-
PDF
- Split View
-
Views
-
Cite
Cite
Francesco Petrella, Pasquale Chieco, Piergiorgio Solli, Giulia Veronesi, Alessandro Borri, Domenico Galetta, Roberto Gasparri, Lorenzo Spaggiari, Which factors affect pulmonary function after lung metastasectomy?, European Journal of Cardio-Thoracic Surgery, Volume 35, Issue 5, May 2009, Pages 792–796, https://doi.org/10.1016/j.ejcts.2009.01.011
- Share Icon Share
Abstract
Background: Pulmonary metastasectomy is an accepted procedure in selected patients, very often requiring multiple non-anatomical resections. Although it is intuitive that functional loss is proportional to the number and extent of pulmonary resections, this link has never been proved and is the hypothesis behind this study. Methods: We retrospectively reviewed pulmonary function changes after lung metastasectomy. Baseline and postoperative spirometric values were evaluated and their changes were correlated to (a) number of resections, (b) extent of resections and (c) intervals between surgery. Results: Sixty-six patients were enrolled (31 men, mean age 56 years, range: 23–75); mean interval between surgery: 54.5 days; mean extent of resection: 11.45 cm; mean number of resections: 3. Preoperative mean spirometric values were: FEV1 2.73 l (97.75%); FVC 3.11 l (95.50%); DLCO/AV 1.21 l (99.80%). Mean changes in FEV1, FCV and DLCO/AV were −13.4%, −12.4% and +1.2% respectively. Patients receiving three or more non-anatomical resections had functional loss similar to those undergoing lobectomy. The extent of total resection (>11 cm, p < 0.05) and the interval between surgery (>90 days, p < 0.0001) influenced FEV1 and FVC modifications. At three months none of these functional modifications remained. Sex, age, side of the operation and histology of primary tumor did not affect spirometric changes. Conclusions: Spirometric changes after pulmonary metastasectomy are affected by total volume lung parenchyma resected within the first 90 days. Functional loss after three or more non-anatomical resections is comparable to that recorded after lobectomy.
1 Introduction
Pulmonary metastasectomy is now a widely accepted procedure in selected patients, depending on the location, number and volume of the lesions, primary tumor histology and the disease-free interval [1,2].
The first series of bilateral metastasectomies were reported during the 1970s with good results. However lung resection for metastasis is often performed for single or unilateral lesions and redo procedures are quite rare, also due to the strict selection criteria adopted [3]. This is probably the main reason for the lack of information on functional assessment in patients undergoing multiple and redo, very often non-anatomical lung resection, for pulmonary metastases.
Studies on standard lobectomy for non-small cell lung cancer (NSCLC) have yielded much information on lung function, largely by comparison of spirometric values before and after surgery. However, there are very few well established spirometric variables for multiple or bilateral metastasectomy candidates.
In addition the cohort of patients requiring lung metastasectomy differs widely from that requiring standard NSCLC surgery: patients are usually younger, non-smokers and without chronic obstructive pulmonary disease (COPD). Anatomical resections (lobectomy, bilobectomy or pneumonectomy) are avoided in these patients in favor of parenchyma-sparing procedures (wedge resection, segmentectomy or nodulectomy) whenever possible.
The aim of the present study was to analyze changes in standardized spirometric values in patients undergoing pulmonary metastasectomies, to gauge the change in lung volume and diffusing capacity after metastasectomy and their relation to lung volume resection, number of resections and time interval between redo procedures.
2 Methods
We retrospectively reviewed pulmonary function changes after lung metastasectomy. Baseline and postoperative spirometric values were evaluated and their changes correlated to (a) number of resections (number of resected specimens), (b) extent of resections (total amount of resected tissue calculated on the major axis of all the resected specimens) and (c) intervals between surgery (days between the first and the second operation).
We studied a group of patients submitted to ipsilateral or controlateral redo procedures. For preoperative assessment before the second surgical procedure, the patients underwent a second spirometry test whose values were compared with the baseline spirometry test performed before the first procedure, thus calculating the difference (%) between the selected values (FEV1, FVC and DLCO/VA).
The cohort comprised (a) candidates for bilateral procedures in the first instance, and thus having a standard short interval between surgical procedures (about 30 days) and (b) patients undergoing redo metastasectomies because of a new metastasis in the same or other lung disclosed during the follow-up period and thus having a varying and longer interval between surgical procedures than the other group.
