-
PDF
- Split View
-
Views
-
Cite
Cite
Stephan Korom, Annette Boehler, Walter Weder, Immunosuppressive therapy in lung transplantation: state of the art, European Journal of Cardio-Thoracic Surgery, Volume 35, Issue 6, June 2009, Pages 1045–1055, https://doi.org/10.1016/j.ejcts.2009.02.035
- Share Icon Share
Summary
The coming of age of lung transplantation is accompanied by an immunosuppressive armamentarium that has been brought forward from other transplant indications. Widely employed on the basis of few small randomized studies, and mostly single-center experience or empirical expert knowledge, anti-rejection therapeutic strategies in pulmonary transplantation have hardly been rigorously evaluated in large-scale prospective international trials. This review compiles the available findings on the use of current immunosuppressants in clinical lung transplantation, accentuating high level-of-evidence study results. Reporting on recent meeting and registry data, and assembling ongoing relevant trials from international databases, this article serves as an update on the state of the art of immunosuppression in lung transplantation.
1 Introduction
The transplanted lung is an interface with the outside world. Comprising an alveolar surface area of 100 m2 and an air–blood diffusion barrier of 0.2 μm [1], the pulmonary parenchyma forms the largest and most direct area of exchange with the milieu extérieur in man. All particularities in treating lung allograft recipients reflect these unique circumstances. The perpetual exposure to infectious agents and allergen from the environment is paralleled by a continuous host immune surveillance from within. Consequently, both infection and rejection markedly contribute to patient attrition, arriving at twice the rates encountered in cardiac transplantation: infectious complications and chronic rejection at 3–5 years following engraftment account for 9.7% versus 19.5% and 16% versus 28.5% of deaths in heart and lung recipients, respectively [2,3]. The survival half life (based on the international ISHLT registry) after cardiac transplantation is 10, versus 5.2 years following pulmonary engraftment [2,3].
Immunosuppressive therapy in lung transplant recipients constantly navigates the narrow strait between Scylla and Charybdis, epitomized by rejection and infection. The required high load of immunosuppressants adds a cumulative risk, as illustrated by the risk for nephrotoxicity, bone marrow depression and malignancy over time. With approximately 2000 annual transplants worldwide, and the majority of centers performing <10 procedures per year, meaningful data from large randomized trials is only tenaciously obtained. To date no consensus has been reached concerning a standardized immunosuppressive regimen, and none of the contemporarily employed immunosuppressants in pulmonary transplantation (Table 1 ) have been approved for this indication by the FDA.
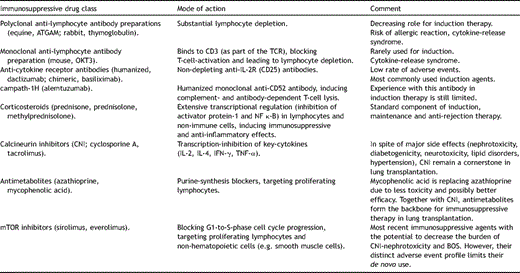
Currently employed immunosuppressive drugs in lung transplantation.
The focus of this manuscript is the current state of the art for pharmacological immunosuppression in lung transplantation, reflecting on available evidence from completed and ongoing clinical trials.
2 Induction
Induction therapy consists of a brief regimen of intravenous antibody therapy, targeting activated host lymphocytes. In 2008, around 54% of all pulmonary allograft recipients have been exposed to induction of some kind [3]. These anti-lymphocytic agents constitute a heterogenous class of drugs, ranging from polyclonal anti-T-cell preparations (ALG, ATG) to monoclonal agents, aimed at lymphocyte surface molecules such as CD3 (OKT3), IL-2R/CD25 (basiliximab, daclizumab) or CD52 (campath-1H). The use of polyclonal antibody preparations for induction has decreased to around 10%, while interleukin-2-receptor antagonists (IL-2RAs) are increasingly employed (around 37%); campath, a novel anti-CD52 monoclonal antibody, is used in around 6% of patients [3].
