-
PDF
- Split View
-
Views
-
Cite
Cite
Remi Neviere, David Montaigne, Lotfi Benhamed, Michele Catto, Jean Louis Edme, Regis Matran, Alain Wurtz, Cardiopulmonary response following surgical repair of pectus excavatum in adult patients, European Journal of Cardio-Thoracic Surgery, Volume 40, Issue 2, August 2011, Pages e77–e82, https://doi.org/10.1016/j.ejcts.2011.03.045
- Share Icon Share
Abstract
Objective: Severe pectus excavatum are common in adult patients, often causing psychological complaints and physiological impairments. Although lung function at rest may minimally deteriorate after surgical correction, it remains unclear if surgery improves exercise capacity. The objective of present study is to assess whether the surgical repair of pectus excavatum in adults would improve exercise tolerance. Methods: A prospective study was performed to compare pulmonary and cardiovascular function at rest and at maximal exercise, before, and at 1 year after pectus excavatum repair. Results: From December 2005 to May 2009, 120 adult patients underwent pectus excavatum repair. Of these patients, 70 (nine women, 61 men) underwent thorough preoperative, 6-, and 12-month postoperative assessments, and were included in the present study. Age ranged from 18 to 62 years (mean 27 years). The pectus index (Haller index) was 4.5 ± 1.1. Lung function tests at rest were within the normal range, whereas maximal oxygen uptake (peak VO2) was only 77 ± 2% of the predicted value. At 1-year follow-up, the pectus excavatum repair was associated with minor changes in lung function tests and significant increase (p = 0.0005) in VO2 (87 ± 2% of the predicted value). Postoperative O2 pulse increase at maximal exercise suggested that aerobic capacity improvement was the result of better cardiovascular adaptation at maximal workload. Conclusion: These results demonstrate sustained improvement in exercise cardiopulmonary function at 1-year follow-up of pectus excavatum surgical repair in adult patients.
1 Introduction
Pectus excavatum (PE), the most common congenital deformity of the chest wall, occurs in approximately 1 of 300–400 births [1]. No consensus exists among physicians as to whether or not the PE deformity produces symptoms that are severe enough to justify a surgical procedure during childhood [2]. Although many pediatric patients remain asymptomatic, symptoms can develop at puberty as physical activity increases, or with worsening of the PE during the second growth spurt [3]. In adults, the chest-wall deformity may be associated with subjective symptoms, such as chest discomfort, chest pain on exertion, and exercise intolerance, as well as moderate lung restriction [4].
An important consideration regarding the decision for PE repair is the identification of defects that could be improved upon surgery. In patients with PE, it remains unclear if surgery improves pulmonary and cardiovascular functions [5–7]. In children, pulmonary function after surgical PE repair has been investigated, with conflicting results. Some investigators have reported improved lung function tests after surgical correction, whereas others have found either no change [6] or deterioration [2]. Growing evidence accumulated in the recent literature suggests, however, that PE repair in childhood is associated with exercise-tolerance improvement [7]. To the best of our knowledge, long-term cardiopulmonary effects of surgical repair in adults with PE have not been previously reported [8]. The present study aims at assessing whether surgical repair would improve exercise tolerance in a large population of adult patients with severe PE.
2 Patients and methods
2.1 Patient selection and study design
All patients referred for evaluation of PE were seen through a single surgical unit. After institutional review board approval, the patients considered for surgical treatment were screened prospectively using a standardized protocol of chest computed-tomography imaging, and cardiopulmonary function studies including lung function tests, exercise tolerance, and echocardiography. Calculation of the pectus index (Haller index) was used to grade the chest-wall deformity [3]. Informed consent was obtained for study enrolment, which consisted of collection of the initial patient data, repeated echocardiography and chest computed-tomography scan, and cardiopulmonary function including exercise testing at 6- and 12-month follow-up. Patients with co-existing cardiopulmonary disease or a Marfan syndrome were excluded. At enrolment and after surgery, patients were reviewed using open-ended questions to evaluate their physical activity.
