-
PDF
- Split View
-
Views
-
Cite
Cite
Zachary N. Kon, Emile N. Brown, Michael C. Grant, Toshinaga Ozeki, Nicholas S. Burris, Michael J. Collins, Michael H. Kwon, Robert S. Poston, Warm ischemia provokes inflammation and regional hypercoagulability within the heart during off-pump coronary artery bypass: a possible target for serine protease inhibition, European Journal of Cardio-Thoracic Surgery, Volume 33, Issue 2, February 2008, Pages 215–221, https://doi.org/10.1016/j.ejcts.2007.11.008
- Share Icon Share
Abstract
Objective: Accumulating evidence suggests that a hypercoagulable state influences early graft failure after off-pump coronary artery bypass (OPCAB). We hypothesized that regional myocardial ischemia caused by obligatory periods of coronary occlusion during OPCAB is an important trigger for this prothrombotic state. Methods: Using a series of biomarkers, 60 consecutive patients undergoing OPCAB were monitored for myocardial injury (myoglobin), inflammation (TNF-α, IL-8) and thrombosis (thrombin generation-F1.2, contact activation pathway-FXII-a, platelet derived microparticles-via flow cytometry). The transcardiac gradients of these markers were determined by assaying both arterial and coronary sinus blood just after protamine administration. Intramyocardial pH was monitored continuously during coronary occlusion in a subset (N = 30 grafts, 11 patients). The influence of management strategies affecting hemostasis (e.g. antiplatelet therapy, anti-fibrinolytics, peak activated clotting time (ACT) during heparinization) was analyzed. Results: Ischemic injury, depicted by the transcardiac myoglobin gradient, significantly correlated with intramyocardial acidosis during coronary occlusion (R = 0.96, p < 0.0001) and predicted the transcardiac gradients of TNF-α (R = 0.83, p < 0.001) and F1.2 (R = 0.72, p < 0.0001). Transcardiac F1.2 strongly correlated with TNF-α (R = 0.73, p = 0.01) and IL-8 (R = 0.51, p = 0.02). Patients receiving aprotinin (N = 20) showed significantly lower transcardiac gradients for myoglobin (4.1 ± 7.5% vs 72.9 ± 108.8% change, p = 0.002), F1.2 (31 ± 37% vs 89 ± 149%, p = 0.03), FXII-a (2.6 ± 4.1% vs 19.2 ± 34%, p = 0.04) and microparticles (7 ± 3.9% vs 12.9 ± 8%, p = 0.01). Conclusions: Strong correlations between myocardial ischemia and the transcardiac gradients of markers for inflammation and thrombosis suggest that even brief episodes of coronary occlusion in the beating heart may have pathophysiologic consequences. Aprotinin, but not other factors that influence the coagulation system, appears to mitigate this process during OPCAB.
1 Introduction
Off-pump coronary artery bypass graft surgery (OPCAB) circumvents the need for global myocardial ischemia inherent with CABG performed on the arrested heart and requires only brief periods of regional ischemia during the coronary anastomoses. On occasion, this regional ischemia is tolerated poorly and results in arrhythmias and myocardial stunning, but this risk is minimal when coronary occlusion is kept to <10–15 min per anastomosis [1]. As a result, randomized studies comparing off- vs on-pump CABG have documented improvements in myocardial injury and early hemodynamics after OPCAB [2–4]. However, the impact of regional warm ischemia on inflammation and coagulation after OPCAB has not been studied.
Thrombin mediates a variety of inflammatory and thrombotic processes associated with ischemia-reperfusion injury. In prior OPCAB studies, we noted a burst of thrombin production from the heart minutes after protamine administration [5], reminiscent of the well-described ‘rebound hypercoagulable state’ that occurs in unstable angina patients after heparin withdrawal [6]. The prothrombin cleavage product, F1.2, has been established as a surrogate marker of a prothrombotic state through significant associations with the risk of acute vein graft failure [7], myocardial injury [8] after CABG, and major adverse cardiovascular events [9]. Thrombin production can be mitigated by enhanced antiplatelet therapy [10] as well as the use of higher heparin doses during cardiopulmonary bypass [11]. Aprotinin, used primarily for its hemostatic properties, has been suggested to have anti-thrombotic effects via inhibition of the thrombin receptor, protease activated receptor-1 (PAR-1) [12–15]. Although other PAR-1 antagonists have been documented to reduce major adverse cardiovascular events [16], there are few clinical reports to suggest an anti-thrombotic effect from aprotinin despite administration to thousands of on-pump CABG patients. In contrast to conventional CABG, we hypothesized that OPCAB creates a series of pathologic findings in response to regional warm ischemia (e.g. local activation of thrombin, platelets and the contact activation pathway) that provide a more favorable model for demonstrating the clinical effects of PAR-1 inhibition by aprotinin.
