-
PDF
- Split View
-
Views
-
Cite
Cite
Hrvoje Gasparovic, Stjepko Plestina, Zeljko Sutlic, Ino Husedzinovic, Vedran Coric, Visnja Ivancan, Ivan Jelic, Pulmonary lactate release following cardiopulmonary bypass, European Journal of Cardio-Thoracic Surgery, Volume 32, Issue 6, December 2007, Pages 882–887, https://doi.org/10.1016/j.ejcts.2007.09.001
- Share Icon Share
Abstract
Objective: The etiology of lung injury following cardiopulmonary bypass (CPB) is multifactorial. Our study focused on quantifying the lactate release from the lungs precipitated by extracorporeal circulation at different time points after the insult. This was complemented by an evaluation of the gas exchange at the level of the alveolar–capillary membrane. Methods: Forty consecutive patients (age 61 ± 11 years, EuroScore 4.7 ± 2.7) undergoing CABG were prospectively analyzed. The data are presented as medians and the interquartile range. Results: The pulmonary lactate release (PLR) increased from a baseline value of 0.033 (range −0.077 to 0.170) to 0.465 mmol/min/m2 (range 0.113–0.922), which was seen 6 h postoperatively (P < 0.001). The A-a O2 gradient increased from 12.7 (range 8.8–15) to 39.1 kPa (range 30.3–46.5) upon discontinuation of CPB (P < 0.001). The systemic arterial lactate (LS) concentration increased from 1.22 (range 1–1.44) to 3.03 mmol/l (range 2.29–4.76) 6 h after surgery (P < 0.001). The veno-arterial pCO2 difference (V-A dpCO2) rose from 0.6 (range 0.5–0.9) to 0.9 kPa (range 0.7–1) (P = 0.014). The mortality in the studied group was 5% (2/40). Conclusions: The lungs were found to be a significant source of lactate, and this pulmonary lactate flux was accentuated by CPB. The PLR correlated with systemic hyperlactatemia as well as the A-a O2 gradient, and was found to be higher in patients requiring prolonged mechanical ventilatory support. The duration of CPB had a significant impact on the systemic lactate concentrations, V-A dpCO2 and the A-a O2 gradient, but not on the PLR.
1 Introduction
Pulmonary dysfunction after cardiac surgery remains a frequent problem that may have a profound influence upon patient recovery. The wide spectrum of manifestations ranges from mild subclinical dysfunction to full-blown adult respiratory distress syndrome [1].
Multiple factors may precipitate acute lung injury following cardiopulmonary bypass, but the systemic inflammatory response is likely one of the most prominent causes [2]. Preoperative factors affecting the evolution of postoperative pulmonary dysfunction are: chronic obstructive pulmonary disease, smoking, and respiratory muscle wasting. Multiple features of the surgical procedure employing cardiopulmonary bypass harbor the potential to precipitate pulmonary injury. Hemodilution, reduction in the plasma oncotic pressure, and left atrial pressure elevation associated with weaning from cardiopulmonary bypass (CPB) contribute to the accumulation of fluid in the lung interstitium [3,4]. The lungs are not immune to the effects of cytotoxic mediators, emboli, or reperfusion injury induced by CPB. Furthermore, lung perfusion is altered in a way that it is solely dependant upon bronchial circulation, since the flow through the pulmonary artery is virtually nonexistent. The complex disruption of pulmonary homeostasis may be further enhanced by ventilator-associated lung injury.
The pulmonary dysfunction may manifest itself by a widening of the alveolar–arterial oxygen gradient (A-a O2 gradient), increase in pulmonary vascular resistance (PVR), or changes in the pulmonary shunt [2]. The most malignant form of CPB-induced pulmonary dysfunction is the adult respiratory distress syndrome (ARDS). The incidence of ARDS following cardiac surgery is below 2% [1,5,6].
Serum lactate concentrations have been used as markers of systemic hypoperfusion in a variety of clinical settings, including the cardiac surgical patient population [7–11]. The etiology of hyperlactatemia following cardiopulmonary bypass, however, remains controversial. While the pathogenesis of type A lactic acidosis is unequivocally linked to hypoperfusion, type B lactic acidosis, which occurs in patients in whom oxygen delivery is not impaired, may also occasionally be seen in patients undergoing CPB [12,13]. The lungs have previously been shown to be a source of lactate in the setting of lung injury, thereby producing a positive transpulmonary lactate gradient [14]. The veno-arterial difference in the partial pressure of carbon dioxide (V-A dpCO2) has been instrumental in demonstrating hypoperfusion in several published studies [15,16].
Our study aimed to test the hypothesis that cardiopulmonary bypass will have a significant impact on the pulmonary production of lactic acid, as well as compromise the functional integrity of the alveolar–capillary membrane. Furthermore, the pulmonary lactate release and the A-a oxygen gradient were documented at different time points in relation to the surgical insult in order to provide a chronological insight into the evolution of these markers of lung injury.
2 Patients and methods
Following the approval from our institutional ethics committee, 40 consecutive patients undergoing coronary artery bypass grafting using cardiopulmonary bypass (CABG) were enrolled into our study. Informed consent was obtained from all patients. The study was conducted in a prospective observational fashion. Exclusion criteria were concomitant valvular pathology requiring surgery and reoperative surgical myocardial revascularization.
