-
PDF
- Split View
-
Views
-
Cite
Cite
Soichiro Funaki, Noriyoshi Sawabata, Tomoyuki Nakagiri, Yasushi Shintani, Masayoshi Inoue, Yoshiki Kadota, Masato Minami, Meinoshin Okumura, Novel approach for detection of isolated tumor cells in pulmonary vein using negative selection method: morphological classification and clinical implications, European Journal of Cardio-Thoracic Surgery, Volume 40, Issue 2, August 2011, Pages 322–327, https://doi.org/10.1016/j.ejcts.2010.11.029
- Share Icon Share
Abstract
Objective: The presence of isolated tumor cells (ITCs) in the pulmonary vein (PV) of a lung resected for lung cancer has been reported to be a prognostic factor. Previous investigations noted correlations between prognosis and the presence or amount of ITCs, although few studies have investigated the clinical implications of the morphological characteristics of those cells. We assessed the clinical implications of ITCs in the PV using a novel enrichment approach that maintained their morphological characteristics. Methods: Ninety-four consecutive patients with primary non-small-cell lung cancer (NSCLC) without preoperative chemo- and/or radiation therapy (p-stage I in 75, II in 13, III or IV in six) were studied. Blood samples were drawn from the PV draining the lung just after pulmonary resection, and ITCs were enriched using a CD45-negative selection method and density-gradient centrifugation, followed by Papanicolaou staining using 1 ml of PV blood and immunohistochemical staining for cytokeratin in cases with an additional available blood sample. The ITCs were classified into four types based on patterns of cluster formation: no tumor cells (N), singular tumor cells (S), clustered cells (≤0.2 mm) (CSs), and bulky clustered cells (>0.2 mm) (BCSs). We evaluated the correlations between ITC morphology and clinical results. Results: ITCs were detected in 68 of 94 patients (72%), of which the BCS type was observed in two, CS in 33, S in 33, and N in 26. Over a median follow-up period of 13 months (range 6–22 months), cancer recurrence occurred in 16 cases (17%): 14 in the combined CS/BCS group, one in S, and one in N. Log-rank analysis revealed that the disease-free survival rate was exclusively worse in patients with clustered ITCs as compared with the other two groups (p ≪ 0.01). Conclusions: The present method was useful to detect and enrich ITCs from the PV, and showed the clinical relevance of their morphology in lung cancer cases. The presence of ITC clusters may be a prognostic biomarker for patients with resected NSCLC.
1 Introduction
Primary lung cancer remains a leading cause of cancer death in most industrialized countries [1], with most cancer deaths related to high rates of recurrence and distant metastasis; thus, useful biomarkers are needed for early detection of both. Recently, isolated tumor cells (ITCs) in blood were reported to be useful markers for prognosis, recurrence, and metastasis [2]. It has been speculated that ITCs are likely shed from the primary tumor, then flow through a drainage vein and circulate throughout the body.
A number of methods to detect ITCs have been reported, with polymerase chain reaction (PCR)-based assays the most widely used [3], as a high sensitivity of ITC detection and clinical implications of the results have been shown [4–6]. Recently, the CellSearch® System (Veridex LLC, Rartian, NJ, USA) was shown to provide accurate detection and enrichment of rare ITCs from blood samples of patients with various types of solid cancer, including lung cancer [7], colon cancer [8], and breast cancer at the single cell level [9]. In addition, associations between the number of ITCs with tumor stage and progression have been reported [10]. Most previous studies were quantitative investigations used to evaluate ITCs, and there are few reports of the clinical implication of ITCs that used morphological classification. We conducted the present investigation to assess the clinical implications and morphological characteristics of ITCs in the PV of resected lungs of non-small-cell lung cancer (NSCLC) patients using a novel approach for ITC enrichment and detection.