2 Methods
2.1 Patient selection and enrollment
Following IRB approval (UMB protocol #25350), 60 consecutive patients underwent off-pump CABG at our institution. All subjects provided informed consent before enrollment into this prospective, observational study of patients undergoing OPCAB with no aprotinin (n = 40) or off-pump CABG with a modified full-dose regimen of aprotinin (n = 20), as previously described [15]. Assignment to the aprotinin group was nonrandomized. Hemodynamic instability, acute coronary syndromes or situations in which complete revascularization was not possible served as exclusion criteria. Patients with chronic renal insufficiency (creatinine >2.0 mg/dl), and allergy to radiographic contrast were also excluded from enrollment. Demographics, preoperative risk factors and medications, intra- and postoperative data were prospectively recorded into a relational database.
2.2 Treatments
A modified full-dose regimen was used as previously described. Heparin dose was calculated by protamine titration using a HMS heparin assay (0.0–2.5 mg/kg cartridge, Medtronic, Minneapolis, Minnesota) to maintain levels greater than 2 μg/ml and a kaolin-based ACT greater than 300 s. The heparin effect was partially reversed by administering half the dose of protamine calculated by the HMS device. The algorithm for intraoperative and postoperative blood product transfusions was based on thrombelastography analysis, as described [17]. All patients received aspirin (325 mg orally each morning and within 6 h after intensive care unit arrival) as the sole postoperative platelet inhibitor. Glucose levels were maintained at less than 150 mg/dl using routine insulin infusions.
2.3 Surgery and perioperative management
After median sternotomy, the left internal thoracic artery was used in all patients; the saphenous vein was harvested using an endoscopic (VasoView6®, Guidant Systems, Inc., Minneapolis, MN) or open approach, based on anatomical considerations. Conduits were stored in heparinized saline after harvest. Proximal anastomoses were performed using a partial occluding aortic clamp. All distal anastomoses were facilitated by suction-based exposure and stabilizing devices (Octopus 4.3®, Medtronic, Inc., Minneapolis, MN) without intracoronary shunts. Shed mediastinal blood was collected intraoperatively using a cellsaving device (Cobe BRAT 2; Arvada, CO), processed, and retransfused.
2.4 Intraoperative blood flow analysis
Blood flow and flow waveform were measured in each graft using transit time ultrasound (Transonic, Inc., Ithica, NY). Waveforms were analyzed for pulsatility index and percentage diastolic flow using digital data acquisition software (WinDaq™, DATAQ Instruments, Inc., Dayton, OH).
2.5 Blood sample collection
Blood samples, collected in tubes containing 3.2% citrate, were obtained preoperatively from the arterial line. Postoperatively, 30 min after heparin reversal with half dose protamine, blood was obtained from the systemic arterial line and coronary sinus (CS). Additional systemic blood was collected on postoperative days 1 and 3. Platelet-poor plasma was obtained by rapid centrifugation (2000 × g) and stored at −80 °C.
2.6 Myocardial injury and acidosis
Cardiac troponin I (Tn-I) and myoglobin were both assessed using ELISA kits (Life Diagnostics, Inc., West Chester, PA). Regional (i.e. anterior and posterior ventricular wall) changes in pH were monitored in real time using the Khuri™ pH monitoring system (Terumo Corp, Tokyo Japan).
2.7 Assays for coagulation and inflammation
Thrombin formation was evaluated by assaying postoperative systemic and CS samples for the presence of prothrombin fragment 1.2 (F1.2) (Dade Behring, Marburg, Germany). The contact activation pathway was evaluated by assaying FXII-a (American Diagnostica, Inc., Stamford, CT). Inflammation was assessed via interleukin-8 (IL-8) and tumor necrosis factor-α (TNF-α) using ELISA (Bender MedSystems, Vienna, Austria). Comparison of the concentration of these markers in the CS to a simultaneously obtained aortic (Ao) sample allowed for calculation of %transcardiac change as follows: (CS − Ao)/Ao × 100.
