-
PDF
- Split View
-
Views
-
Cite
Cite
Makoto Hamaishi, Kazumasa Orihashi, Shinya Takahashi, Mitsuhiro Isaka, Kenji Okada, Taijiro Sueda, Transcranial motor-evoked potentials following intra-aortic cold blood infusion facilitates detection of critical supplying artery of spinal cord, European Journal of Cardio-Thoracic Surgery, Volume 33, Issue 4, April 2008, Pages 695–699, https://doi.org/10.1016/j.ejcts.2008.01.003
- Share Icon Share
Abstract
Objective: In order to determine whether critical intercostal artery is present in the aneurysm during descending thoracic or thoracoabdominal aortic surgery, changes of transcranial motor-evoked potentials (Tc-MEPs) were monitored following infusion of cold blood into the aorta as an adjunct ‘on-site assessment’. Accuracy of this method was evaluated. Methods: Fourteen patients were examined for Tc-MEPs changes following infusion of cold blood (4 °C, 300–450 ml) into the aneurysm. The intercostal arteries in the aneurysm were reconstructed when the Tc-MEPs amplitude decreased to below 50% of the baseline within 3 min after cold blood infusion. When the amplitude did not decrease, every intercostal artery in the aneurysm was ligated. Results: The Tc-MEPs amplitude did not decrease in eight cases (57%), while it decreased in six cases (43%). In the former, no case presented with paraplegia despite every intercostal artery being ligated. In the latter, the amplitude recovered after reconstruction in four patients, who had no paraplegia postoperatively. In the remaining two cases, however, the amplitude did not recover: one died of multiple organ failure with postoperative assessment unfeasible; the other developed paraplegia following surgery. Except one case with operative death, both sensitivity and specificity of our criteria with cold blood infusion was 100% in this series. Conclusions: Cold blood infusion into the clamped segment of aorta accelerates Tc-MEPs changes and can possibly reduce ischemic insults of spinal cord during diagnostic process, while it accurately detects presence of critical intercostal artery in the segment. This method appears to be promising adjunct on-site assessment.
1 Introduction
To prevent spinal cord injury following descending thoracic or thoracoabdominal aortic surgery, various methods have been used, including distal aortic perfusion, reimplantation of intercostal or lumbar arteries, systemic hypothermia, cerebrospinal fluid (CSF) drainage, and pharmacologic agents [1–4]. In addition, intraoperative monitoring of spinal cord potentials, such as motor-evoked potentials (MEPs), evoked spinal cord potentials (ESCPs), and somatosensory evoked potentials (SSEPs) have been used to assess spinal cord function during surgery [5–10]. However, when spinal cord potentials are monitored, the incidence of paraplegia following thoracic and thoracoabdominal aortic surgery ranges from 4.2% to 11.3% [5,7,8].
Reconstruction of critical arteries with minimal duration of spinal cord ischemia is considered to be advantageous to protect the spinal cord [11]. Preoperative assessment with computed tomography angiography (CTA) [12] or magnetic resonance angiography (MRA) [13] is used for detecting the critical artery that supplies the spinal cord. However, it is difficult to determine which intercostal artery is to be reconstructed when these modalities indicate multiple or no intercostal or lumbar arteries that perfuse the spinal cord. We previously reported prompt changes in transcranial motor-evoked potentials (Tc-MEPs) immediately following cold blood infusion into the clamped segment of aorta [14]. The Tc-MEPs amplitude decreased significantly within 3 min after cold blood infusion when the critical intercostal arteries were present in the thoracoabdominal aorta. In that report, MEPs were elicited by transcranial electrical stimulation and recorded from the spinal epidural space (D-wave).
Based on this result, Tc-MEPs were monitored following cold blood infusion into the clamped segment of aorta as an adjunct ‘on-site assessment’ to determine whether intercostal or lumbar arteries in the aneurysm should be reconstructed. The purpose of this study was to examine the sensitivity and specificity of our Tc-MEP criterion for the presence of a critical artery supplying the spinal cord within the clamped aortic segment.
2 Materials and methods
2.1 Patients
We examined 14 cases that underwent repair of descending thoracic or thoracoabdominal aortic aneurysm, in which intraoperative Tc-MEPs monitoring was feasible throughout the operations and Tc-MEPs changes following cold blood infusion were examined. The profiles of the patients and their surgical procedures are summarized in Table 1 . They included nine men and five women ranging in age from 55 to 86 years old (71.1 ± 9.2 years). The pathology of the aorta included descending thoracic aortic aneurysm in six patients and thoracoabdominal aortic aneurysm classified as Crawford type I, II, III, and IV in 4, 1, 3, and 0, respectively.