2 Patients and methods
2.1 Cell-spiking experiment
Initially, to evaluate the accuracy and sensitivity of our method to detect ITCs, a cell-spiking experiment was performed using two lung cancer cell lines, COR-L32 (human small-cell lung carcinoma; ATCC® Catalogue No. 96020744) and SK-LU-1 (human adenocarcinoma; ATCC® Catalogue No. HTB-57™), prior to the examination of clinical samples to detect ITCs in blood samples. The cell lines were maintained in Eagle’s Minimum Essential Medium (EMEM) supplemented with fetal bovine serum (FBS) to a final concentration of 10% and incubated at 37 °C. After adding a known number of cells to 1-ml whole blood samples obtained from healthy volunteers, we assessed the sensitivity of our method by determining the ratio of the number of enriched cells to the number of added cells with a hemacytometer.
The method of cell extraction employed was as follows. A RosetteSep® Human CD45 Depletion Cocktail (Stemcell Technologies, Inc.) was added at 50 μl ml−1 to individual whole blood samples and mixed well. After incubation for 20 min at room temperature, the mixture was diluted with an equal volume of phosphate-buffered saline + 2% fetal bovine serum (PBS + 2% FBS) and mixed gently. The diluted sample was then layered on top of a Ficoll-Paque™ PLUS and centrifuged for 20 min at 1200 × g at room temperature, with the brake in the off position; then, the enriched ITCs were removed from the Ficoll-Paque™ PLUS–plasma interface. After washing enriched ITCs with PBS + 2% FBS, the cells were centrifuged down to polylysine-coated glass slides using a cytospin device at 1000 × g for 3 min. Cells on the slides were stained using Papanicolaou stain and a cytokeratin immunohistochemistry kit (Carcinoma cell detection kit human, Miltenyi Biotec® Catalogue No. 130-090-463), which resulted in a cell collection ratio of 60% for both single cells and clustered cells. Tumor cells that existed among the total nuclear cell count ranged from 1 × 102 to 1 × 103 in number.
2.2 Patients
Ninety-four consecutive patients (56 males, 38 females; range 28–88 years old, median 67.7 years) with primary NSCLC, who did not undergo preoperative chemo- and/or radiation therapy, were evaluated using our method (Table 1 ). Written informed consent was obtained from all patients enrolled. This study conformed to the ethical guidelines of Osaka University Graduate School of Medicine, and was approved by the institutional review board of Osaka University Medical Hospital. All patients underwent a segmentectomy (n = 11), lobectomy (n = 80), or bilobectomy (n = 3) with a systematic mediastinal lymphadenectomy from August 2008 to January 2010 at Osaka University Medical Hospital.
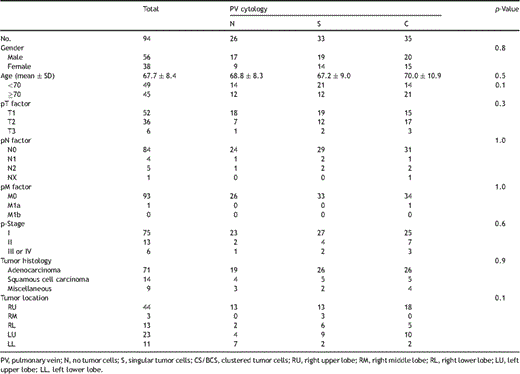
Patient characteristics and distribution of isolated tumor cells in pulmonary venous blood.
The postoperative staging of all patients was determined according to the tumor–node–metastasis (TNM) classification of the Union for International Cancer Control (UICC), ver. 7, 2009 (Table 1). The median follow-up duration was 13 months (6–22 months). In follow-up examinations, all patients were evaluated at 3-month intervals. Each evaluation included a physical examination, chest X-ray, and blood tests including tumor markers, while additional thoracic–abdominal computed tomography (CT) scans were generally performed at 6-month intervals.