2.2 Cardiovascular function
Resting cardiovascular function was evaluated using standard supine transthoracic echocardiography (Vivid7 GE Healthcare, France) at the time of evaluation and 6 months after surgery. Echocardiography indices were computed by standard methods. The left-ventricular ejection fraction (LVEF) was analyzed in an apical four-cavity incidence by Simpson’s method. Pulsed Doppler recorded mitral inflow with the sample cursor placed at the mitral valve tips. Early diastolic velocity was recorded from Doppler tissue imaging, with the sample cursor placed on the lateral mitral annulus. To assess a patent foramen ovale, all patients underwent a microbubble test during normal breathing at rest and during a Valsalva maneuver, with evaluation of microbubbles appearing in the left atrium.
2.3 Lung function tests and exercise tolerance
Lung function tests were performed at the time of evaluation and 12 months after surgery. Spirometry, body plethysmography, and diffusion lung capacity of carbon monoxide (DLCO) were performed/evaluated according to American Thoracic Society standards [9] using the Jaeger MasterLab (MasterScreen Pneumo, Viasys, France). The spirometer was systematically calibrated immediately before each test using a 3-l calibrating syringe. The highest value for forced expiratory volume in 1 s (FEV1) and forced vital capacity (FVC) after three reproducible trials was recorded and compared with predicted normal values. Results were compared with predicted normal values for lung volumes. DLCO was measured by the single-breath technique.
Incremental exercise tests were performed on a calibrated electromagnetically braked cycle ergometer (ER-900; Jaeger, Hochberg, Germany), using a 1-min step protocol at 10 W min−1 until exhaustion [10]. Maximal incremental exercise was performed with the subjects maintaining a pedaling frequency of 60 ± 5 revolutions of the crank per min. Peak exercise was defined as the highest work level reached during the incremental exercise test. During testing, continuous non-invasive monitoring of heart rate and blood pressure was done (Marquette electrocardiogram system, Marquette Electronics, WI, USA). Cardiopulmonary exercise testing was performed with a metabolic cart (Oxycon Pro, Viasys, France). Continuous measurement of inspired and expired oxygen and carbon dioxide output were done with in-line sensors. Minute ventilation (VE) and CO2 produced (VCO2) values acquired from the initiation of exercise to peak exercise were input into spreadsheet software (Microsoft Excel, Microsoft Corp., Paris France) to calculate the VE/VCO2 slope via least squares linear regression. Peak O2 pulse was calculated by dividing peak oxygen uptake (VO2) by heart rate at the time of peak VO2.
2.4 Operative technique
All patients underwent a simplified Ravitch-type PE repair through a limited transversal, or sub-mammary incision in female patients. The elongated cartilages were resected subperichondrially (generally total bilateral cartilage resection from 3rd to 6th, limited to the extremities of the 7th cartilages). An optional transverse anterior osteotomy of the upper sternum, followed by elevation of the distal sternum, was then performed. Sternal stabilization was achieved using an easily removable metallic strut (Medicalex, Bagneux, France), which was removed under local anesthesia on an outpatient basis, 6 months after the initial procedure.
2.5 Statistical analysis
The reference values for lung function tests and exercise testing indices were based on age, gender, height, and weight. Statistical comparisons were done, by Student’s paired t-test, on data normalized as to patient’s age and weight. Non-parametric values were compared by the Wilcoxon’s signed sum rank test. A p value ≪0.05 was considered significant. All statistical analyses were conducted with a commercially available software program (Sigmastat, version 4.0; Jandel Co; San Raphael; CA; USA). Results are reported as mean ± SD. p values of ≪0.05 were considered significant for all analyses.
3 Results
3.1 Patient characteristics
From December 2005 to May 2009, 120 adult patients underwent a PE surgical repair and had bar removal 6 months after the initial procedure. Of these 120 patients, only 70 (nine women, 61 men) underwent thorough preoperative, 6-, and 12-month postoperative assessments, and could be included in the present study. Their mean (±SD) age was 27 ± 11 years (18–62 years), height was 1.80 ± 0.08 m, body weight was 67 ± 8 kg, and pectus index was 4.5 ± 1.1. Fifty patients (80%) performed moderate aerobic activity during 2 ± 1 h per week, 10 patients had no physical activities, and 10 patients were considered as trained athletes.