None of the patients underwent adjuvant therapy (chemotherapy or radiotherapy) between the two operations. In addition, only patients submitted to pure parenchyma resections were considered eligible for the study, thereby excluding patients submitted to concomitant diaphragm or chest wall resections that may have affected spirometric changes (Table 1 ).
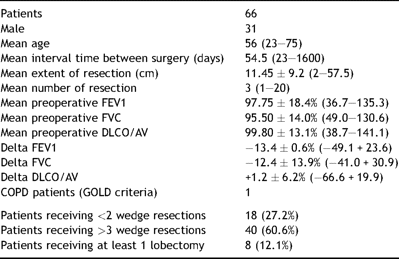
All patients were operated on via standard lateral muscle sparing thoracotomy (except three patients operated on both sides at the same time by median sternotomy and thus excluded from statistical analysis) thereby avoiding spirometric changes due to surgical incisions.
All patients received physiotherapy instructions on the day before surgery and were enrolled in the standard postoperative physiotherapy program depending on the operation (basically volume incentivation, forced expiratory flow and cough). Forced expiratory flow was interrupted or avoided if intense early air leakage was detected. The standard analgesic protocol was routinely applied in all patients.
Spirometry tests were done using a Jaeger Master Screen Pneumo spirometer according to the criteria of the American Thoracic Society. The results of spirometry were collected after bronchodilatator administration. Carbon monoxide lung diffusion capacity was measured by the single breath method. FEV1 and DLCO were expressed as percentage of predicted value for age, gender and height according to the European Community for Steel and Coal Prediction equations [4].
3 Statistical analysis
The aim of the statistical analysis was to test whether the extent of total resection taken as a binary variable (< or >11 cm) and the interval between surgery also taken as a binary variable (< or >90 days) affects FEV1, FVC and DLCO/VA.
Only differences between second and first assessment were used.
We used unpaired t-test and factorial ANOVA. These analyses were done with StatView 5.0 statistical software (SAS Institute, Cary, NC, USA).
4 Results
Sixty-six patients submitted to redo pulmonary metastasectomies were enrolled in the study (31 men, mean age 56 years, range: 23–75). Mean interval time between surgery was 54.5 days and the mean extent of resection was 11.45 cm, calculated on the major axis of every single resected specimen; mean number of resections was three. Thirty-one patients underwent ipsilateral procedure; 35 patients were scheduled for bilateral procedure in first instance.
Eighteen patients received one or two wedge resections; 40 patients received three or more wedge resections or typical segmentectomies; 8 patients received at least a lobectomy with or without further resections.
Preoperative mean spirometry values were: FEV1 2.73 (97.75%), FVC 3.11 (95.50%), DLCO/AV 1.21 (99.80%). Mean changes in FEV1, FCV and DLCO/AV after the first metastasectomy were −13.4%, −12.4% and +1.2%, respectively.
According to GOLD criteria [4] (FEV1 <80% predicted and FEV1/FVC ratio <0.7) only one of the 66 patients had chronic obstructive pulmonary disease (COPD).
The histology of the primary lesion was: gynecologic tumors in 17 patients; colonic tumors in 18; breast tumors in two; melanoma in one; osteosarcoma in three; respiratory tumors in two; teratoma in one; soft tissue sarcoma in nine; head and neck tumors in three; thymic tumor in one; urinary tract tumors in nine (Table 2 ).
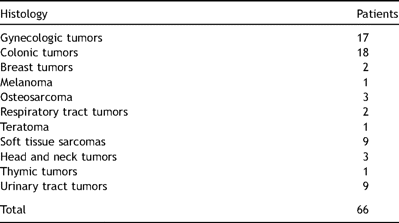
Only patients submitted to standard pulmonary typical or atypical resections were enrolled (lobectomy, typical and atypical segmentectomy, tumorectomy) while patients undergoing laser or argon resection were excluded in order to avoid functional discrepancy due to the different resection technique.
Patients receiving three or more peripheral non-anatomical resections, whose global extension was bigger than 11 cm, had functional loss similar to those undergoing lobectomy, performed because of the presence of multiple or single but centrally located lesions, not amenable to parenchyma sparing procedures.