2.3 Blood samples and ITC detection and enrichment
All blood samples were collected on the back table immediately after lung resection by gentle aspiration with an 18-gauge needle from the tumor-draining pulmonary vein (PV), which was stapled before the resection in all cases, and placed in 10-ml ethylene diamine tetraacetic acid (EDTA) tubes. ITCs were isolated using a negative selection method from 1-ml blood samples by the method described above. In addition, cytokeratin immunohistochemistry was performed, if an additional blood sample was available.
2.4 Evaluation and classification of clusters
Using all of the samples, one glass slide containing enriched ITCs from each patient was prepared and assessed by Papanicolaou staining. This examination was performed independently by two cytologists (E.Y and H.Y) who were unaware of the patient’s clinical data. For morphological assessment, each cytologist distinguished cancer cells from normal cells by light microscopy based on their morphological appearance, such as cell size and shape, nuclear size and shape, and nuclear–cytoplasmic ratio (N/C). Furthermore, for cluster formation assessment, patterns of ITCs were classified into the following four types: no tumor cells (N), singular cells (S), clustered cells (≤0.2 mm in size) (CSs) including singular cells, and bulky clustered cells (>0.2 mm in size) (BCSs), which included clustered cells and singular cells.
2.5 Statistical analysis
Statistical analysis was performed using the SPSS Exact Tests software. The Kruskal–Wallis test was used to calculate mean values, and prevalence was analyzed with Fisher’s exact test. For analysis of follow-up data, survival curves were calculated with the Kaplan–Meier method and survival distributions were compared by a log-rank test. The Cox proportional hazards model was applied for calculating hazard ratio by uni- and multivariate analyses. The threshold for statistical significance was a p-value less than 0.05.
3 Results
3.1 Detection of ITCs
Using Papanicolaou staining, ITCs classified as S were detected in 33 (35%) of the 94 patients, while those classified as CS or BCS were found in 35 (37%). Fig. 1 shows examples of singular and cluster formations of CTCs. Cases classified as CS and BCS were considered as a single group for analysis of survival, as the number of BCS cases was small. Cytokeratin examinations were performed in 59 cases (15 classified as N, 21 as S, 22 as CS, and one as BCS). All cases classified as CS or BCS revealed positive results for cytokeratin staining, whereas only seven (33%) of those classified as S and none as N showed positive cytokeratin staining results.
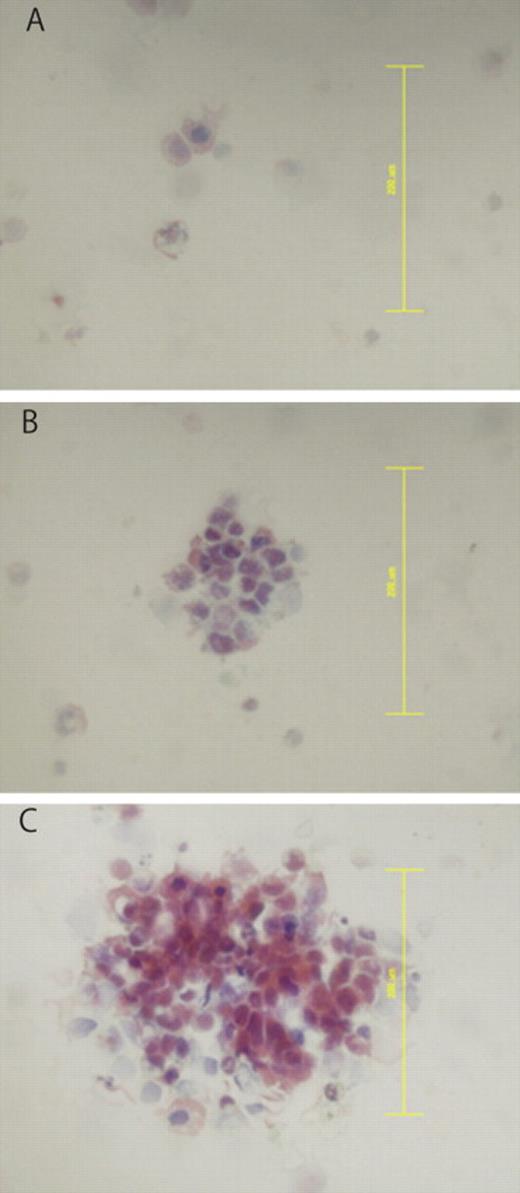
Classification of isolated tumor cells by cluster formation. Shown are results following Papanicolaou staining. (A) Arrows indicate singular cancer cells. (B) Clustered cancer cells (≤0.2 mm). (C) Bulky clustered cells (>0.2 mm). Original magnification ×40. Scale bars = 0.2 mm.