Polyclonal antibody preparations contain equine (ATGAM) or rabbit (Thymoglobulin) γ-globulin fractions, directed against human surface T- and B-cell-molecules [4]. When binding to lymphocytes, they induce complement-dependent cytolysis, resulting in substantial lymphocyte depletion [5]. Due to their xenogeneic nature, hypersensitivity responses may develop after repeated exposures. Leucopoenia and thrombocytopenia have to be monitored, and prophylactic anti-CMV treatment should be initiated [6]. Based on the analysis of ISHLT data, ATG/ALG-induction significantly reduced the incidence of acute rejection (AR) during the first year post-transplantation, compared to no induction or IL-2RA-induction [3]. These registry findings reconfirm a previous prospective, single-center study by Palmer et al. in 44 recipients of single or bilateral lung transplants, where the incidence of biopsy-proven acute rejection was significantly reduced in the presence of ATG-induction, versus no induction [7]. Yet, rate of survival, infection or malignancy at 2 years did not differ between these groups [7]. After 2 years, a trend toward a lower incidence of bronchiolitis obliterans syndrome (BOS) was documented in the ATG-group (20%) versus the non-induction arm (38%) [7].
Muromonab-CD3 (OKT3) is a mouse-anti-human-CD3 monoclonal antibody, which also acts by depleting human T-cells from the circulation. Since CD3 is part of the T-cell receptor complex, indispensable for lymphocyte activation, binding of OKT3 may trigger cytokine-release syndrome, with potentially life-threatening consequences [8]. Due to these side effects, use of OKT3 as induction agent is declining. Compared to ATG and daclizumab, induction with OKT3 did not lead to improved incidence of freedom from acute rejection or BOS at 2 years post-transplantation [9]. However, in this single-center, prospective study, rate of bacterial infection was significantly higher in the group that had been exposed to OKT3 [9].
In contrast to polyclonal antibody preparations and OKT3, the two IL-2RA basiliximab (simulect) and daclizumab (zenapax) show fewer side effects and are well tolerated. Both antibodies are murine in origin, but have undergone genetic engineering to replace part of the original amino acid sequences by human protein: basiliximab represents a chimerized (around 75% human protein), and daclizumab a humanized (around 90% human protein) antibody.
During the last decade, several trials have analyzed the impact of IL-2RA on acute rejection, infection, BOS and survival, compared to no induction and/or ATG and/or OKT3 (Table 2 ). Although some studies have been prospective in nature, they were limited by their small patient numbers and single-center approach [9–12]. Retrospective studies, albeit larger, were based on single-center data as well, mostly comparing historical findings with contemporary patient outcomes [13–15]. Taken together, and backed from the most recent ISHLT registry reports [3,16], evidence seems to be emerging that (i) induction therapy (either ALG/ATG or IL-2RA) is associated with significantly less rejection episodes than without induction; (ii) induction is associated with better long-term survival; (iii) induction treatment has no significant effect on freedom from BOS. As reflected in ongoing prospective trials (Table 3 : NCT00592306, NCT00188825, NCT00105183) there is a need to further clarify the role of induction regimen on the outcome of lung transplantation.
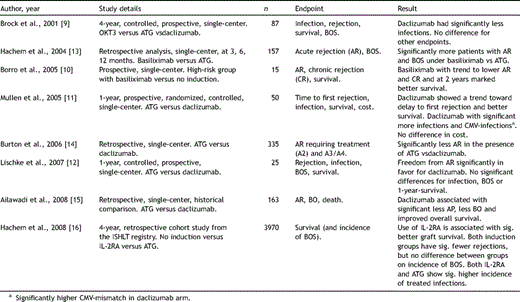
Key studies analyzing IL-2RA for induction therapy in lung transplantation.
Ongoing trials, assessing the use of pharmacological immunosuppressants in lung transplantation.
First data are available with campath-1H (alemtuzumab)-based induction in pulmonary transplant recipients [17]. However, these phase II results were obtained in a study design employing induction with campath-1H versus ATG, followed by tacrolimus near-monotherapy. At 6 months, there were significantly fewer acute rejections in the campath-1H group, compared to ATG [17].
