-
PDF
- Split View
-
Views
-
Cite
Cite
Abdulrazzaq Sulaiman, Loîc Boussel, Frédéric Taconnet, Jean Michel Serfaty, Hasan Alsaid, Cherif Attia, Laurent Huet, Philippe Douek, In vitro non-rigid life-size model of aortic arch aneurysm for endovascular prosthesis assessment, European Journal of Cardio-Thoracic Surgery, Volume 33, Issue 1, January 2008, Pages 53–57, https://doi.org/10.1016/j.ejcts.2007.10.016
- Share Icon Share
Abstract
Purpose: It is essential to evaluate new stent designs before in vivo testing. The purpose of this study was to develop and validate a controlled and reproducible patient-derived process to produce a life-size in vitro model of aortic arch aneurysm for endovascular procedure simulation. Methods: A three-dimensional magnetic resonance angiography (3D MRA) image derived from a 60-year-old patient with aortic arch aneurysm was segmented using a home-made software package which allows one-click automatic segmentation of the aorta, meshing, and conversion to standard tessellation language (STL) format. A rapid prototyping technique established a stereolithographic model to produce a replica of the whole aorta, including the arch aneurysm and supra-aortic arteries. Results: The final model was made by pouring silicone rubber to obtain a sturdy, life-size, soft, transparent, plastic cast, accurately reproducing both the internal and external anatomy of the aortic aneurysm. This model was used under perfusion by an extracorporeal circulation pump, to test ex vivo stent deployment. Conclusion: The combination of easy segmentation and conversion to the STL format with industrial stereolithography techniques enabled a realistic silicon vascular phantom to be created for endovascular procedure simulation, image modality calibration, and new stent design.
1 Introduction
Prototyping techniques (stereolithography) originally developed for building components from computer-aided designs (CAD) in the motor industry are now being applied in medicine to build models of human anatomy from high-resolution multiplanar imaging data such a computed tomography (CT) or magnetic resonance imagery (MRI). These techniques have found applications in radiotherapy [1] and for surgical planning, particularly in the craniofacial and maxillofacial fields, and for neurosurgery [2,3]. There has been steady progress in the quality of in vitro models during the past decade and it is now possible to obtain models and actual replicas of many arterial diseases [4–14].
In vivo animal experimentation is an interesting means of validating new endovascular techniques or measurements, as clinical situations can be mimicked. These are, however, expensive procedures that are difficult to control, and measurement accuracy cannot be precisely determined.
To overcome these limitations, many in vitro phantoms have been developed, but almost all of them are simple and not physiologically shaped. There is a real need for realistic in vitro models for evaluating endovascular techniques, testing new endovascular material, and for training purposes ahead of in vivo studies.
Stereolithography, a rapid technique used by industrial engineers to prototype parts, could offer an advantageous alternative to animal models in mimicking clinical situations. It can design complex luminal shapes with accurate geometrical data, using computer-assisted technology to build a digital model from a liquid photopolymer that solidifies under laser. The technique requires conversion of digital imaging and communication in medicine (DICOM) format CT or MR files to the STL format, which is a file format native for stereolithography; these files describe only the surface geometry of a three-dimensional object without any representation of color, texture, or other common CAD model attributes.
The purpose of the present study was to develop and validate a controlled and reproducible patient-derived process to produce a life-size in vitro model of aortic arch aneurysm. This phantom was then used to evaluate an endovascular stenting procedure.
2 Material and methods
2.1 Imaging
The original data set was obtained from 3D MR angiography images of a 60-year-old patient with thoracic aorta aneurysm. The MRI technique consisted of a 3D gradient echo gadolinium-enhanced subtract scan (Philips Intera 1.5T, Best, Nederland) with 1 mm3 voxel size. The resulting 3D data volume includes the entire thoracic aorta as well as large portions of supra-aortic arteries. Image data were stored on a CD-Rom in standard DICOM format for processing by Maracas software [15].
2.2 Image processing
The DICOM MRI images were then exported (Fig. 1 ) using Maracas (magnetic resonance angiography computer-assisted analysis). This PC-based software combines the most commonly used 3D visualization techniques with image processing applied to vascular morphology analysis in MRA images. The process is performed in four steps: vessel centerline extraction, lumen boundary detection in planes locally orthogonal to the centreline, segmentation and conversion to STL format. The geometry of the complete model was then exported in STL format in a form that can be interpreted by a rapid prototyping apparatus where the information can be processed into a physical model.
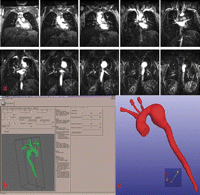
(a) Native coronal MRA images in DICOM format show the aortic arch aneurysm. (b) 3D image of the whole volume after segmentation by Maracas software. (c) Final 3D image after conversion to STL format.