3.2 ITC classification and prognosis
The correlations of patient characteristics with distribution of ITCs in pulmonary venous blood are shown in Table 1. There were no significant differences regarding patient characteristics among the three groups (N, S, and CS/BCS). Sixteen patients suffered cancer relapse, including 14 in the CS/BCS group, one in S, and one in N: these could be detailed as exclusive local recurrence in four (pleura in one, chest wall in one, hilar lymph node in one, mediastinal lymph node in one), local and distant metastasis in seven (bilateral lungs in four, mediastinal lymph node and neck lymph node in two, mediastinal lymph node and bone in one), and exclusive distant metastasis in five (contralateral lung in three, adrenal gland in one, and brain and liver in one). Relapse-free survival curves are shown in Fig. 2 , which demonstrated that the cluster group (CS/BCS) exclusively was statistically significant; overall survival curves are shown in Fig. 3 . Univariate analysis revealed that the presence of clustered ITCs and p-stage III or IV were significant prognostic factors in the analysis of disease-free survival, and those results were confirmed by multivariate analysis findings (Table 2 ).
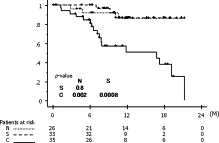
Relapse-free survival curves.
N, patients with no tumor cells; S, patients with singular tumor cells; C, patients with clustered tumor cells (CS/BCS).
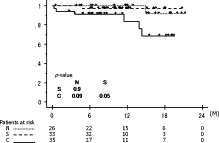
Overall survival curves.
N, patients with no tumor cells; S, patients with singular tumor cells; C, patients with clustered tumor cells (CS/BCS).
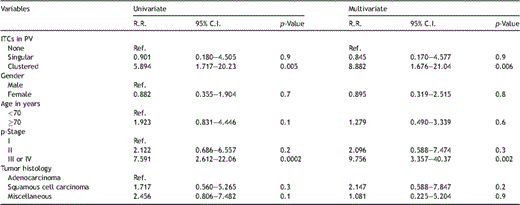
Results of univariate and multivariate analyses of relapse-free survival.
4 Discussion
In the present study, we focused on the morphological appearance of ITCs and investigated related clinical implications. Our results showed that cluster formations of ITCs may be a prognostic indicator for early recurrence of lung cancer. In cases with singular cancer cells, the recurrence rate was low as compared with those with cluster formation. However, the clinical implications of the presence of singular ITCs remain unclear and a longer duration follow-up study is needed to determine clinical outcomes over a long period.
In spite of early discovery of cancer and complete surgical resection, the rates of recurrence and mortality in lung cancer cases remain high [1]. During treatment of postoperative patients, it is especially important to detect early relapse and distant metastasis. Thus, it is crucial to develop useful biomarkers for predicting early recurrence and metastasis. Some recent studies have demonstrated that the presence of ITCs in circulating blood was useful as a biomarker for the prognosis of various types of disease, such as breast cancer [7], colorectal cancer [6], and lung cancer [11].