2.8 Assays for platelet function
2.8.1 Thrombelastography (TEG™, Haemoscope, Niles, IL)
Maximum amplitude (MA) of the TEG trace was measured after 30 s of activation by kaolin or tissue factor (0.0001% Innovin®, Dade-Behring).
2.8.2 Whole blood aggregometry (Chronolog, Hawerton, PA)
Impedance changes (Ω) were assessed at 6 min following the addition of 1 μg/ml and 5 μg/ml collagen as described [7].
2.8.3 Whole blood flow cytometry (Becton-Dickinson FACScan, Franklin Lakes, NJ)
Citrated blood samples were diluted with modified Tyrode Buffer containing 5 mM GPRP (Centerchem Inc., Norwalk, CT) and then incubated with antibodies against CD41-FITC (Serotec, Raleigh, NC) and AnnexinV-APC (BD Biosciences, San Jose, CA). After 20 min incubation, the samples were fixed with 1% paraformaldehyde and stored at 4 °C until analysis within 72 h by FACS. Both, forward scatter and sideward light scatter were set at logarithmic gain, and platelet derived microparticles were identified. The %annexin-positive microparticle events compared to total CD41 positive events was calculated as described [18].
2.9 Endothelial integrity
Surplus segments from each bypass conduit were stored in balanced salt solution at room temperature for 30–60 min, embedded in cutting compound (Tissue-Tek® O.C.T., Redding, CA) and then frozen in liquid nitrogen. Four separate 5 μm thick sections were assessed for the expression of an EC marker, CD31 (R&D System, Inc., Minneapolis, MN) using immunohistochemistry. The %vessel circumference positive for CD31 was calculated in each section using image analysis software (Bioquant Nova Prime, Nashville, TN) and EC integrity defined by the average %CD31 staining for all sections analyzed [7].
2.10 Graft patency
Bypass graft patency was determined by blinded review of a 64 detector row, CT angiography (CTA) scan (420 ms rotation, 100–150 ml contrast agent IV at 5 cc/s) using retrospective ECG gating. Patency was defined as any flow through the entire graft regardless of the presence of stenosis. The graft was classified as nonpatent if a stump was seen or if there was no contrast in an area known by operative report to contain a graft.
2.11 Statistics
The primary endpoint of this trial was a comparison of markers of regional hypercoagulability and inflammation after OPCAB with and without the use of aprotinin. A previous investigation of aprotinin by our group [15] showed a three-fold decrease in transcardiac F1.2 compared to control. Therefore, 20 patients per group would provide a >80% power to detect a similar difference in F1.2 and other markers in this cohort at p = 0.05, assuming a standard deviation of 25%1. Groups were compared by means of the unpaired Student’s t-test for continuous variables and the Fisher’s exact test for categorical variables. The area under the concentration–time curves was compared for markers that were measured serially (e.g. cTn-I). Correlations were examined using linear regression for parametric data. Statistical analysis was performed using the InStat™ statistical package with the assistance of a biostatistician.
3 Results
3.1 Patient population
OPCAB patients that received aprotinin were similar to the control group in all analyzed clinical variables (Table 1 ) and received equal number of grafts per group (3.1 ± 0.4 vs 3.2 ± 0.5). None of the patients in this cohort required conversion to on-pump techniques and 100% underwent follow-up of graft patency prior to hospital discharge. There were no deaths and no evidence of hypersensitivity reaction to aprotinin in any of the patients during the study.