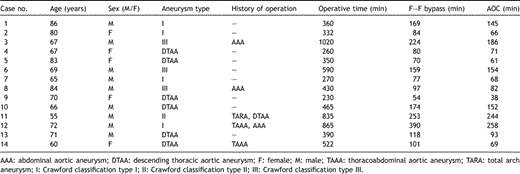
2.2 Measurements of evoked potentials
An epidural catheter with five unipolar electrodes (UKG-100-5PM, Unique Medical, Tokyo, Japan) was placed at the level of the lumbar enlargement (T12-L1) through a 17-gauge Tuohy needle for recording Tc-MEPs and descending ESCPs. Another epidural catheter was introduced into the dorsal epidural space of the cervical spinal cord (C5–C6) for electrical stimulation to record the descending ESCPs. A CSF drainage catheter was inserted at a low lumbar location (L3–L4). Every catheter was inserted on the day before surgery.
After induction of anesthesia, two spiral needle electrodes (001-220, CS-F Electrodes, Agram Co., USA) were placed on the scalp above the bilateral cerebral motor cortices. Direct single-pulse transcranial electrical stimulation (duration, 0.5–1 ms; intensity, 400 V; rate, 7.3 Hz) of the cerebral motor cortices was applied, and Tc-MEPs were recorded at the lumbar enlargement (D-wave) (Fig. 1 ). The descending ESCPs were also recorded at the lumbar enlargement after single-pulse stimulation (duration, 0.2 ms; intensity, 10 mA; rate, 7.3 Hz) of the cervical spinal cord in parallel (Fig. 1). Each spinal cord potential recording was obtained by averaging a total of 20–50 responses.
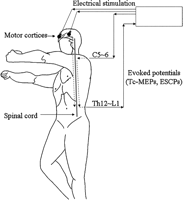
Monitoring of spinal cord evoked potentials. Direct transcranial electrical stimulation of the cerebral motor cortices was applied, and the transcranial motor-evoked potentials (Tc-MEPs) were recorded at the lumbar enlargement. The descending evoked spinal cord potentials (ESCPs) were also recorded at the lumbar enlargement after stimulation of the cervical spinal cord (C = cervical; Th = thoracic; L = lumbar).
Stimulation and recording were performed using a Nicolet Viking select (Nicolet Biomedical, Inc., USA). All action potentials were recorded by one of five electrodes at the lumbar enlargement, which recorded the largest amplitude along the trunk. The amplitude of each spinal cord potential was expressed as a percent change from the corresponding control value recorded prior to the injection of cold blood into the clamped aortic segment.
2.3 Anesthesia
Anesthesia was induced with 10 μg/kg fentanyl and 5 mg/kg propofol and was maintained with 2–4 μg/kg fentanyl per h and 2 mg/kg propofol per h. Muscle relaxation was induced with pancuronium at 0.1 mg/kg and maintained with 0.02–0.04 mg/kg h. Intubation was performed with a double-lumen endotracheal tube under fiberoptic bronchoscopy. Controlled ventilation and isolated lung ventilation were adequately performed.
2.4 Surgical technique
On the right lateral decubitus position, left thoracotomy or thoracoabdominal incisions were adequately performed. A femorofemoral partial cardiopulmonary bypass was established, and the rectal temperature was maintained at 34 °C. While the aorta was cross-clamped, systemic blood pressure was mainly controlled by adjusting the bypass flow. Mean radial arterial pressure was maintained at 60–100 mmHg, while distal aortic pressure was >60 mmHg.
The aorta was clamped at sites proximal and distal to the aneurysm. If the aneurysm extended longer than several vertebrae, the aneurysm was segmentally clamped [15]. Cold blood (4 °C) was infused into the clamped segment of aorta through a 14-gauge needle at a rate of 100–200 ml/min, to a total amount of 200–450 ml. The amount of infused cold blood was determined according to the size of the clamped aortic segment: 300–450 ml for a large segment and 200–300 ml for a small segment. We then observed changes of the Tc-MEPs and ESCPs amplitudes during and after cold blood infusion, and after aneurysmectomy.
2.5 Tc-MEPs criterion following cold blood infusion
When the Tc-MEPs amplitude did not decrease to a level below 50% of baseline during and after cold blood infusion, we concluded that no critical supplying artery was present in the aneurysm, and ligated all intercostal or lumbar arteries in the aneurysm. Then, the proximal and distal parts of the aorta were anastomosed using a 20–30 mm woven double velour vascular graft, and the visceral arteries were reconstructed with the branched graft under visceral arterial perfusion (150 ml/min for each visceral artery) if necessary.