When single variables were examined with a t-test, both the extent of total resection (>11 cm, p < 0.05) and the interval between surgery (>90 days, p < 0.0001) influenced FEV1 and FVC modifications. When both variables were introduced in a factorial ANOVA analysis, only the interval between surgery was statistically significant for FEV1 and FVC (p < 0.0001 for both). Interactions between the two variables were not present.
The extent of total resection (>11 cm, p < 0.05) and the interval between surgery (>90 days, p < 0.0001) influenced FEV1 and FVC changes. Effect sizes are depicted in box plots of Fig. 1 where the y-axis differences between the median lines inside the boxes can be easily calculated (Fig. 1).
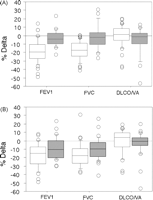
Box-plot graphic display of % differences in FEV1, FVC and DLCO/VA. (A) According to interval between surgery, <90 days = white box, >90 days = grey box. (B) According to extent of total resection <11 cm days = white box, >11 cm = grey box. The three horizontal bars in the box from top to bottom are the 75th, 50th (median) and 25th percentiles, respectively. The upper line ends at the 90 percentile and the lower line at the 10th percentile. Outliers are represented by circles.
At three months none of these functional changes remained. Sex, age, side of the operation and primary tumor histology did not affect spirometric changes.
5 Discussion
The lung is the most common site of metastases for all malignancies except those developing in the area of portal venous drainage [1–3].
In the past pulmonary metastasectomy was contraindicated because metastases were considered as an untreatable disseminated disease. In the modern era of surgery the intentional resection of a pulmonary metastasis gained some favor thanks to the pioneering work of Barney and Churchill in 1939 [5] and Alezander and Haight in 1947 [6] until more recent series published good results even in patients with multiple bilateral metastases [7–9] (Fig. 2 ).
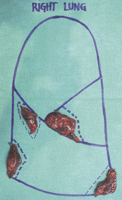
Many pulmonary function tests (PFTs) have been used to evaluate patients undergoing lung resection. Most of them emphasize the importance of preoperative forced expiratory volume in 1 s (FEV1), FEV1/forced vital capacity and transfer factor of the lung for carbon monoxide (TLCO)[10]. The 6 min walking test, Master test, maximum oxygen consumption per unit time test and prediction of postoperative FEV1 by different formulas are also used to evaluate postoperative risk [10]. We opted for the easiest and most reproducible values obtained by simple standard spirometry, also because spirometry results are not conditioned by extrapulmonary factors such as muscular fatigue (e.g. during stair climbing test or tread mill test).
A second spirometry test was done to reassess patients before the second surgical procedure and values were compared with the baseline spirometry test performed before the first procedure. On the one hand this prevented us having the spirometric control at the same time after the first surgery, thus constituting a lack of information due to the absence of temporal homogeneity. On the other hand it offered us the chance to stratify our population by time and obtain at least two different groups (<90 days >90 days interval between surgery) thus evaluating the effect of time on pulmonary recovery.
Some patients received a third spirometry as they were further scheduled for a third redo metastasectomy; considering that just few of them underwent this further assessment and, among them, many received chemotherapy interfering with DLCO/VA values, we decided not to investigate these additional values.
The significant FEV1 and FVC changes observed in the group undergoing redo surgery before the 90th day may be due to impaired chest wall compliance, accumulated bronchial secretions, bronchial hyper reactivity, persistent zone of microatelectasis, increased lung water, diaphragm dysfunction and reduced surfactant activity [11].
Our spirometric measurements obtained at rest were similar to those of Wang et al. who evaluated the effect of lung resection on lung function and exercise capacity values, including DLCO, during exercise, demonstrating a significant reduction in FEV1, FVC and DLCO (without correction for alveolar volume) of 12%, 13% and 22% [12]. Similar results had already been obtained by pulmonary static function tests and after exercise in lung cancer patients undergoing pulmonary resection [13,14].
We stopped using median sternotomy for one stage operation because the surgical field may be insufficient, mainly in case of lesions located in the posterior part of the lower lobes, in particular on the left side. We would rather avoid one-step operations by two simultaneous lateral thoracotomies because many patients would not tolerate the global parenchyma extent of resection; moreover the short time between the two planned bilateral resection (never longer than 30 days) has never taken into account the oncological growth of the target lesions, as confirmed by the second staging CT scan.