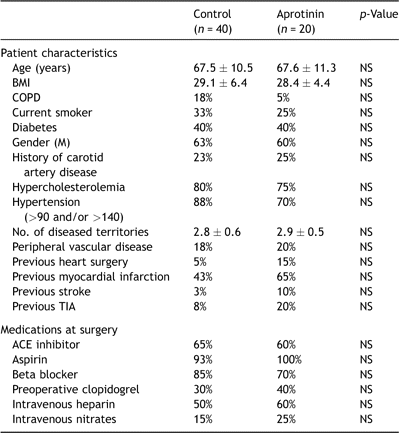
3.2 Myocardial acidosis/ischemia and regional hypercoagulability
Despite equivalent periods in which the coronary arteries were occluded during OPCAB, the subset of patients that underwent tissue pH monitoring showed a wide variation in the degree of acidosis that developed in the regional myocardium (Fig. 1 ). The mean pH drop during native vessel occlusion showed a significant correlation with the transcardiac gradient of myoglobin assessed 30 min after the completion of grafting (R = 0.96, p < 0.001). Myocardial injury, as defined by the transcardiac myoglobin release, showed a strong correlation with the transcardiac gradient of F1.2 (R = 0.72, p < 0.0001). This F1.2 gradient, in turn, showed significant correlations with the transcardiac gradients of TNF-α (R = 0.73, p = 0.01), IL-8 (R = 0.51, p = 0.02) and FXIIa (R = 0.29, p = 0.03) but not with the release of platelet derived microparticles.
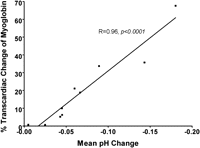
Correlation between regional acidosis and myoglobin release. Tissue pH was monitored during the distal anastomoses performed during OPCAB. We noted varying degrees of intramyocardial acidosis despite coronary occlusion times that were nearly identical between anastomoses and always <15 min. Myoglobin release was measured at the completion of OPCAB, 30 min after protamine administration. A transcardiac gradient was calculated by analyzing myoglobin levels from blood samples obtained simultaneously from the aorta and coronary sinus. A strong linear correlation between the mean regional pH change intraoperatively and transcardiac myoglobin release postoperatively.
3.3 Effects of aprotinin
When comparing perioperative myocardial injury for patients in the aprotinin vs control groups, we noted a significant reduction in transcardiac myoglobin gradient measured postoperatively in those treated with aprotinin. However, Tn-I release was not significantly different between groups (Fig. 2 ). Compared to control, the aprotinin group showed statistically significant decreases in the transcardiac gradients of F1.2, FXII-a, and platelet derived microparticles released into the CS (Fig. 3 ). We found no statistically significant difference in systemic platelet function between the groups at any measured time point (Table 2 ).
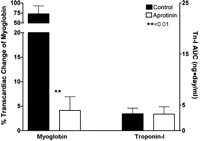
Myocardial injury after OPCAB in the aprotinin vs control groups. Myocardial injury was measured by analyzing the transcardiac gradient of myoglobin at 30 min following the completion of OPCAB and by comparing the area under the concentration–-time curve of cardiac troponin I (cTn-I) levels measured at 6, 24 and 72 h. While transcardiac myoglobin release was significantly reduced in the aprotinin vs control group, cTn-I was not significantly different.
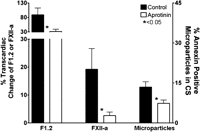
Differences in thrombosis markers in the aprotinin and control groups. Thrombin production and the contact activation pathway were monitored after OPCAB by measuring the transcardiac gradients of F1.2 and FXII-a. Platelet activation within the coronary circulation was assessed by determining the proportion of CD41+, Annexin+ microparticles released from the heart, normalized against the number of platelets in the sample. The aprotinin group showed significantly reduced thrombin formation (F1.2), inhibition of contact activation (FXII-a) and reduced microparticle formation within the heart after OPCAB.
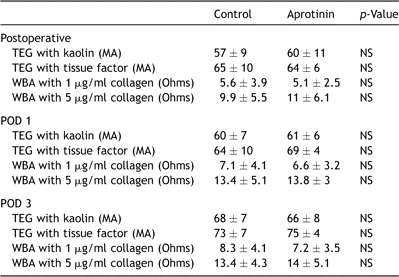
Other management strategies affecting hemostasis such as receiving clopidogrel until surgery or developing a peak ACT of >400 s, did not appear to significantly decrease any of the transcardiac coagulation markers (Table 3 ).

