is usually undetectable in the peripheral blood of healthy children. IL-6 and IL-8 plasma levels typically increase during and after heart surgery involving CPB [14]. IL-6 is considered a good predictor of a complicated clinical course and clinical outcomes. Patients showing a significant increase in IL-6 during or early after CPB had higher mortality [15]. Longer bypass time, higher serological lactate levels, a greater need for inotropic support and longer postoperative mechanical ventilation were associated with lower interstitial IL-6 concentrations, probably caused by the diluting effects of CLS in adipose tissue. In addition, we observed negative correlations between subcutaneous IL-6 and IL-8 levels and the S/T-ratio. The lower the levels in adipose tissue, the higher the ratio is at 1, 24 and 48 h after surgery.
Taken together, both IL-6 and IL-8 seem to be progression parameters during and after heart surgery involving CPB. The reason for negative correlations is probably the development of capillary leakage syndrome. Increased vascular permeability causes a fluid shift into the interstitium, thus diluting inflammatory markers. Previous working groups measured both cytokines in the blood and reported both to be excellent predictive parameters in postoperative morbidity. On the strength of experience in our feasibility study, IL-6 and IL-8 seem to be reliable markers in monitoring the local inflammatory response also in adipose tissue. IL-10 is one of the typical immunosuppressive and immunostimulatory cytokines released during and after cardiac surgery with CPB. The greatest release occurs after the onset of CPB before pro-inflammatory cytokines increase to prevent the potentially harmful effects of inflammation [16]. Rising immunosuppression seems to be involved in developing the postoperative oedema and effusion, which may lead to CLS [7]. We noticed an age-dependent release of IL-10 immediately after CPB. A reason, therefore, is perhaps the ability of older children's more sophisticated immune response and more mature organ function. We cannot demonstrate a correlation between IL-10 and the S/T-ratio. Low interstitial IL-10 levels at the end of CPB is associated with higher serological lactate values 24 h after surgery. Considering our patients' low subcutaneous IL-10 levels and the age-related release, we believe that IL-10 is less appropriate as a predictive parameter in heart surgery of children within the first year of life.
The split products C3a and C5a are important markers when investigating complement activation during and after heart surgery. Seghaye et al. [6] reported significant complement activation via an alternative pathway in neonates undergoing CPB as indicated by the release of C3 and C5. Previous studies reported a rapid increase in complement at the end of CPB [17]. In line with those findings, we found out a maximum release of C3a during CPB, followed by a significant decrease over 24 h. One reason for this rapid drop could be the histamine liberation and leucocyte activation induced by the complement system, which leads to higher vascular permeability and effusion, which in turn dilute the local inflammatory mediators.
Differences in the inflammatory response between neonates and infants
We demonstrate that our newborns experienced longer mechanical ventilation times on the ICU, higher serological lactate levels and greater inotropic demand after surgery. We noticed no significant differences in bypass time, aortic-clamp time and leucocyte levels between these two age groups.
It is well documented that individual parameters can modify the immune response in humans, i.e. the severity of CHD [18], underlying diseases [6] and patient age. The non-specific innate immune defense works congenitally and is supplemented by the pathogen and antigen-specific response in the adaptive immune system. Orlikowsky et al. [19] reported on lower monocytes and macrophage activity in neonates—compared with adults. Other working groups have described significantly higher serological release of IL-6 and IL-8 in newborns [20]. In contrast, our infants revealed the tendency to present higher IL-6 levels. Due to our study cohort's small size, we cannot demonstrate significance. We observed no differences in IL-8 and IL-10. One reason for this discrepancy is perhaps the fact that five of our six neonates developed CLS. Increased vascular permeability makes fluid shift, causing interstitial cytokines to become diluted. This effect failed to become intravascularly apparent.
As a matter of fact, the data on activation of the complement system and neutrophil granulocytes are controversial. On the one hand, there are studies attributing a reduced alternative pathway of complement activation and immature complement receptors on neutrophils in newborns [21]. On the other hand, there are recent reports of a dramatic increase in complement factors in blood and leucocyte stimulation in children undergoing CPB [20, 22, 23]. We observed significantly higher C3a levels in newborns. Both our groups presented a drop in leucocytes 8 h after surgery.
Finally, we demonstrate differences between neonates and infants particularly in the release of cytokine IL-6 and the anaphylatoxine C3a. Diagnostic microdialysis enables us to show that infants undergoing CPB react with a much higher increase in IL-6 than neonates. Moreover, we observed statistically significantly higher C3a levels in the adipose tissue of newborns.
