-
PDF
- Split View
-
Views
-
Cite
Cite
Takuhiro Ikeda, Yoshihiro Miyata, Yasuhiro Tsutani, Keizo Misumi, Koji Arihiro, Morihito Okada, Fibrinogen/thrombin-based collagen fleece (TachoComb®) promotes regeneration in pulmonary arterial injury, European Journal of Cardio-Thoracic Surgery, Volume 41, Issue 4, April 2012, Pages 926–932, https://doi.org/10.1093/ejcts/ezr128
- Share Icon Share
Abstract
To repair unexpected damage of the pulmonary artery (PA) during thoracic surgery, fibrinogen/thrombin-based collagen fleece (TachoComb® [TC]) can be applied as a haemostatic material. The progression of vessel restoration with TC has not been elucidated. In this study, we investigate details of the healing process with TC after PA injury using a canine model.
Left thoracotomy was performed on female beagles under general anaesthesia. PA injury was induced and repaired using TC. Repair sites were histologically evaluated 2, 4 and 8 weeks after surgery (n = 3 in each group).
Haemostasis of PA injury was achieved promptly after TC application. After surgery, no bleeding was found in the thoracic cavity, and no repair sites revealed stenosis, thrombi or false aneurism formation. Two weeks after surgery, inflammatory cells had infiltrated around the vascular defect, and vascular endothelium had regenerated on the innermost surface of TC applied to the defect. At Week 4, elastic and smooth muscle fibres had begun to extend into the defect between the endothelial layer and collagen fleece. By Week 8, elastic fibres and smooth muscle had completely regenerated in the medial layer. The adventitial layer had also fully regenerated.
Haemostasis of injured PA using TC was safe and reliable. TC provided a mechanical scaffold on which vascular regeneration occurred. Three layers reconstructed in the PA defect were identical to those in normal structures.
INTRODUCTION
Vascular bleeding is a common problem encountered during surgical procedures. Bleeding is a particular source of concern in thoracic surgery because pulmonary resection involves exposure of large vessels such as the pulmonary artery (PA), in which minimal damage leads to massive bleeding. In the event of accidental PA bleeding, suturing is often used to repair the injury. This procedure requires advanced surgical techniques because precise suturing involves exposure and clamping of sites proximal and distal to the PA injury, all within a small surgical field. Such procedures are especially challenging during video-assisted thoracic surgery.
In recent years, TachoComb® (TC) (CSL Behring, Tokyo, Japan) has become widely used for the suture-free haematostasis of arterial and venous bleeding and haemorrhage in organ parencyma [1–6] or oozing of the suture line of vessels [7]. TC consists of a structural support made of a honeycomb equine collagen sheet coated with human fibrinogen and bovine thrombin. When it makes contact with host blood, human fibrinogen and bovine thrombin react to produce fibrin. The fibrin immediately polymerizes and becomes a stable fibrin clot that glues and closes the tissue [8].
Several studies describe the use of TC to prevent pulmonary air leakage during thoracic surgery [9–11]. There are fewer reports that discuss the use of TC for PA-bleeding haemostasis [12] and, to date, no studies address the subsequent healing of vessels treated with TC to achieve temporal haemostasis. If temporal haemostasis is achieved by application of TC to the PA defect, the next major concern is the mid- and long-term integrity of the vascular defect and whether it is vulnerable to stenosis, thrombi and pseudo-aneurysm formation. In this study, we use a canine model to examine the feasibility of using TC to control bleeding of the PA and to investigate the healing process of PA injury after the use of TC.
MATERIALS AND METHODS
Animal preparation and anaesthesia
Female beagles (Kitayama Labes Co. Ltd., Nagano, Japan), weighing ∼10 kg each, were used for this study. After pre-anaesthetization with ketaminol (10 mg/kg) and atropine sulphate (0.25 mg), intravenous access was established in a forearm vein. Propofol (5 mg/kg) and suxametnium (1 mg/kg) were administered to allow endotracheal intubation and coupling to an anaesthesia unit with a ventilator. Anaesthesia was maintained by periodic injection of propofol and suxametnium.