2.3 Manufacture (rapid prototyping)
A life-size model of the aortic arch aneurysm was produced with the main left subclavian, left carotid, and brachiocephalic arteries on a stereolithography apparatus (3D Systems Corp., Valencia, CA). This prototyping device creates 3D replicas in epoxy resin. A low-powered, highly collimated laser beam is focused on the surface of a container filled with liquid resin. The laser traces a cross-section of the model, solidifying a thin layer of the liquid resin. The first cross-section is lowered and recoated with liquid resin and the next slice is placed on top of the previous one. The process continues layer by layer until the part is complete. Two polished, stereolithographic models were produced, one to scale and the other 2 mm larger. The oversized model was used to make a two-piece outer mold with a filling funnel and alignment/support tabs. The two pieces of this mold were assembled over the scale model, so as to obtain a three-piece cast with an annular interval of 2 mm between the external surface of the scale model and the interior surface of the mold (Fig. 2 ).
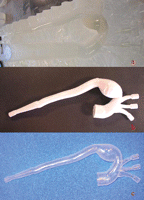
After reconstruction of the aorta by the stereolithography machine, the plaster-like reproduction of the vessel lumen was used to make a master mold (a). In (b) the mold is open, revealing the wax model. (c) Soft, transparent, plastic, life-size replica of the aneurysm made by the lost-wax technique.
Clear silicone rubber was then poured into this cast. After curing, the mold was opened and the inner core broken out so as to obtain a sturdy, soft, transparent, plastic, life-size replica of the aneurysm.
A test bench was then designed in the form of a non-magnetic MR-compatible support with various articulated branches to hold the ascending aorta, the three branches of the arch, and the abdominal aorta. A plastic tubing network allowed each of these branches to be perfused under extracorporeal circulation (Ismatec pump, Fishier Bioblock Scientific, Illkirch, France). The phantom was easily connected to the test bench and articulated branches and to the connecting tubes by non-magnetic clamping rings, without leakage at any of the pump pressures (mean pressure = 60–120 mmHg)
3 Results
3.1 Phantom
Image processing (segmentation and STL file format conversion) lasted 20 min, surface reconstruction 2 days (with a qualified CAD user), stereolithography 48 h (polymerization requiring 4 h), and the total time from MRA to final replica was 1 week.
Considering the MRI images in their STL file format, the internal dimensions of the resulting life-size replica of the aortic arch aneurysm are very close to that of the patient’s STL format images with a variance less than 0.52 at four different levels. While the external diameter of the replica, as measured with electronic calipers, corresponded to that of the internal diameter of the replica plus 2 mm as the wall thickness of the replica is uniform everywhere.
3.2 Experimental procedure
The experiment was performed in the angiography room (Philips V5000, Best Nederland) with both X-ray and video camera recording, under extracorporeal circulation, using normal water warmed to 37 °C as perfusate with 1 ml Azorubine (Gifrer, France) added to get a red-colored, radio-opaque circuit (Fig. 3a ). The delivery system was introduced through a femoral-like port-entry 24F introducer, along a 0.035 guide wire, under fluoroscopic control. The in vitro stent deployment was performed using the valiant thoracic stent graft with Xcelerant (R) Delivery System (Medtronic, Inc.). The system also used a FreeFlo proximal end. The stent dimension was 24 mm by 150 mm.
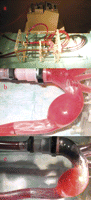
(a) Aortic arch aneurysm under extracorporeal circulation. (b) Stent deployment. (c) Absence of endoleak after methylin blue injection.
Under direct visual and angiographic control, the guide wires, straight catheters, and self-expandable stent were easily deployed with negligible air and water escape during the installation of the introducer and the delivery system. No difficulties were encountered in fitting the stent.
Angiograms of the stented aortic phantom showed that the stent distended the lumen. No flow defect, luminal narrowing, or occlusion of side branches were noted.
After stenting, injection of methylin blue in the circuit resulted in a violet coloration of the aorta. Stent positioning and absence of endoleak were demonstrated by the absence of violet coloration outside the graft in the aneurismal sac (Fig. 3c).
4 Discussion
The results from this study demonstrate that rapid vessel prototyping can successfully be used to create vascular geometries as 3D MRA into a representation with large anatomical coverage, and test endovascular procedure.
4.1 Image processing
The Maracas software [15] extracts the vessel boundaries and thus supplies automatic measurements for accurate quantitative analysis of vascular morphology. From the detected lumen boundaries, several values – diameter (maximum, minimum, and average), perimeter, and area – can be deduced, enabling automatic one-click aorta segmentation and STL conversion. The vessel axis centered in the lumen indicates the true blood-flow path and can be used for guiding virtual endoscopic exploration of the vessel, but presents significant curvature in the regions adjacent to the pathological segment. Our axis extraction method differs from other vascular network extraction techniques founded on second derivatives [16,17] or on curvatures [18], which have been shown to be noise-sensitive. Our technique includes reducing noise-sensitivity so as to cope with even very small and low-contrast vessels. Due to its expansible nature, the centerline extraction algorithm overcomes the initialization limitations of deformable models as well as some of the geometric flexibility constraints such as adaptation to significant curvature. It therefore gives satisfactory results in terms of vessel tracking even in complex geometric situations.