3.4 Graft quality and patency
The quality of the conduits and their anastomoses appeared to be similar between the control and aprotinin groups as defined by similar endothelial integrity (38 ± 37% vs 38 ± 39% luminal surface positive for CD31, p = NS); and measurements of blood flow (37 ± 17 ml/min vs 42 ± 21 ml/min, p = NS), %diastolic flow (51 ± 19% vs 60 ± 4%, p = NS), and pulsatility index (2.1 ± 0.9 vs 3 ± 2, p = NS). Flow in the venous grafts in both groups showed inverse correlations with the transcardiac gradient of myoglobin (R = −0.65, p < 0.05) and the risk of developing the thrombin ‘burst’ after protamine (i.e. >two-fold transcardiac gradient in F1.2, Fig. 4 ). Although we noted an interesting trend in the incidence of vein graft failure defined by postoperative CT angiography (5% vs 0% failure for control vs aprotinin), the results did not reach statistical significance.
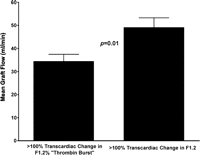
Increased risk of the thrombin burst in patients with low flow bypass grafts. Most patients showed at least some increase in F1.2 in the coronary sinus vs the aortic blood after OPCAB. However, the ‘thrombin burst’, defined as a two-fold or greater transcardiac increase in F1.2, was noted in only 18 (30%) patients. Mean blood flow within the venous grafts was significant reduced in those patients who developed the thrombin burst at the end of the case compared to those with F1.2 levels less than this threshold.
4 Discussion
Perhaps due to differences in collateral formation or ischemic preconditioning [1], we noted a wide variability in the cardiac release of myoglobin during this study despite the requirement of similar periods of coronary occlusion between OPCAB patients in this cohort. As a low-molecular-weight protein, myoglobin leaks from injured myocytes earlier than traditional markers such as cTn-I [19], thus providing a more sensitive measure of injury. Patients with more severe myocardial injury, in turn, developed a local inflammatory and hypercoagulable state as depicted by heightened arterial-coronary sinus (i.e. transcardiac) gradients for TNF, IL-8, F1.2, FXII-a, and platelet derived microparticles. We previously established that regional thrombin production defined by these assays is exacerbated when CABG is performed off- compared to on-pump [5]. The main finding of the current study was that myocardial injury induced by warm ischemia, a feature unique to OPCAB, appears to be an important trigger to explain this difference. In addition, the strong correlation noted between the gradient of F1.2 with most of the other markers that were analyzed supports a role for thrombin as critical link between coagulation and inflammation occurring within the heart during OPCAB.
We also analyzed the impact of different therapeutic approaches employed in our cohort that might have an influence on hemostasis and thrombotic risk. A portion of our cohort was receiving clopidogrel until surgery and/or developed a higher intraoperative ACT level (e.g. >400 s). In these patients, we found no difference in the regional production of thrombin or any other marker compared to those treated with aspirin monotherapy and intraoperative ACT levels <400 s. These findings contradict other reports suggesting that these strategies are effective ways to reduce thrombin production [10,11]. However, these investigations analyzed thrombin production in the systemic circulation. Analysis of CS blood provides a more sensitive and specific assay of events within the upstream coronary circulation [20,21]. We speculate that the application of this method in our study improved our pathophysiological insight into conditions that influence the risk of graft thrombosis after OPCAB.
Aprotinin administered prior to skin incision at full dose was associated with a significant reduction in the transcardiac gradient of the coagulation markers that were analyzed when compared to the control group. Activation of PAR-1 is likely an important avenue through which the platelet is able to respond to thrombin and participate in the feedback thrombin burst necessary for graft thrombosis. Aprotinin has been shown to block PAR-1 in vitro[12] and in vivo[14]. Nevertheless, conventional wisdom suggests that aprotinin is a prothromobic agent because of its potent hemostatic effects. Establishing a clear definition of the hypercoagulable state is a vital step towards better understanding the relationship between the use of aprotinin and risk of thrombosis.
On the other hand, aprotinin also inhibits fibrinolysis, an important mechanism through which the coronary circulation keeps thrombus formation in check. This has raised considerable concern that aprotinin might increase the risk of thrombosis [22]. The safety of aprotinin remains an important and controversial issue in cardiothoracic surgery, with conflicting evidence arising from randomized clinical trials [23] and observational studies [22] during on-pump CABG regarding issues such as renal dysfunction and even death. However, these nonrandomized observational trials have recently been reviewed by an FDA advisory panel and deemed statistically flawed in their analysis. As a result of the reanalysis of the raw data from these studies, the panel reached significantly more favorable conclusions regarding the safety of aprotinin2. There has also been a more recent meta analysis by the Cochrane group [24] looking at over 200 randomized controlled trials and over 20,000 patients that found no increased risk of myocardial infarction, stroke, graft thrombosis or death with aprotinin use. On the other hand, renal dysfunction does appear to be a consistent risk that emerges in studies analyzing the safety of aprotinin use. The continued marketing of aprotinin for cardiac surgery is likely going to require further more in depth study of this important issue.