Various methods to detect and enrich ITCs have been reported. In older studies, ITCs were observed using whole blood samples [12], though those results are not considered to be reliable. Recently, Sienel et al. used a cytokeratin immunohistochemistry method to reveal that the presence of ITCs in samples collected from the PV of a pulmonary lobe containing lung cancer before resection was a predictor of poor survival [13]. In addition, Yamashita et al. reported a study that used reverse transcriptase-polymerase chain reaction (RT-PCR) assays of peripheral blood to detect messenger RNA (mRNA) of carcinoembryonic antigen (CEA), and noted that its presence was a prognostic indicator in patients with NSCLC [11]. Current advancement in technology allow for ITCs to be captured and quantitatively evaluated with the semi-automated CellSearch® System (Veridex LLC, NJ, USA). Using this system, some groups have reported that determination of the number of pre- and postoperative ITCs was useful as a biomarker for survival and prognosis [7,10], while another showed that those results were able to reveal cancer cell spreading caused by the operation [14]. However, most of those studies used quantitative evaluation methods and few have shown the significance of qualitative evaluation of ITCs.
In the present study, early relapse was associated with the presence of clustered cancer cells. We speculated that cancer cells may be able to live for a longer period in circulating blood when clustered, thus allowing direct access to distant organs and easy establishment of a secondary tumor. In addition, when considering tumor-initiating cells, it is possible that cells with a large diameter have a greater potential of causing relapse [15].
A recent study noted that epithelial–mesenchymal transition (EMT) plays important roles in cancer progression and metastasis [16]. Through the EMT process, the morphology and gene expression of the epithelial markers E-cadherin and cytokeratin become altered in cancer cells [17,18]. Our present analysis using immunohistochemical staining for cytokeratin showed that the positive rate of CS/BCS cases was 100%, whereas that in cases classified as S was low (33%). The reason for the different rates of positivity between S and CS/BCS cases may be related to EMT. Furthermore, the low occurrence of cytokeratin staining in cases classified as S may indicate that the expression of cytokeratin was reduced during the EMT process.
There are some limitations to our method, as accurate cell counting and ITC enrichment have not been perfected. On the other hand, with the CellSearch® system [7–10], cell-number counting and enrichment are easily performed in a semiautomatic fashion, though it is difficult to detect morphological features, in contrast to the method used in the present study. Moreover, there are problems with the procedure used to collect the samples. In the present method, in patients, after resecting the lung and placing it on the back table, blood was collected by aspiration from the PV, which had been stapled before the resection. Ideally, blood samples should be collected from the PV with proximal clamping before lung resection. In addition, it would be good to perform the assays with blood samples obtained from the peripheral vein. Some studies have presented preoperative and postoperative analyses of ITCs obtained from peripheral blood samples [7,11]. In the future, we intend to collect blood samples before lung resection and perform the assays using peripheral blood samples. In addition, we hope to conduct additional research using blood samples obtained at various time points before, during, and after surgery.
In conclusion, the present CD45-negative selection method was found useful to detect and enrich ITCs from blood samples obtained from the PV of lungs resected for NSCLC. In addition, our results show the clinical relevance of morphological classification of ITCs in NSCLC cases, as the presence of clustered ITCs was a prognostic indicator for patients following surgical resection.
Acknowledgments
The authors thank Professor Katsuyuki Aozasa, Dr Eiichi Morii, and Dr Hideo Yoshimura (Department of Pathology, Osaka University Graduate School of Medicine) for their contributions to the cytological diagnosis.
References
Author notes
Presented at the 24th Annual Meeting of the European Association for Cardio-thoracic Surgery, Geneva, Switzerland, September 11–15, 2010.
This research was supported by a Grant-in-Aid for Scientific Research (B) from the Japan Ministry of Education, Science, Sports and Culture, and the Uehara Memorial Foundation.
- radiation therapy
- churg-strauss syndrome
- immunohistochemistry
- lung
- carotid sinus syncope
- blood tests
- cancer
- chemotherapy regimen
- cd45 antigens
- non-small-cell lung carcinoma
- follow-up
- keratins
- preoperative care
- pulmonary veins
- lung volume reduction
- lung cancer
- tumor cells
- prognostic factors
- isolated tumor cells