Surgical procedure
Animals were placed in a right decubitus position, and a left fourth-intercostal thoracotomy was performed. The left main PA (∼10 mm in diameter) was exposed and clamped, and a hole in the PA (3 × 3 mm) was carefully created with fine scissors. TC was then applied to cover the hole and compressed for ∼5 min (Fig. 1a and b). After the procedure, the clamp was released and complete haematostasis was confirmed (Fig. 1c). The chest wall was closed after air evacuation with a Nelaton catheter. Ketoprofen (1 mg/kg) and ampicillin sodium (15 mg/kg) were injected intramuscularly. After the animals recovered from anaesthesia, they were returned to their cages.
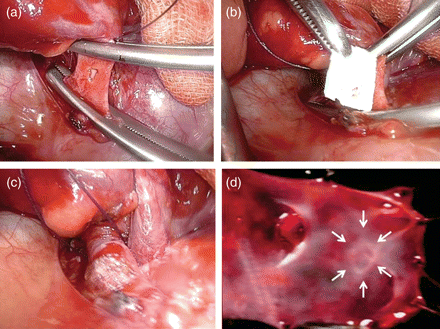
(a) Main trunks of PA were clamped and a PA hole was created. (b) TC was applied to cover the hole. (c) Five minutes after the TC application. (d) Intravascular lumen findings at 8 weeks. Repaired site (arrow) shows just a fine trace at the incision site.
Histological analysis
The animals were euthanized 2, 4 and 8 weeks after surgery (n = 3 in each group) by pentobarbital overdose, and the left PA was resected along with the left lung. The specimens were fixed in formalin, embedded in paraffin and cut into 4-μm sections. Repaired sites were histologically evaluated using haematoxylin–eosin, Elastica-van-Gieson, α-smooth muscle actin (α-SMA) and vascular endothelial growth factor (VEGF) staining.
Immunohistochemistry
Immunohistochemical staining of VEGF was performed using an indirect polymer immunoperoxidase method. Sections were placed on slides, deparaffined in xylene (3 × 3 min) and washed in absolute ethanol (3 × 2 min). The slides were heated in a water bath at 95–99°C for 20 min with epitope retrieval buffer (citrate buffer, 0.01 M, pH 6.0) and then cooled. Endogenous peroxidase was blocked by incubating slides in 3% H2O2 for 30 min at room temperature, followed by staining (Ultra Tech horseradish peroxidase) and incubation in Protein Block for 10 min. The primary antibody [mouse monoclonal VEGF antibody (ab1316; Abcam)] was applied and incubated overnight at 4°C. Slides were incubated for 60 min in a solution containing a biotinylated secondary antibody, followed by incubation with streptoavidin-peroxidase for 30 min. After washing in buffer, the slides were treated with diaminobenzidine substrate for 5 min, treated with haematoxylin, dehydrated and mounted.
The protocols for this study were approved by the Ethics Committee of Hiroshima University, and the guidelines for laboratory animal care [13] were observed.
RESULTS
Haemostasis of PA injury was achieved in all cases (n = 9) promptly after TC application (Fig. 1c). All animals survived through the observation period. At the time of sacrifice, no bleeding and no haematomas were found in the thoracic cavity. No repair sites revealed stenosis, thrombi or false aneurysm formation. Within the intravascular lumen of repair sites, the defect closed very well, showing just a fine trace at the incision site 8 weeks after surgery (Fig. 1d).
Histological analysis just after the application of the TC to the defect revealed that the TC was firmly attached, only on the PA defect, as two layers of collagen fleece (Fig. 2). After 2 weeks, fibroblasts and inflammatory cells (such as macrophages and neutrophils) had infiltrated the TC from around the vascular defect (Fig. 3a–c), and the vascular endothelium had already regenerated on the innermost surface of TC. There was no elastic fibre formation, as indicated by Elastica-van-Gieson staining, at this time point (Fig. 3d–f). α-SMA staining revealed many small neo-vascular vessels and myofibroblastic cells within the vascular defect, but no smooth muscle fibre (Fig. 3g–i). Newly generated vascular endothelial cells on the TC surface, as well as the neo-vasculature that formed in the TC collagen layer also showed strong expression of VEGF (Fig. 6a–c). At 4 weeks after surgery, myofibroblastic cells and a small amount of neo-vasculature remained in the former TC layer (Fig. 4a–c and g–i). Elastic and smooth muscle fibres had begun to extend into the defect between the endothelial layer and the TC from both edges (Fig. 4d–f and g–i). Endothelial cells and the neo-vasculature still expressed VEGF (Fig. 6d–f). At Week 8, elastic and smooth muscle fibres completely covered the defect below the endothelial layer (Fig. 5d–f and g–i), indicating that the medial layer had completely regenerated. VEGF expression on the endothelium disappeared at this point (Fig. 6g–i). The number of myofibroblastic cells and the amount of small neo-vasculature in the former TC layer were reduced, and the transition from this layer to the original adventitial layer was quite smooth (Fig. 5a–c and g–i), indicating that the reconstructed layer had become a complete, newly developed adventitial layer. Table 1 summarizes the histological evidence for these histopathological findings.