3.2 Echocardiography, lung function tests, and exercise tolerance
Specific testing of cardiac function at rest showed that cardiac indices were within the normal ranges (Table 1 ). Twenty of the patients (28%) had patent foramen ovale, which was graded moderate (10–15 bubbles). As shown in Table 2 , the observed values for FVC, FEV1, total lung volume (TLV), functional residual capacity (FRC), residual volume (RV), and DLCO before surgery were lower than predicted values. However, percent of predicted values for FVC, FEV1, TLV, FRC, and RV was within the normal ranges. Observed peak VO2 was evaluated and anaerobic threshold was calculated by the V-slope method, in which VCO2 is plotted against VO2 on X–Y coordinates of equal scale. Mean observed anaerobic threshold was 20.3 ± 5.3 ml kg−1 min−1, which corresponded to 58 ± 9% of the peak VO2 value. At anaerobic threshold, VE was 38 ± 10 l min−1 (112 ± 13% of the predicted value). The absence of major hyperventilation was reinforced by the findings that mean VE/VO2 was 29 ± 4 at anaerobic threshold. Mean observed peak VO2 was 34.9 ± 5 ml kg−1 min−1 (77 ± 14% of the predicted value). VE at maximal exercise was 83 ± 18 l min−1 (54 ± 11% of the predicted maximum value). At maximal exercise, tidal volume to FVC ratio was 49 ± 12. The mean observed maximum heart rate was 174 ± 19 beats min−1 (91 ± 9% of the predicted maximum value). Observed values for O2 pulse were 13.2 ± 3.1 ml beat−1 (91 ± 16% of the predicted value). Gas-exchange mechanisms at maximal exercise indicated moderate hyperventilation response as judged by ventilatory equivalents (mean VE/VO2 36 ± 6) and peripheral arterial CO2 partial pressure (PaCO2 4.66 ± 0.53 kPa). Resting values of O2 partial pressure alveolar–arterial gradient were normal in patients with and without patent foramen ovale (1.46 ± 0.39 and 1.59 ± 0.53 kPa, respectively). Oxygen partial pressure alveolar–arterial gradient was 2.26 ± 0.66 kPa in patients without foramen ovale and 3.33 ± 1.19 kPa in patients with patent foramen ovale.
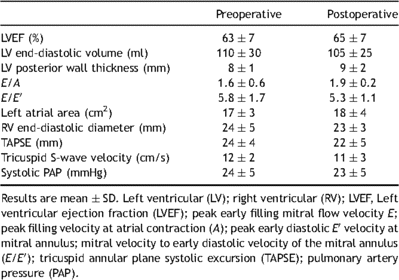
Pre- and postoperative transthoracic echocardiography evaluation.
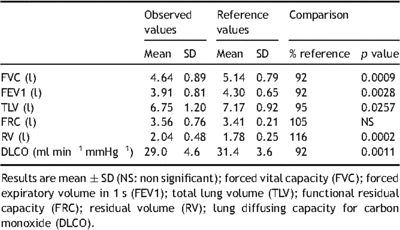
Comparison of observed lung function tests and references values.