When the Tc-MEPs amplitude decreased to below 50% of baseline within 3 min after cold blood infusion, we determined that the critical supplying artery was present in the clamped segment of aorta, and then the intercostal or lumbar arteries with significant back bleeding were reconstructed. Other intercostal or lumbar arteries were ligated immediately to minimize the steal of collateral flow away from the anterior spinal artery during anastomosis. The intercostal or lumbar arteries were reconstructed using 8 or 10 mm woven double velour vascular grafts. The intercostal artery was reperfused immediately through the attached vascular graft with warm blood at a flow rate of 40–50 ml/min and a perfusion pressure of 100 mmHg for each intercostal artery pair [16]. If the recovery of the Tc-MEPs amplitude was not satisfactory, other intercostal or lumbar arteries were additionally reconstructed. After completing the prosthetic replacement of the aneurysm, each attached vascular graft of the intercostal arteries was anastomosed to the main tube graft and perfused segmentally.
2.6 Statistical analysis
Data were processed using StatView J-5.0 (SAS Institute, Cary, NC) software. All values are expressed as the mean ± standard deviation. Statistical analysis was performed with the Mann–Whitney U test to compare the incidence of spinal cord injury between patients with and without a decrease in Tc-MEPs amplitude to below 50% of baseline, as well as between patients with and without reimplantation of visceral arteries. Differences were considered statistically significant when the p value was <0.05. Confidence intervals for sensitivity and specificity were defined as 95%.
3 Results
3.1 Changes in evoked potentials after cold blood infusion
Changes in spinal cord potentials (Tc-MEPs and ESCPs) and neurologic outcomes are summarized in Table 2 . The changes of Tc-MEPs amplitude after cold blood infusion were examined in all 14 patients during the operation.
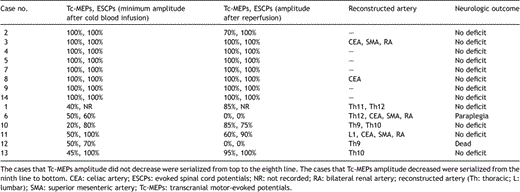
The Tc-MEPs amplitude did not decrease to below 50% of baseline in eight patients (57%) during and after cold blood infusion into the clamped segment of aorta (Fig. 2 ). There was no change in Tc-MEPs amplitude in these eight patients and the Tc-MEPs amplitude within 3 min after cold blood infusion was 100% ± 0% of the baseline value. The ESCPs amplitude also did not change during and after cold blood infusion in these eight cases. Although every intercostal or lumbar artery was ligated, none of these patients presented with paraplegia after surgery.
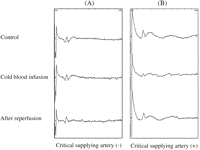
Changes in transcranial motor-evoked potentials (Tc-MEPs) following cold blood infusion. (A) Tc-MEPs without decrease to <50% of baseline during and after cold blood infusion. (B) Tc-MEPs with a decrease to <50% of baseline within 3 min after cold blood infusion.
The Tc-MEPs amplitude decreased to below 50% of baseline in six patients (43%) within 3 min after cold blood infusion (Fig. 2). The minimal Tc-MEPs amplitude within 3 min after cold blood infusion was 43% ± 12%. However, in these six patients, the amplitude of the ESCPs did not change in two, decreased in three, and was not recorded in one. We determined that the critical supplying artery was present in these cases, and therefore, the intercostal or lumbar arteries with significant back bleeding were reconstructed. The number of intercostal arteries reconstructed was one or two pairs in each case. The amplitude of the Tc-MEPs significantly recovered to >50% of baseline after reconstruction in four patients, and these patients had no postoperative paraplegia. In the remaining two cases, however, the amplitude of the Tc-MEPs did not recover, even though we reconstructed as many intercostal and lumbar arteries as possible, and they were perfused immediately with warm blood through the attached vascular graft. One patient died of multiple organ failure and did not have any postoperative assessment. The other patient developed paraplegia following surgery. In the latter case, the amplitude of the Tc-MEPs decreased within 3 min after cold blood infusion and further dropped following aortotomy. The twelfth intercostal artery with significant back bleeding was reconstructed. The amplitude of Tc-MEPs did not recover despite selective perfusion of this artery. The first and second lumbar arteries were not reconstructed because they were already occluded. There was no significant difference in the incidence of spinal cord injury between six patients with a decrease in Tc-MEPs amplitude and eight patients without a decrease in Tc-MEPs amplitude (p = 0.21). In 13 cases in which postoperative neurological assessment was feasible, a critical artery supplying the spinal cord was detected in 5 patients, and was not detected in 8 patients. Both the sensitivity and specificity of our criterion for detection of a critical artery was 100%.