Weeks after injury . | Endothelial cells . | Elastic fibres . | Smooth muscle fibres . | Infiltration of inflammatory cells . | Expression of VEGF . |
---|---|---|---|---|---|
0 | (−) | (−) | (−) | (−) | (−) |
2 | (++) | (−) | (+) | (++) | (++) |
4 | (++) | (+) | (+) | (+) | (+) |
8 | (++) | (++) | (++) | (−) | (−) |
Weeks after injury . | Endothelial cells . | Elastic fibres . | Smooth muscle fibres . | Infiltration of inflammatory cells . | Expression of VEGF . |
---|---|---|---|---|---|
0 | (−) | (−) | (−) | (−) | (−) |
2 | (++) | (−) | (+) | (++) | (++) |
4 | (++) | (+) | (+) | (+) | (+) |
8 | (++) | (++) | (++) | (−) | (−) |
Weeks after injury . | Endothelial cells . | Elastic fibres . | Smooth muscle fibres . | Infiltration of inflammatory cells . | Expression of VEGF . |
---|---|---|---|---|---|
0 | (−) | (−) | (−) | (−) | (−) |
2 | (++) | (−) | (+) | (++) | (++) |
4 | (++) | (+) | (+) | (+) | (+) |
8 | (++) | (++) | (++) | (−) | (−) |
Weeks after injury . | Endothelial cells . | Elastic fibres . | Smooth muscle fibres . | Infiltration of inflammatory cells . | Expression of VEGF . |
---|---|---|---|---|---|
0 | (−) | (−) | (−) | (−) | (−) |
2 | (++) | (−) | (+) | (++) | (++) |
4 | (++) | (+) | (+) | (+) | (+) |
8 | (++) | (++) | (++) | (−) | (−) |
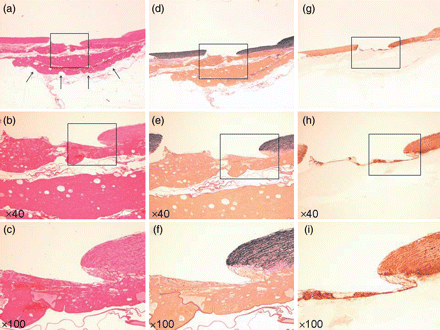
Specimen injured vessel with fresh TC. (a–c) Haematoxylin–eosin stain (arrow: TC). (d–f) EVG stain. (g–i) α-SMA stain. (a, d and g) Loupe. (b, e and h) ×40 Magnification. (c, f and i) ×100 Magnification.
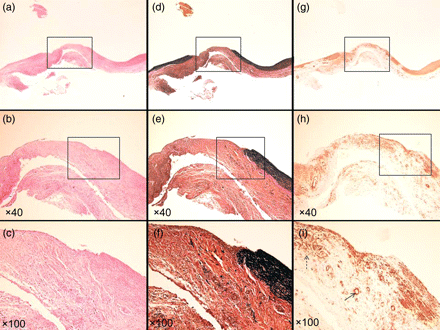
Specimen at 2 weeks. (a–c) Haematoxylin–eosin stain. (d–f) EVG stain. (g–i) α-SMA stain. (a, d and g) Loupe. (b, e and h) ×40 Magnification. (c, f and i) ×100 Magnification. Inflammatory cells gathered around the vascular defect and vascular endothelium had already been regenerated on the innermost surface of TC (c). Many neovascular vessels (arrow) and myofibroblastic cells (dotted arrow) can be seen in the vascular defect (i).