3.3 Patient follow-up
Satisfactory anterior chest-wall contour was obtained in all patients. Evaluation of physical activity following surgery was unchanged compared with preoperative exercise habits. The mean postoperative pectus index was 2.7 ± 0.5, and postoperative echocardiography found no major functional changes (Table 1). Microbubbles shunting to the left atria, suggesting patent foramen ovale, were present in 20 patients before surgery and only in 10 patients after PE repair. Results of lung function tests are presented in Tables 3 and 4 . Measures of FVC, FEV1, TLV, and RV at rest were only slightly reduced 12 months after surgery (Table 3). Analysis of pre- and postoperative exercise testing parameters (Table 4) showed significant improvement of both observed anaerobic threshold and peak VO2 value. Postoperative peak VO2 was achieved at similar maximum heart rate systolic arterial pressure and VE/VCO2 slope compared with the preoperative value (Table 4). Peak VO2 increase was accompanied with increased maximal VE and O2 pulse compared with preoperative value (Table 4, Fig. 1 ). Change in maximal VE was mainly the result of respiratory rate increase at maximal workload. At maximal exercise, tidal volume to FVC ratio was 51 ± 13. Postoperative increase in O2 pulse was higher in patients with patent foramen ovale (13.4 ± 2.5 vs 15.7 ± 2.6 ml beat−1) compared with patients without foramen ovale (13.2 ± 3.1 vs 14.6 ± 3.2 ml beat−1), whereas improvement in peak VO2 was similar in both groups. Although two PE patients with patent foramen ovale had significant hypoxemia (PaO2 ≪ 10 kPa) before surgery, there were no changes in postoperative values of O2 partial pressure alveolar–arterial gradient at maximal VO2 compared with preoperative values (3.33 ± 1.19 vs 2.26 ± 0.66 kPa; p > 0.05). Postoperative increase in peak VO2 was higher in trained athletes compared with non-trained patients (42.1 ± 6.0 vs 37.6 ± 7.1 ml kg−1 min−1), whereas their training program was unchanged after surgery.
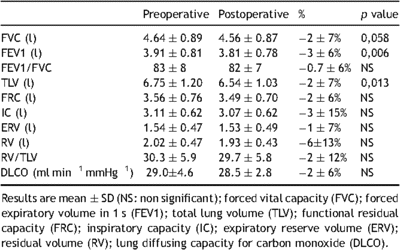
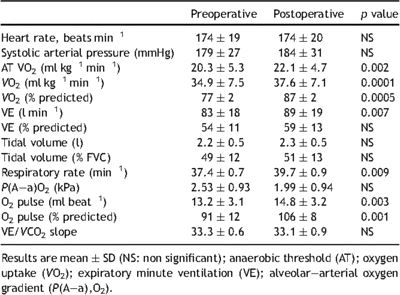
Pre- and postoperative cardiopulmonary values observed at maximal exercise.
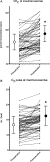
Individual and mean ± SD values (70 patients) of pre- and postoperative cardiopulmonary values observed at maximal exercise (panel A: peak VO2 expressed as percent of predicted values; panel B: oxygen pulse expressed in ml beat−1). * indicates p ≪ 0.05.
4 Discussion
There is still a controversy in the medical community whether actual physiological limitations exist in PE patients and whether surgical correction might improve cardiopulmonary function [5–8]. There is evidence from the available literature that PE repair has been implemented in pediatric and adolescent groups of patients [8]. To the best of our knowledge, there is no information regarding the physiological impact of PE repair in a homogeneous population of adults. We here report that PE surgical repair improved exercise tolerance in the largest series of patients with pre- and postoperative cardiopulmonary functional evaluation.
Our study examined resting lung function tests before and 1 year after PE repair. We found that preoperative spirometry values and lung volumes in PE adult patients were lower than predicted values, yet within the normal ranges. One year after surgery, there were modest reductions in FVC, FEV1, TLC, and RV, in the range of 2–6%, owing to the postoperative chest elasticity decrease [11,12]. These results were consistent with studies showing postoperative moderate decreases in FVC, FEV1, and TLC after both Nuss and Ravitch-type surgical procedures [3,6,7]. In these previous studies, lung function tests performed after Ravitch-type PE repair demonstrated an increased restriction despite symptomatic improvement and increased antero-posterior chest diameter, but restricted anterior chest-wall motion [11,12].