3 Maintenance
In lung transplantation, maintenance immunosuppressive therapy is based in the majority of patients on a triple regimen [3],[18], composed of a calcineurin inhibitor (CNI; cyclosporine A or tacrolimus), an anti-metabolite (azathioprine, mycophenolate mofetil or enteric-coated mycophenolate sodium) and steroids. As a fourth group, mammalian target of rapamycin (mTOR) inhibitors (sirolimus, everolimus) may be introduced, often in substitution for the CNI or the anti-metabolite. In contrast to other perfused organ transplants, there is very limited experience on steroid withdrawal in lung allograft recipients [19,20]. Based on most recent data (time period 2002–2007), tacrolimus and mycophenolic acid were the most frequently used CNI and anti-metabolite, respectively. At 1 year, 77% of recipients were exposed to a CNI/anti-metabolite-combination, and 70% at 5 years [3].
3.1 Calcineurin inhibitors (CNI)
The introduction of cyclosporine A (CsA) in the early 1980s revolutionized clinical lung transplantation [21]. This cyclic fungal polypeptide binds to cytoplasmic cyclophilins, impairing the enzymatic activity of calcineurin, leading to a transcription-inhibition of key cytokines (IL-2, IL-4, INF-γ, TNF-α). The relatively low concentration of calcineurin in T-cells renders them especially sensitive to CsA [22]. A modified microemulsion formulation (Neoral; Novartis Pharma AG) has led to better bioavailability, achieving maximum blood levels more rapidly with less variability [23]. It has been shown that CsA trough levels correlate inadequately with the effective systemic exposure, yet, assessing concentrations at 2 h after intake (C2) better correspond with the effective pharmacokinetic profile [24]. Glanville et al. identified in 50 de novo lung transplant recipients (including 20 cystic fibrosis patients) target C2 levels, which were associated with improved rates of AR and BOS, compared to historic controls: C2 >800 μg/l at 48 h; 1200 μg/l in month 1; >1000 μg/l in month 2; > 800 μg/l in month 3; >700 μg/l month 3–6; > 600 μg/l beyond month 6 [25].
Recently, a randomized, placebo-controlled, double-blind, multicenter trial on efficacy of inhaled cyclosporine in lung transplant recipients under a conventional triple immunosuppressive regimen, was published [26]. Although the study had some limitations, and did not reach its primary efficacy endpoint (prevention of acute rejection), both survival and freedom from chronic rejection were significantly increased in the CsA arm, compared to placebo [26]. At the 2008 ISHLT meeting, the same group presented data on 30 transplanted patients, where aerosolized CsA in addition to conventional immunosuppression significantly preserved FEV1, versus placebo and historical controls [27]. The concept of selectively delivering an immunosuppressive agent to the allograft is intriguing, and may hold future promise to circumvent systemic side effects associated with the class of drugs. The increasing interest in this approach is underscored by two current ongoing studies (Table 3: NCT00633373, NCT00378677).
Tacrolimus (Tac, synonymous with FK506; Prograf, Astellas Pharma, Inc.), a macrolide antibiotic, was adopted as an immunosuppressant in transplantation medicine in the early 1990s. In analogy to CsA it functions as a CNI, however, through binding to a distinct immunophilin [28]. In vitro, Tac displays a 50–100 times greater immunosuppressive potency than CsA [22]. In contrast to CsA, Tac is dosed according to trough (C0) levels, and bioavailability varies between patients. Recently, Tac has been introduced as a once daily formulation (advagraf) in Europe and Canada for the prophylaxis of acute rejection in liver and kidney transplant recipients [29], yet, so far, no published data exists on use of this formulation in lung transplantation. Robust study data comparing efficacy of Tac versus CsA in pulmonary engraftment is scarce, and to date, only three prospectively designed trials have been reported [30–32] (Table 4 ). Two of them were based on single- or two-center experience, over 1 or 2 years, enrolling 74 and 133 patients. Only one large international multicenter study has been performed, with 3-year results presented at the 2008 ISHLT meeting [32]. Emerging from these investigations, there seems to be a trend of fewer AR episodes in the presence of Tac, compared to CsA. Likewise, ISHLT registry data between 2000 and 2005 indicated a slightly lower average number of AR per year in patients under Tac/mycophenolate mofetil (MMF), versus CsA/MMF [3]. However, throughout all studies there was no significant difference seen in terms of survival between groups [30–32], and only one trial showed a clear advantage of Tac over CsA for development of BOS at 2 years [30]. Furthermore, it has to be taken into account that in all trials, CsA dosing was based on C0, instead of C2 levels, which may have limited achieving the optimal individual systemic exposure [25]. Therefore, to accurately investigate state-of-the art immunosuppressive potential of CNIs, there is a need for large, randomized, multicenter trials, comparing CsA C2-monitoring versus Tac standard and once daily formulation.