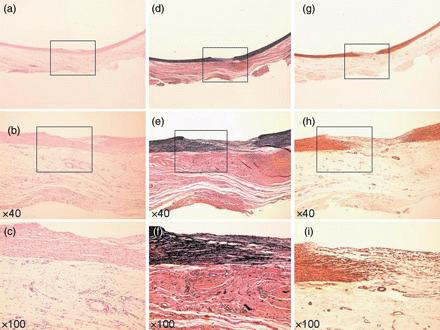
Specimen at 4 weeks. (a–c) Haematoxylin–eosin stain. (d–f) EVG stain. (g–i) α-SMA stain. (a, d and g) Loupe. (b, e and h) ×40 Magnification. (c, f and i) ×100 Magnification. Elastic fibre (e) and smooth muscle fibre (h) began to invade the defect between the endothelial layer and TC. Inflammatory cells remained in the defect (c).
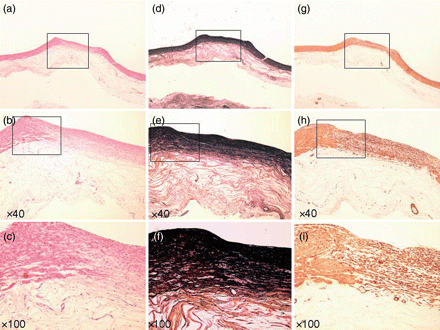
Specimen at 8 weeks. (a–c) Haematoxylin–eosin stain. (d–f) EVG stain. (g–i) α-SMA stain. (a, d and g) Loupe. (b, e and h) ×40 Magnification. (c, f and i) ×100 Magnification. Three-layer construction completely regenerated (b, c and h).
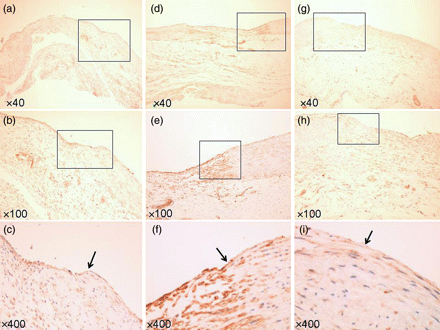
Specimen of VEGF staining. (a–c) At 2 weeks. (d–f) At 4 weeks. (g–i) At 8 weeks. (Arrow: border of TC and the intima.) (a, d and g) ×40 Magnification. (b, e and h) ×100 Magnification. (c, f and i) ×400 Magnification. Endothelial cells strongly expressed VEGF at 2 and 4 weeks.
DISCUSSION
For thoracic surgeons, injury of the PA during a surgical procedure is a major source of concern: a tiny vessel injury can be lethal, and the high flow rate of blood coupled with thin vessel walls makes repair difficult without significant technical skills. Precise exposure and clamping of the PA on the proximal and distal sites of the injury are mandatory in the repair using suturing. Although this technique is an essential skill for all thoracic surgeons, PA exposure and clamping after an unexpected PA injury is sometimes difficult, especially in cases of dense adhesions and limited surgical field. In video-assisted thoracic surgery, bleeding of the PA is the most frequent reason for conversion to thoracotomy; this statistic reflects the surgeons’ fears associated with PA bleeding [14]. Establishment of an easy and reliable haemostasis method is therefore important in thoracic surgery.
TC has been used to stop bleeding from organ parenchyma, but it has been thought to be difficult to use for major bleeding from a great vessel injury. In this study, we produced a relatively large defect on the canine PA, matching the largest size that we would expect to encounter in a human clinical setting. Canine's mean PA pressure and composition PA wall are known to be similar with human's [15]. Despite the size of the defect, haemostasis was easily achieved by clamping on the proximal and distal sites of the injury, applying TC onto the vessel defect, and compressing for several minutes. Haemostasis with TC has an advantage over suturing, as vessel stricture is avoided—even in cases requiring proximal clamping. When a vessel injury is relatively small and the amount of bleeding is small enough to be controlled with compression of the local wound site, TC can be applied without longitudinal PA exposure and clamping. Complete adherence and attachment of TC to the vessel wall in a relatively dry field is the most critical aspect of haemostasis with TC. In a preliminary experiment, we found that bleeding from an aortic defect of the same size (3 × 3 mm) was much more difficult to stop by TC without suturing, even after complete clamping, due to the high blood pressure in the aorta. The low blood pressure and thin vessel walls of the PA makes it suitable for TC attachment and compression of the injury site.