The major findings of the present study were twofold. First, preoperative peak VO2 was 77% of predicted value, which represents a consistent reduced aerobic capacity. This is in line with previous studies showing significant decreases in O2 uptake and O2 pulse in PE patients, which was not explained by physical deconditioning [13,14]. Second, aerobic exercise tolerance was significantly improved after PE repair, despite the absence of postoperative improvements in resting lung tests and resting cardiac function evaluated by transthoracic echocardiography. This is consistent with some reports of physical activity improvement and better subjective exercise tolerance after PE repair by different surgical approaches [5,7,8]. Beneficial effects of surgical repair on exercise performance testing remain, however, controversial [8]. Our study suggests that PE patients achieved higher maximal workload after surgery, which was associated with increased peak VO2, maximal VE, and O2 pulse. At maximal workload, none of our patients had VE limitation, expressed as the percentage of predicted maximal VE. In comparison with preoperative values, postoperative maximal VE increased as the result of maximal respiratory rate increases, whereas maximal tidal volume was unchanged. The inability to increase tidal volume was further reflected in the lower than expected tidal volume to FVC ratio at maximal exercise. These findings might be related to the effects of chest surgery leading to lung volume reduction despite a significant increase in the antero-posterior diameter of the chest. In fact, patients with pulmonary restriction are typically not able to increase tidal volume when needed, as with exercise [11,12]. It is our contention that the PE patients have pre- and postoperative dynamic pulmonary restriction that cannot be predicted from baseline lung function testing.
In healthy individuals, exercise tolerance is limited by the cardiovascular response and the degree of physical fitness, whereas no VE limitation is typically achieved [10]. In our study, as PE patients had no preoperative ventilatory limitation, better postoperative exercise tolerance could be related to either increased muscular fitness, improvement in the cardiovascular response, or both. To ascertain that subjects did not change their exercise habits after operation, we use an open questionnaire to control fitness levels before and after surgery. The absence of relationship between a postoperative patient’s physical training changes and aerobic capacity alterations was particular easy to demonstrate in athletic patients. As these patients experienced long-term, constant, and heavy training protocols before and after PE repair, observed maximal VO2 increases after surgery could not be attributed to changes in their exercise habits.
We reasoned next that postoperative increased aerobic capacity was the result of an improved cardiovascular response to exercise. Indeed, in PE patients, the cardiovascular response might be limited by the chest deformity leading to right-ventricle compression and changes in right-ventricular geometry [2,3]. Because abnormal cardiac anatomy might be difficult to quantify by routine transthoracic echocardiography, it remains controversial whether PE repair might improve baseline cardiovascular function [15]. However, intra-operative transesophageal echocardiography performed during PE repair clearly showed that LV ejection fraction significantly increased as the result of end-diastolic right-ventricular diameter and volume increases [16,17]. These findings suggest that, in PE patients, cardiac filling and cardiac output might be limited by the result of compression by the displaced sternum on the right heart chambers, thereby limiting increase of stroke volume. At exercise, these changes might impede further the heart’s ability to increase the stroke volume, to meet increased metabolic demands. For example, a recent study elegantly showed that patients with PE exercising in the sitting position had reduced aerobic capacity and stroke volume, whereas they approached control values during supine exercise when gravity augments venous return [18]. These findings suggest that PE repair could improve cardiac filling and cardiac output at maximal workload as a result of the chest’s antero-posterior diameter increase. In our study, repositioning the intrathoracic structures after PE repair improved cardiovascular adaptation at maximal exercise. This contention was based on O2 pulse calculation, a surrogate of cardiovascular function, because direct methods to study cardiac function are difficult to handle during maximal exercise testing. Likewise, we interpreted the closure of patent foramen ovale in 50% of cases after surgery as the result of repositioning the intrathoracic structures, which allows septum primum and secundum co-aptation. Postoperative increase in O2 pulse was even higher in PE patients with preoperative patent foramen ovale, compared with the overall PE population.
In conclusion, our results suggested that PE repair resulted in sustained improvement in both aerobic exercise tolerance and oxygen pulse, a surrogate of cardiac function. In our series, the presence of preoperative patent foramen ovale delineated a subgroup of patients with intense cardiovascular benefit due to PE surgical repair. These results might provide useful insight into the mechanisms that underlie both subjective and objective improvements of physical activity experienced by adult patients following the PE correction, mainly in trained athletes.
Acknowledgments
Authors thank the staff of the Lung Function and Laboratory for their skilful support during the study.
References
- congenital funnel chest
- maximum oxygen uptake
- lung
- exercise
- adult
- cardiovascular physiology
- cardiovascular system
- exercise tolerance
- follow-up
- preoperative care
- surgical procedures, operative
- workload
- pulse
- pulmonary function tests
- pulmonary function
- reconstructive repair of pectus excavatum
- aerobic capacity