Key studies analyzing efficacy of CsA versus Tac in lung transplantation.
Altogether, short- and long-term immunosuppressive efficacy as an unambiguous primary endpoint, when discussing CsA and Tac in recipients of lung allografts, may not be the adequate measure. Alternatively, the characteristic side effect profile for the compounds should be considered. Nephrotoxicity is similar with both drugs [22], but CsA causes less new-onset diabetes, neurotoxicity and gastrointestinal complications [22,31]. Tac is associated with fewer lipid metabolism disorders, less hypertension, and does not cause hirsutism and gingival hyperplasia [22]. Based on an individualized approach, Tac may thus be preferred in recipients with cardiovascular risk factors, whereas in patients with a history of diabetes mellitus, CsA would be the CNI of choice. This rationale is supported by ISHLT registry data, listing hypertension (85.3%), hyperlipidemia (53.6%) and diabetes mellitus (35.5%) among the most prevalent morbidities at 5 years following lung transplantation [3]. Furthermore, pre-existing diabetes mellitus has been identified as a categoric risk factor (RR 1.15; p = 0.00489) for 5-year mortality [3]. Revisiting the paradigm of immunosuppressive potency, greater significance should be attributed to recipient profiles, choosing CNI based on individual risk factors and comorbidities.
3.2 Anti-metabolites
Azathioprine (Aza), initially used to prevent rejection in recipients of kidney allografts in the 1960s by Murray and co-workers [33], signified the first breakthrough in immunosuppressive therapy and prepared the ground for clinical expansion of transplantation medicine. 6-Mercaptopurine, the active metabolite of Aza, inhibits de novo purine and DNA/RNA synthesis, and T-cell proliferation pathways [34]. After the initiation of successful lung transplantation by Cooper et al. in the 1980s [35], Aza, together with CsA and steroids formed the therapeutic backbone of immune modulation in these patients.
Mycophenolate mofetil (cellcept, Roche AG) has been used in experimental transplantation since the late 1980s [36], and in the clinical setting from the early 1990s on [37]. Mycophenolic acid (MPA), the active compound of MMF, displays a more selective and less toxic effect, compared to Aza. As a reversible inhibitor of inosine monophosphate dehydrogenase (IMPDH), MPA impairs the rate-limiting enzyme in de novo purine synthesis [38]. In contrast to other blood cells that can utilize the salvage purine synthesis pathway, proliferating lymphocytes rely heavily on de novo synthesis. Thus, the key effector component responsible for cellular and humoral immunity is selectively inhibited [22]. Large prospective randomized trials in other perfused organ transplants confirmed the superior efficacy of MMF over Aza in the late 1990s [39,40], and several smaller studies from single centers versus historical controls, indicated a similar trend in de novo lung transplantation [41–44]. However, only two randomized prospective trials have been undertaken to elucidate the value of MMF in pulmonary transplantation (Table 5 ): Palmer et al., enrolling 81 participants, in a 6-month, two-center trial did not find a significant difference in biopsy-proven acute rejection, incidence of CMV infection or survival, between MMF versus Aza [45]. Likewise, in the only international multicenter study, in a follow-up over 3 years in 320 patients, McNeil et al. [46] did not show a significant difference between these two anti-metabolites for incidences of acute rejection episodes, BOS or for survival. Concluding from the available study data, and despite its increasing use [3], the unambiguous proof of the superiority of MMF over Aza in lung transplantation is still outstanding.
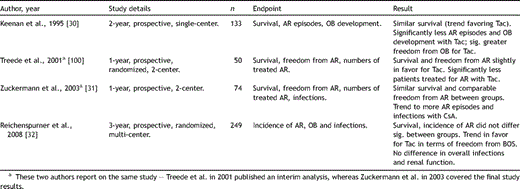
Key studies analyzing efficacy of Aza versus MMF in lung transplantation.