As already demonstrated in standard lobectomy for NSCLC, pulmonary resection may improve lung function and hence spirometric values, namely FEV1 [15]. This outcome may be attributed to the lung volume reduction effect of the lobectomy (removal of bullous shunting parenchyma well vascularized but not ventilated) and the reduction of the baseline hyperinsufflation normally presents among COPD patients. In addition, pulmonary resection of extra-anatomical respiratory dead space, caused by direct pulmonary artery infiltration from the neoplasm, may improve pulmonary function. Lastly, improved airway calibre and elastic recoil have been demonstrated after resection [15].
All these favorable results may be obtained in COPD patients. As only one patient in our cohort was classified as COPD, this may explain why we did not observe any FEV1 improvement but a consistent FEV deterioration after surgery (−13.4%). On the contrary, while carbon monoxide lung diffusion capacity/AV often deteriorates after NSCLC resection mainly because of induction chemotherapy [16], DLCO/AV presented a +1.2% improvement in our series. This new finding may be due to the absence of inductive chemotherapy damaging alveolar membrane in our series. The fact that membrane diffusing capacity remains stable after metastasectomy while capillary volume declines after pulmonary resection may explain the DLCO/VC increase in our series. Moreover it is well known that diffusing capacity is composed of membrane diffusing capacity (DMCO) and pulmonary capillary volume (Vc), and that DLCO increases in relation to cardiac output. As none of our patients underwent adjuvant therapy between the two operations, spirometric changes were basically due to the resections performed rather than other factors like other intercurrent therapies.
The diffusion capacity of the lung for carbon monoxide (DLCO), also known as the transfer factor (TL) is commonly recognized as an indicator of the gas exchange function of the lungs [16]. The DLCO is usually reported as an absolute number (DLCO) and as a value divided by alveolar volume (AV), also known as the carbon monoxide transfer coefficient (KCO) [17]. Since the DLCO/AV appears to account for differences in lung size, it might represent a more accurate expression of the intrinsic gas exchange function of the lung [16]. Johnson demonstrated that unadjusted DLCO and KCO for AV percent predicted values showed wide differences and variability, and hence may be misleading. Changes in DLCO and KCO with alveolar volume are relevant for accurate interpretation of diffusion in patients with low lung volumes. Adjusting predicted DLCO and KCO for alveolar volume provides a better assessment of lung function [18].
Even commonly accepted functional evaluation guidelines recommend limiting DLCO measurement only in patients with a FEV1 lower than 80% of the predicted value [19,20]. We measured DLCO and then DLCO/AV in all patients able to maintain the apnea for the adequate time, as required by the standard execution measurement technique because FEV1 and DLCO reflect the status of two different components of pulmonary function (airflow and gas exchange) not necessarily correlated to each other [21].
Moreover Takeda recently demonstrated that to predict pulmonary morbidity predicted postoperative %DLCO is more important than static pulmonary function (like predicted postoperative %VC or %FEV1) for patients undergoing pulmonary resection after induction therapy. The decrease in DLCO is thought to reflect a limited gas exchange reserve caused by the potential toxicity of chemotherapy or chemoradiotherapy [22]. No worsening of DLCO was encountered in our series because none of the selected patients underwent chemotherapy or radiotherapy.
In conclusion, spirometric changes after pulmonary metastasectomy are affected by total volume lung parenchyma resected within the first 90 days. Functional loss after three or more non-anatomical resections is comparable with that recorded after lobectomy. Standard spirometry performed according to the criteria of the American Thoracic Society, including carbon monoxide lung diffusion capacity measured by the single breath method, is an adequate pulmonary function test in patients undergoing redo metastasectomies. These findings offer the thoracic surgeon valuable information on the indications for redo metastasectomy and intraoperative surgical strategy.
Presented at the 22nd Annual Meeting of the European Association for Cardio-thoracic Surgery, Lisbon, Portugal, September 14–17, 2008.
Acknowledgement
Anne Collins edited the English text.
Abbreviations
- FEV1
forced expiratory volume in 1 s
- FVC
forced vital capacity
- DLCO
carbon monoxide lung diffusion capacity
- NSCLC
non-small cell lung cancer
- KCO
carbon monoxide transfer coefficient
- TL
transfer factor
- AV
alveolar volume
- PFTs
pulmonary function tests