Visually, the results were satisfactory for large as well as for small and low-intensity vessels. The vessel centerline correctly tracked the vessel path and lay inside it even in the presence of severely deformed arteries (aneurysm or stenosis). It could, however, be argued that in some cases the axis should lie outside the actual lumen. In MR angiography, only the vessel lumen is imaged. Therefore, the vessel axis extracted by our algorithm is in fact the circulating blood lumen centerline.
Other examples for model generation based on CT scan data have already been reported in the literature [12,14]. For computer model generation alone there is no clear advantage of MRI over CT scan; however, MRI offers the possibility to acquire additional information such as blood flow in the model [13] while being strictly non-invasive (no radiation effects).
4.2 Stereolithography
Rapid prototyping can be considered as a revolution in imaging techniques in medicine. Stereolithographic biomodeling has already been used in craniomaxillofacial surgery for the management of deformities, trauma, and tumor [19,20]. Benefits include better understanding of the surgical anatomy, better surgical planning with shorter operative times, and improved outcome. Test-bench pressure measurements using these elastic models should provide significant insight into the mechanisms underlying endoleaks.
The goal of the present study was to provide a more suitable experimental model for interventional radiology than the traditional rigid glass model, and one that would also assist in the choice of stent type and design.
Several fluid dynamics studies [21–23] have shown that vessel models can be conveniently used to evaluate vascular imaging techniques [24], flow measurements, and endovascular treatment strategies [25]. Most previous studies have been carried out on models obtained using injection molds based on normal or diseased arteries [21–23], computer-assisted technology [25], or plastic tubes [24]. The simulator proposed by Chong et al. [25] is a rigid model composed of solid silicone poured around a replica. The present model had the advantages of being life-size, transparent, and non-rigid and its originality is that it is derived directly from living patients.
However, this type of process is well adapted to large- and middle-size arteries but not to small vessels. Furthermore, only one type of silicone with visco-elastic properties similar to a normal vessel can be achieved using the stereolithography technique. We could not replicate the variations in visco-elastic properties of calcified or thrombosed atherosclerotic thoracic aneurysms.
The nature of the fluid used during testing was important. Plasma substitute was not mandatory in testing for endoleaks, and we preferred normal water as perfusate, adding 1 ml of Azorubine (Gifrer, France) to get a red-colored and radio-opaque fluid (Fig. 3c). Methylin blue was added secondarily in the circuit, resulting in a violet coloring of the aorta, clearly demonstrating the absence of endoleak by means of the color video camera.
4.3 Endovascular procedure
Inclusion in a flow-pulse simulator was used to simulate the in vivo situation in this transparent model, thereby providing a more realistic endovascular training device for the purposes of interventional radiology and surgery certification.
The Valiant system used in the model was shown to be more conformable than earlier generation devices; the new delivery system enables precise transluminal stent deployment. This new system significantly reduces the amount of force needed for deployment, increasing accuracy in positioning. It also offers a better variety of size and end configuration. Another option enables better matching of the stent to the anatomy of the lesion (Fig. 3b). The catheter section of the Xcelerant Delivery System tapers to a thinner diameter near the handle, letting it pass through narrow vessels more easily for precise stent positioning. The new system also offers both closed web and FreeFlo proximal end configurations, allowing the most appropriate stent to be selected for the type of lesion, with improved stent matching to the anatomy of the patient.
Three types of endoleak can be detected – mainly type I (where blood flows into the aneurysm sac due to an incomplete seal or an ineffective seal at the end of the graft, this type of endoleak usually occurs in the early course of treatment) and to a lesser extent types III and IV (type III endoleak is the blood flow into the aneurysm sac due to inadequate or ineffective sealing of overlapping graft joints or rupture of the graft fabric; type IV is the blood flow into the aneurysm sac due to the porosity of the graft fabric, causing blood to pass through from the graft and into the aneurysm sac). Type II endoleak cannot be detected because of the absence of collateral vessels.
This model cannot be used (for the time being) for the training in frozen elephant trunk technique (a hybrid vascular graft consisting of a conventional tube graft with an endovascular stented graft at the distal end is used to achieve a blood-tight seal in the descending aorta that cannot easily be accessed directly from an anterior approach).
The in vitro insertion of stentgraft into the aortic arch is furthermore reproducible and the procedure can be performed after removing the stentgraft from the replica with the aid of lubricant.
This model will be used in our department for in vitro training of young interventional radiologists and surgeons prior to in vivo application.
In future models, we are planning to reproduce different vascular pathologies including different forms and locations of aneurysms, and areas with thrombus, dissection, and stenosis, for preoperative determination of stent size and shape, and models adaptable for frozen elephant trunk technique.
5 Conclusion
Using computer-aided design techniques, the creation of an accurate replica of human arterial pathology from a live patient is feasible; the replicas created allow a physiologically accurate flow dynamics study of the altered anatomy. In addition, being able to hold a wax replica of the pathology in ones hand, rotating it in any direction wanted, allows both surgeons and interventional radiologists to optimize treatment approach and anticipate problems. Also, this 3D model gives a realistic impression of the complex underlying structure and is useful as an experimental model for the assessment of new stents and materials, and for training young surgeons and radiologists.
Acknowledgement
The authors thank Alain Bliez from Medtronic for the aortic stent.