When evaluating the immunosuppressive efficacy of MMF in the context of rejection prophylaxis in pulmonary engraftment, drug–drug interactions with CNI have to be taken into consideration. Since CsA impairs the enterohepatic recirculation for MPA, MPA concentrations are lower in recipients receiving CsA, compared to patients on Tac-based regimens [47]. Gerbase et al. recently showed in maintenance lung transplant recipients that MPA trough levels decreased by half when MMF was combined with CsA [48]. Optimizing future immunosuppression in lung transplantation, more effort will have to be directed toward therapeutic drug monitoring of MMF [49].
Myelosuppression and gastrointestinal adverse events are the most common encountered side effects of MMF, often necessitating dose reduction or even temporary discontinuation early after transplantation. Especially in the presence of intensified anti-CMV or antibiotic therapy, developing leucopenia may force treating physicians to lower MMF exposure, thus compromising on net immunosuppressive potency. Enteric-coating of MPA (EC-MPS; myfortic, Novartis Pharma AG) has been introduced to improve tolerability by decreasing upper gastrointestinal side effects, while maintaining equivalent MPA exposure and maximal concentration to MMF [50]. Approved in Europe and the USA in 2004, EC-MPS has been shown to be equally effective to MMF in de novo renal transplantation [51], and to be safely used for converting renal maintenance patients from MMF [52]. Study results reporting on EC-MPS in lung transplant recipients have not been published so far. However, in a currently ongoing international, prospective, randomized trial (Table 3; ACTRN12605000141640) EC-MPS is being compared to everolimus to assess incidence of BOS over a time period of 3 years in recipients of pulmonary allografts. Although a MMF treatment group was not planned to be included in this study, more data on immunosuppressive efficacy, safety and tolerability of the enteric formulation in de novo patients following lung transplantation may be expected.
3.3 mTOR inhibitors
This class of drugs signify the most recent pillar in immunosuppressive therapy employed in pulmonary transplantation. Rapamycin (sirolimus; Rapammune, Wyeth-Ayerst), intensely studied in the 1990s, was approved in the US and Europe in 1999 and 2000, respectively. Binding to FKBP12 (FK-binding protein 12; interestingly this immunophilin also serves as a binding site to Tac, but Tac/FKBP12 targets calcineurin-phosphatase), Rapamycin/FKBP12 then inhibits a 289-kDa-kinase, mammalian target of rapamycin (mTOR), eventually blocking G1- to S-phase cell cycle progression [53]. By interfering with DNA replication at such an early stage, rapamycin exerts a profound antiproliferative effect in hematopoietic and non-hematopoietic tissues. Proliferation of activated T-cells is arrested in the late G1 phase [54],[55], IL-2-dependent and IL-2-independent stimulation of B-cells is blocked [56] and differentiation into antibody-producing clones abrogated [57]. In addition, this class of drugs inhibit smooth muscle cell proliferation [58], thereby ameliorating long-term graft-specific histological changes such as bronchiolitis obliterans [59]. The promise to curb the dynamics of chronic rejection fuelled an interest early on to use rapamycin in de novo lung transplant recipients. Regrettably, in two single-center pilot studies, out of 19 recipients treated perioperatively with rapamycin, 7 experienced major airway anastomotic complications (5 with partial or complete bronchial dehiscence), and 5 patients died [60,61]. Findings from heart [62], kidney [63], and liver [64] transplantation trials on de novo employment of rapamycin indicated similar problems. Therefore, use of the drug shifted from first-line treatment to ‘reserve’ immunosuppression in maintenance patients suffering from particular problems. Calcineurin inhibitor-associated nephrotoxicity has been alleviated by minimization/discontinuation of CNI following the introduction of rapamycin in several smaller studies [65–67]. Bronchiolitis obliterans, developing in maintenance patients, has been targeted with mixed success in some single-/double-center trials [59,68,69]: although individual responses were observed across cohorts, small numbers, heterogeneity of enrolled patients and varying adjunctive immunosuppressive treatments limit the significance of these results.