Another plausible approach to investigate would have been to prevent warm ischemic injury to the myocardium by the routine use of intracoronary shunts during the distal anastomoses. Shunts were not used in this cohort out of concern for injury to the coronary endothelium during placement [25]. Therefore, we are not able to address the merits of this idea.
In previous studies, our group has demonstrated that endothelial disruption in the bypass conduit directly relates to regional thrombin production after OPCAB [7]. In this study, we add lower intraluminal blood flow within the grafts as an additional risk factor (Fig. 4). Platelets and coagulation factors are known to react to abnormalities in flow and endothelium by participating in thrombin generation. There is not likely to be a systematic difference in the endothelial quality or blood flow within the coronary circulation during on- and off-pump CABG. Therefore, technical differences between these procedures may help explain the discrepancy in thrombin production. Warm myocardial ischemia is an issue that is unique to OPCAB and has been shown to be a more potent stimulant of thrombin production than cold ischemia in a variety of experimental and clinical studies [26]. We feel that our data point to this issue is a primary stimulus of the hypercoagulable state after OPCAB.
4.1 Limitations
The main limitations of this study were the small number of subjects that were analyzed and nonrandomized study design. Given insufficient numbers, we were unable to perform a multivariate analysis to confirm that aprotinin was an independent predictor of regional hypercoagulability and were underpowered to establish a link to hard endpoints such as postoperative saphenous vein graft (SVG) failure and myocardial infarction (MI). Our study screened for factors that are most likely to influence regional hypercoagulability such as anastomotic and endothelial quality of each SVG and platelet function using three separate assays. However, the nonrandomized use of aprotinin is likely to have introduced confounding factors that remain unaccounted for in our analysis. In addition, the clinical intrusiveness of the protocol limited our coronary sinus measurements to a single 30 min time point after protamine administration, which may have underestimated the incidence of the thrombin burst reaction after OPCAB. Finally, because this study was performed only at a single center, generalizability to other centers may be limited by institution specific practices that effect thrombin production such as the aggressive use of the cell saver during OPCAB and SVG harvesting and preparation techniques. For these reasons, our findings are best interpreted as ‘hypothesis generating’ pending the completion of an ongoing prospective clinical trial to investigate the relationship between regional hypercoagulability markers and early graft failure.
4.2 Conclusion
In the present study, we demonstrated that intramyocardial acidosis following obligatory periods of warm ischemia during OPCAB was associated with the activation of a regional hypercoagulable and inflammatory state. Intraoperative aprotinin use during OPCAB was found to attenuate these findings, perhaps via inhibitory effects on PAR-1 and/or other serine proteases. These data support further investigation of a novel role for aprotinin (or other PAR-1 antagonists) during surgical revascularization: as an adjunct to help abrogate the postoperative hypercoagulable state after OPCAB, and potentially improve early graft patency.
References
http://www.fda.gov/OHRMS/DOCKETS/AC/07/slides/2007-4316s1-12-FDA-Levenson.ppt, accessed on 10/15/07.
Author notes
Presented at the 21st Annual Meeting of the European Association for Cardio-thoracic Surgery, Geneva, Switzerland, September 16–19, 2007.
R.P. is funded by grants from the NIH (R01HL084080), American Heart Association (Scientist Development Grant, 043518N), University of Maryland (intramural grant), Tobacco Restitution Fund at the University of Maryland and Bayer Pharmaceutical Corp (phase IV grant).
- aprotinin
- ischemia
- myocardial ischemia
- tumor necrosis factors
- thrombosis
- acidosis
- coronary occlusion
- myoglobin
- hemostatic function
- blood platelets
- inflammation
- thrombophilia
- flow cytometry
- blood coagulation
- interleukin-8
- protamines
- serine endopeptidases
- tissue transplants
- heart
- hemostasis procedures
- thrombin
- myocardial injury
- coagulation process
- off-pump coronary artery bypass
- coronary sinus
- cell-derived microparticles