Microdialysis predicting capillary leak syndrome
Newborns and small infants undergoing congenital heart surgery with CPB have a high risk of developing CLS [2, 3]. The exposure of blood to foreign surfaces in the extracorporeal circuit activates the contact and complement system and leads to changes in adhesion molecules, which in turn causes the release of histamine, TNF and ILs [24]. Stiller et al. [23] showed serological differences in complement activation between infants who developed in process CLS, compared with those infants who did not develop CLS later. Of course, this effect exists only during surgery and in the first postoperative hours. In the present study, 6 of our patients (26%) developed CLS, 5 of whom were newborns. Their postoperative S/T ratios were significantly higher in newborns than in the older infants. Furthermore, the 12-h postoperative fluid balance was positive in patients with an S/T greater than the 12% that indicates a CLS. As risk factors for developing CLS we noticed longer bypass time, longer mechanical ventilation on the ICU, greater inotropic demands and elevated postoperative serological lactate levels mainly indicating sicker patients.
In contrast to results of previous studies reporting on higher intravascular (and in bronchoalveolar lavage) IL-6 and IL-8 levels in patients with fluid retention, we observed lower concentrations in adipose tissue in CLS [15]. We noted a strongly significant difference in IL-6 levels immediately after CPB. In the pathophysiology of CLS, the fluid shift from intravascular to interstitial space leads to the dilution of the incoming cytokines. We found no significant difference in IL-10 levels between the children with and without CLS.
Compared with IL-6 and IL-8, the anaphylatoxin C3a level was considerably higher in the CLS group. Whereas the patients without CLS presented a continuous decrease in C3a, those that did develop CLS demonstrated a gentle but significant second peak of C3a 8–10 h postoperatively. C3a appears to be a sensitive inflammatory marker that results in a new systemic release during CLS and depended on existing increased permeability to higher levels in interstitial spaces. Actually, our study finding reveals that microdialysis can reveal changes in inflammatory cytokines that may be associated with CLS. Furthermore, we believe that diagnostic microdialysis may eventually prove to be a promising new technique to predict CLS early 4–8 h after CPB and before severe clinical signs appear. At this juncture it is difficult to detect CLS in the individual patient. A promising method especially for further studies could be to calculate serum/microdialysis cytokine ratios.
Diagnostic microdialysis is a valid but elaborate method. We currently see microdialysis as playing a greater role in experimental research than in clinical routine. It may prove to be useful in further studies, such as testing and optimizing miniaturized CPB parts with a view to reducing morbidity, particularly in high-risk patients with small blood volume. A more homogeneous patient cohort will be advisable in future studies, and determining serum/microdialysis ratios may provide more information with which to detect early CLS.
Study limitations
Our feasibility study has several limitations that may affect its results. For one, our study cohort (containing 23 children) is relatively small and heterogeneous.
Cytokine activity is primarily local. We can only detect them systemically in the blood during an acute systemic inflammatory response. Inflammatory mediators are instable molecules with extremely short half-value periods due to endocytosis and subsequent proteolysis. Further factors influencing the recovery of inflammatory mediators could be the perfusion fluid, interactions with proteins, the infusion time of the microdialysis pump [25], diluting effects triggered by CPB and the postoperative development of CLS. Finally, our study is limited by the long sampling periods of 120 min each and the absence of preoperative data. It remains unclear which influence on the inflammatory response is solely attributable to CPB and which can be attributed to anaesthesia, medication and surgical stress.
CONCLUSION
In summary, our study results provide evidence of the feasibility of verifying subcutaneously the inflammatory response in newborns and small infants during and after CPB via microdialysis. Diagnostic microdialysis seems to be a pomising new technique to predict CLS on the cytokine level before severe clinical signs appear.
ACKNOWLEDGEMENT
We thank Carole Cürten for language editing.
Conflict of interest: none declared.
REFERENCES
Author notes
Presented at the 41st Annual Meeting of the German Society of Thoracic and Cardiovascular Surgery (DGHTG), Freiburg, Germany, 12–15 February 2012.
Rouven Kubicki and Jochen Grohmann contributed equally to this work.
- anti-inflammatory agents
- aorta
- cytokine
- cardiopulmonary bypass
- cardiac surgery procedures
- inflammation
- capillary leak syndrome
- complement activation
- infant
- newborn
- interleukin-10
- interleukin-8
- lactates
- microdialysis
- surgical procedures, operative
- adipose tissue
- diagnosis
- interleukin-6
- morbidity
- surgery specialty
- inotropic agents
- bypass
- catheters
- inflammatory response