At the 2008 ISHLT Meeting, Bhorade et al. presented data from the AIRSAC trial, a US, multi-center, randomized and prospective investigation, assessing sirolimus versus azathioprine (both with Tac and steroids) in 181 lung transplant recipients [70]. To avoid the potential for wound healing complications, patients were randomized at 3 months post-transplantation. At 12 months, the incidence of AR did not differ significantly between groups [70]. The study was limited by a discontinuation rate of 66% in the sirolimus arm (vs 47% in Aza) and an imbalance in terms of pre-transplant diagnosis (more patients suffering from fibrosis and CF in the sirolimus arm). Interestingly, there were significantly more overall infections in the presence of sirolimus, yet, the incidence of CMV infection was significantly lower than under Aza [70].
Hyperlipidemia, cutaneous rash, oral ulcers, anemia, thrombocytopenia, edema, hemolytic uremic syndrome and delayed wound healing are side effects associated with rapamycin. Of note, pulmonary toxicity has been reported in kidney, liver and cardiac transplant recipients, presenting as interstitial pneumonitis, lymphocytic alveolitis, BOOP or alveolar hemorrhage [71]. Only a few cases of sirolimus-associated pneumonitis have been reported in lung transplant patients, yet, since it affects the allograft, arriving at the appropriate differential diagnosis may constitute a challenge [72,73]. Most of the described patients improved without sequelae after discontinuation of the drug, which indicates an allergic/toxic, probably T-cell mediated etiology [74]. In addition, overdosing may play a role, since increased sirolimus troughs have been detected in patients developing pulmonary toxicity [72,74].
Everolimus (certican, Novartis Pharma AG) is a more recently introduced mTOR inhibitor, which is distinguished from rapamycin by a hydroxyl group at position C40. Approved in Europe in 2004, this proliferation signal inhibitor displays a modified bioavailability, due to its shorter half life of 28 h (rapamycin: 62 h) and greater polarity [75,76], as compared to rapamycin. Although the adverse event profile overlaps with sirolimus, emerging data in de novo cardiac transplantation indicate fewer wound healing events in the presence of everolimus [77]. There are less reports on pulmonary toxicity-associated events relating to everolimus [78], and even switching patients from rapamycin to everolimus for pneumonitis has been shown to alleviate symptoms [79].
In an international, randomized multicenter study enrolling 213 BOS-free lung transplant recipients, efficacy was evaluated between azathioprine and everolimus [80]. Although at 12 months the everolimus group showed a significantly smaller decline in FEV1 and had experienced less acute rejections, at 24 months only the incidence of acute rejection episodes still differed significantly between arms [80]. In addition, there is an ongoing study (NOCTET study), investigating whether initiation of everolimus together with reduction of CNI in maintenance heart or lung transplant patients with renal impairment will improve renal function (Table 3; NCT00377962). At the 2008 ISHLT meeting, Strueber et al. presented their first year interim analysis on delayed de novo treatment of lung allograft recipients, evaluating everolimus versus standard of care MMF (with CsA and steroids) in 100 patients. In the interim analysis, there was a significant (p=0.043) survival benefit in favor of the everolimus group. As in the study results presented by Bhorade et al. [70], there were markedly less CMV infections in the everolimus arm, compared to MMF [81]. A similar designed European-Australian trial is currently under way, with a follow-up of 3 years and incidence of BOS as primary endpoint (Table 3: ACTRN12605000141640).
3.4 Corticosteroids
Corticosteroids are employed during induction, maintenance and anti-rejection therapy in pulmonary transplantation, emphasizing their crucial role in modulating the host immune response. They suppress prostaglandin synthesis, reduce histamine/bradykinin release, decrease vascular permeability and down-regulate key cytokines by influencing gene transcription [82]. Used systemically just prior to re-perfusion of the implanted lung, they are then rapidly tapered in the early postoperative period. In clinical practice, steroid withdrawal in pulmonary transplant recipients is rarely undertaken [19,20], reflecting the increased immunogenicity of the allograft, as compared to kidney or heart. Impaired glucose tolerance, psychological disorders, acne, hirsutism and M. Cushing are common side effects, and glucocorticoids significantly contribute to bone loss following transplantation, with most of the damage occurring in the first 12 months, when doses are highest. The prevalence of osteoporosis among lung transplant patients is 73%, and fracture rates between 18 and 37% have been reported [83].