If temporal haemostasis is achieved during surgery, the next major concern of thoracic surgeons is the mid- and long-term integrity of the vascular defect. Re-bleeding, which is the most serious complication, stenosis, thrombi and pseudo-aneurysm formation, could occur at the repair site. In our study, we observed none of these conditions 2, 4 or 8 weeks after surgery. To our surprise, the defects were completely reconstructed with minimal circular scarring of the defect, appearing the same as native blood vessels at 8 weeks.
Blood vessels consist of three layers (in order from the luminal side outward): the intima (with endothelial cells), media (with elastic fibre and smooth muscle) and adventitia (with collagen fibres and fibroblasts). In our experiments, TC provided a mechanical scaffold on which these three complete layers were able to regenerate. To our knowledge, the promotion of vessel regeneration by TC has not been previously reported.
Reconstruction of all layers is crucial to the integrity of repaired vessels. Medial layer reconstruction is required to prevent pseudoaneurysms and stenosis, while reconstruction of the adventitial layer is needed to prevent infection and rupture of the vessel. Shinoka et al. [16] described the use of a reconstructed PA made of biodegradable tubular scaffolds seeded with autologous cells as a living vascular replacement graft. Although these tissue-engineered grafts were functionally analogous to homografts, the regeneration method was too cumbersome to apply in a clinical setting. Biomaterials in clinical use, such as polytetrafluoroethylene and polyethylene terephtalate fibres, exhibit no elasticity; their use can result in obstruction, stenosis and thrombosis in small-diameter vessels such as the coronary artery [17]. Our study demonstrates that TC can provide not only a fibrin glue to stop bleeding instantly, but also a mechanical scaffold on which complete three-layer vascular regeneration can occur in vivo. Our favourable outcomes were precisely due to complete regeneration of the three-layer vasculature.
We observed that fibroblasts, macrophages and neutrophils infiltrated the collagen honeycomb structure of the TC, and vascular endothelium regenerated on the inner TC surface at the early phase of the application of TC to the defect. Neo-vasculature formed in the TC collagen layer, and vascular endothelium on the luminal surface showed high levels of VEGF expression. Thus, the equine collagen honeycomb structure of the TC itself provided an ideal scaffold for inflammatory cells, neo-vasculature formation and endothelial cells, resulting in the release of growth factors that promote vessel regeneration. Extension of elastic and smooth muscle fibres then formed a complete medial layer. Among cells accumulated in the TC, myofibroblasts have been shown to play a key role in vessel remodelling. Myofibroblasts are derived from fibroblasts in the adventitia, smooth muscle cells in the media and endothelial cells in the intima through endothelial–mesenchymal transition. Differentiation of myofibroblasts promotes wound healing through transforming growth factor-β and VEGF [18]. In the present study, VEGF expression and inflammatory cell infiltration disappeared 8 weeks after injury, and the TC itself became unified with the host adventitial layer.
In conclusion, we observed that haemostasis of PA defects using TC was safe and reliable. In the process of healing from PA injury, TC provided a mechanical scaffold on which vascular regeneration occurred, inducing reconstruction of the three layers of the PA wall.
Conflict of interest: none declared.
REFERENCES
- inflammatory cells
- fibrinogen
- pulmonary artery
- hemorrhage
- hemostatic function
- smooth muscle
- lung
- aneurysm
- vascular endothelium
- anesthesia, general
- collagen
- constriction, pathologic
- dog, domestic
- endothelium
- objective (goal)
- hemostatics
- surgical procedures, operative
- thoracotomy
- hemostasis procedures
- surgery specialty
- thoracic surgery procedures
- thrombin
- thrombus
- arterial injuries
- thoracic cavity
- myocytes, smooth muscle
- elastic fibers