3.2 Classification by the extent of aortic replacement
The 13 patients except for 1 patient with operative death were divided into two groups: a thoracic group (descending thoracic aortic aneurysm and Crawford type I aneurysm) and a thoracoabdominal group (Crawford type II and III aneurysm). The incidence of spinal cord injury was examined in each group. In the thoracic group, three of nine patients (33%) presented with a Tc-MEPs decrease (below 50% of baseline) but none had spinal cord dysfunction (0%). In the thoracoabdominal group, two of four patients (50%) had a decrease of Tc-MEPs and one had postoperative paraplegia (25%). There was no significant difference in the incidence of spinal cord injury between the two groups (p = 0.13).
4 Discussion
This study showed that changes in Tc-MEPs amplitude within 3 min after cold blood infusion indicate the presence or absence of a critical artery that supplies the spinal cord. The Tc-MEPs monitoring with cold blood infusion may potentially serve as an adjunct on-site method in thoracoabdominal aortic surgery.
Although Tc-MEPs monitoring is widely used for assessment of spinal cord function during surgery [5,8–10], it often takes longer than 10 min to detect amplitude changes and the diagnostic process is associated with ischemic injury of the spinal cord. We previously reported that a rapid decrease in amplitude of Tc-MEPs following cold blood infusion indicated the presence of a critical spinal cord artery in the aneurysm [14] and have used Tc-MEP monitoring with cold blood infusion. The mechanisms of amplitude decrease may be related to local hypothermia by cold blood (4 °C). In an experimental study, intra-aortic infusion of cold lactated Ringer’s solution (3 °C) cooled the spinal cord by 6–7 °C [17]. Hypothermia induces a depression of the electrophysiologic response of both cerebral and peripheral neurologic activity [18,19]. This topical cooling of spinal cord might induce the rapid decrease of Tc-MEP amplitude [17–19].
Van Dongen and colleagues reported that when the amplitude of Tc-MEPs was ≤50% of baseline during cross-clamping and closure of the skin, the relative risks of paraplegia after thoracic and thoracoabdominal aortic aneurysm repair were respectively 3.4-fold and 31-fold compared with Tc-MEPs >50% of baseline [8]. Based on this data, we assigned the cut-off point of the Tc-MEP amplitude as 50% of baseline. A duration of 3 min was used for detecting Tc-MEP changes, because the Tc-MEP amplitude decreased within 3 min in our previous experience [14].
Paraplegia did not occur in cases without a significant decrease in Tc-MEP amplitude, although the intercostal or lumbar arteries were ligated. This on-site assessment may reduce the operative time by shorting the assessment time (3 min) and reducing the time for unnecessary reconstruction.
In a case of postoperative paraplegia, the spinal cord might have been injured, or another intercostal artery was the critical artery. Regretfully it is not feasible to specifically identify one intercostal artery. Nevertheless, the presence/absence of a critical supplying artery can be determined within 3 min with a sensitivity and specificity of 100%. Accelerated Tc-MEP changes with topical cooling can possibly reduce the ischemic insult of the spinal cord during the diagnostic process.
The extent of aortic replacement can affect outcomes as well. In this series, the incidence of paraplegia was 0% in the thoracic group and 25% in the thoracoabdominal group. There was no statistical difference between the two groups because of the small number of cases. We admit that the incidence of postoperative paraplegia (7.7%) is not lower than that in other excellent centers, because of the limited number of cases in this series. Further study with a larger number of cases is needed to evaluate our criterion.
Changes in Tc-MEP amplitude were more rapid than in ESCP amplitude. This difference may be due to temperature sensitivity of each evoked potential or due to ischemia [18,19]. The Tc-MEPs appeared to be more accurate than ESCPs in the assessment of spinal cord ischemia during aortic surgery.
In this series, changes in Tc-MEPs were discrete; either there was no change or a decrease to below 50%. Although it is uncertain as to whether a 50% decrease is an appropriate cut-off value, this criterion was adequate in this series. A 100% sensitivity and specificity can be obtained with a cut-off value between 50% and 90% of baseline as well. If the cut-off value is increased to limit false-negative results (for example, ligation of a critical supplying artery), then the number of arteries that need reconstruction will increase.
A limitation of cold blood infusion should be noted. It is hard to carry out in cases of dissecting aneurysm or highly atheromatous aneurysm, because of possible inappropriate infusion into the false lumen in the former and because of embolism in the latter.
In conclusion, Tc-MEP monitoring following cold blood infusion into the clamped segment of aorta is a rapid and accurate method for detecting the presence of critical supplying arteries in the clamped segment of aorta. This method may be helpful for minimizing the duration of spinal cord ischemia and unnecessary reconstruction of intercostal arteries. It appears to be a promising adjunct for on-site assessment during descending thoracic or thoracoabdominal aortic surgery.
References
Author notes
Presented at the 21st Annual Meeting of the European Association for Cardio-thoracic Surgery, Geneva, Switzerland, September 16–19, 2007.