Inhaled steroids have been investigated as adjunctive local immunosuppressive treatment in lung transplantation. Although reports on single patient outcome were favorable [84,85], in a randomized, double-blind study on thirty stable pulmonary transplant patients, no effect on BOS or survival was seen [86].
4 Acute rejection
Based on the ISHLT registry data, in the first year, 27–40% of all lung allograft recipients transplanted between July 2004 and June 2007 were treated for acute rejection, compared to 40–51% of patients receiving their transplants between January 2000 and June 2005 [3]. Improved diagnosis and treatment has further decreased the risk of death from AR from 4.3% within the first 30 days post-transplantation to 1.8% at 1 year [3]. Although multiple non-immunologic injuries may contribute to development of BOS, the incidence of AR episodes constitutes one of the major risk factors [87].
Intravenous steroid pulses (500 mg to 1000 mg/d) for 3 (to 5) days, followed by a temporary increase in maintenance doses for a few weeks, are the preferred treatment. In the majority of cases, AR can be reversed, as monitored in subsequent surveillance bronchoscopies. For refractory AR, various agents have been used, including polyclonal/monoclonal antibodies [88], inhaled cyclosporine [89], and methotrexate [90]. Recurrent AR, representing persistent, inadequate control of the host immune response, has been targeted successfully by switching-strategies, e.g. from CsA to tacrolimus [91], or by adding a mTOR inhibitor.
5 Chronic rejection
Chronic rejection in pulmonary transplantation is synonymous with BOS, and at times the diagnosis of BOS moves into the therapeutic focus in patients with a history of recurrent/steroid-refractory AR episodes. Often, though, BO develops insidiously, in recipients with an uneventful clinical course following lung engraftment. By 5.6 years post-transplantation, 51% of patients will have developed BOS, which in turn accounts for 19.2% of all deaths in patients that survive between 3 and 5 years [3].
When the diagnosis of BOS is established, the current therapeutic regimen has to be re-evaluated; modifying pharmacological immunosuppressive therapy may be pivotal, but photopheresis [92,93], gastroesophageal reflux [94] and non-compliance [95] should also be considered. Focusing on the pharmacological side, augmentation of existing regimens, switching within classes of drugs and/or substitution of one class for another are conceptual. Steroids may be transiently increased, and ATG has been advocated [96]. Within-class switches comprise mainly of CsA to Tac and Aza to MMF [97]. Especially mTOR inhibitors have been used in several single-center programs to stabilize lung function after the diagnosis of BOS [59,65].
Recently, the macrolide antibiotic azithromycin has shown efficacy in improving FEV1 in lung transplant recipients suffering from BOS. Although a distinct mode of action has yet to be elucidated, neutrophilia, chemokine release and bacterial exacerbations have been down-regulated in the presence of azithromycin [98].
Given the impact of chronic rejection on quality of life and recipient survival following lung transplantation, the need for international, randomized and prospective trials, evaluating therapeutic regimens for established BOS is obvious.
6 Conclusion
Advances in surgical technique, perioperative management and anti-infectious strategy have markedly improved early morbidity and mortality in recipients of pulmonary allografts. Yet, during the last 15 years, long-term survival has failed to increase accordingly [3,99]. The widespread acceptance of Tac and MPA in lung transplantation occurred without robust clinical trial evidence, and neither one of these compounds has unequivocally been proven superior to their predecessors. Novel as a class, mTOR inhibitors have so far not shown higher immunosuppressive efficacy or better containment of emerging BOS, however, several large-scale prospective studies are currently ongoing. Due to the pronounced immunogenicity of the pulmonary parenchyma, side effects of the required high load of immunosuppressants are considerable, and the ensuing disposition for infectious complications substantially increases morbidity. In spite of the low annual frequency of procedures performed, distributed across a high number of centers worldwide, efforts to better co-operate and converge on study design should be undertaken. Only with the help of large, international, randomized and prospective trials will it be possible to truly benchmark future immunosuppressive strategies against contemporary practices.
This article is dedicated to Professor emeritus Harald Morr.