2.1 Perioperative management
The patients received diazepam and morphine 30 min prior to induction of anesthesia. Endotracheal tube, urinary catheter, as well as radial artery and pulmonary artery catheters were inserted. The anesthetic regime included induction and maintenance of anesthesia with midazolam, fentanyl, and pancuronium bromide. This was coupled with sevoflurane inhalation. The initial ventilator settings included a tidal volume of 8 ml/kg and a respiratory rate of 12 breaths per min. Typically, the FiO2 was set at 50%. The critical components of the employed cardiopulmonary circuit were the Medtronic Affinity Trillium membrane oxygenator, venous reservoir and PVC tubing (Medtronic, Minneapolis, USA), and a Stoeckert III roller pump (Stoeckert, Munich, Germany). Systemic heparinization aiming at an ACT > 480 s was used, followed by full reversal with protamine after decannulation. Tepid CPB was employed, targeting the flow at 2.2 l/min/m2. The target mean arterial pressure during CPB was 60 mmHg. If necessary, norepinephrine was employed to reach the aimed blood pressure. The surgical myocardial revascularization was performed on an arrested heart, during a period of aortic cross-clamping. The lungs were open to atmosphere during CPB. Weaning from CPB was initiated once the patient’s rhythm had stabilized and normothermia had been achieved. Inotropic support was initiated in order to maintain a cardiac index greater than 2.2 l/min/m2. The preferred inotropic agent was dobutamine. Norepinephrine was used if dobutamine produced excessive vasodilatation. Epinephrine was used if the hemodynamic performance remained inadequate with the previously mentioned catecholamines. An intra-aortic balloon pump was inserted if further support was required.
2.2 Measurement and calculations


The effects of hemodilution were taken into account in order to obtain a hemoglobin-adjusted pulmonary lactate release as was suggested by Kellum et al. [14]. The A-a oxygen gradient was obtained by subtracting the partial pressure of oxygen in arterial blood from the calculated alveolar oxygen tension [17]. The formulas for calculating the A-a oxygen gradient are shown here:


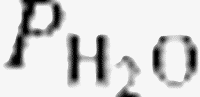
One sample of arterial blood was analyzed at any given time point to obtain the A-a oxygen gradient. Individual A-a oxygen gradients were recorded for every patient at every time point. The data from all of the patients were then pooled and analyzed in order to obtain median values for the whole cohort at every preset time point (T1–T4). The initial FiO2 was 0.5 for the samples taken prior to the surgical incision. The subsequent fraction of inspired oxygen was dictated by each patient’s present partial pressure of oxygen in arterial blood. This value of FiO2 was then incorporated into the calculation for the corresponding A-a oxygen gradient. Only one FiO2 value was used for one time point, i.e. no attempt to investigate the effects of different ventilation settings on the A-a oxygen gradient was made.
The veno-arterial pCO2 difference was obtained by subtracting the partial pressure of CO2 in radial arterial blood from the partial CO2 pressure measured in the pulmonary artery.
2.3 Statistical analysis
The data are presented as mean values ± standard deviation or medians followed by the interquartile range for variables with a distribution that is not normal. Longitudinal comparisons among samples of the same subject were analyzed using the ANOVA test. Correlations were analyzed using the nonparametric Spearman R correlation methodology. Analyses between different groups of patients were performed using the Mann–Whitney U-test. A P < 0.05 was considered to be of statistical significance. The data were processed using the Statistica software package (StatSoft Inc., Tulsa, USA).
3 Results
3.1 Perioperative summary
Forty consecutive patients undergoing coronary artery bypass grafting were enrolled into our study. The patient demographic data are presented in Table 1 . The spectrum of comorbidities seen in our patient population is typical for the contemporary cardiac surgical practice.
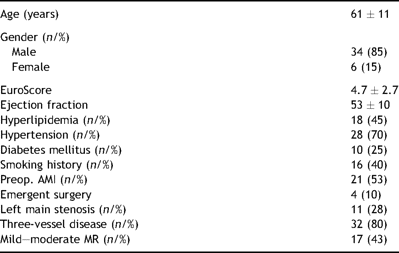
The patient population profile included both elective and non-elective cases. There were four patients in whom the operation was performed on an emergent basis, related to complications of percutaneous coronary interventions. All of these had acute coronary syndromes noted prior to surgery. Two of these patients were found to be in significant hemodynamic compromise when referred to our tertiary care center from outside hospitals. Two patients had intra-aortic balloon pumps placed preoperatively. The mortality was 5% (2/40). One of these patients died due to left ventricular free wall rupture on postoperative day 14, whereas the second death in this cohort was stroke related. The perioperative summary is presented in Table 2 .
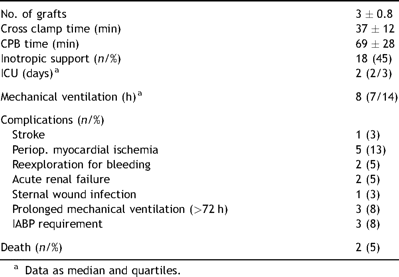
Perioperative patient data presented as mean values ± standard deviation, unless otherwise indicated
The median values of the pulmonary lactate release increased from a baseline value of 0.033 (range −0.077 to 0.170) to 0.465 (range 0.113–0.922) observed 6 h after surgery (P< 0.001). The transpulmonary lactate gradient (TPLG) increased from the preoperative 0.014 (range −0.034 to 0.085) to 0.163 mmol/l (range 0.041–0.249) calculated from the samples taken 6 h after surgery (P = 0.001).
The A-a O2 gradient increased from a baseline value of 12.7 (range 8.8–15) to 39.1 kPa (range 30.3–46.5) immediately upon discontinuation of cardiopulmonary bypass (P < 0.001). The total arterial lactate content measured in the radial artery increased from 1.22 (range 1–1.44) to 3.03 mmol/l (range 2.29–4.76) 6 h postoperatively (P < 0.001). The serum lactate concentration did not reach preoperative values by the end of the study protocol. A rise in the veno-arterial pCO2 gradient (V-A dpCO2) from 0.6 (range 0.5–0.9) to 0.9 kPa (range 0.7–1) at 6 h postoperatively was also found to be statistically significant (P = 0.014). This peak corresponded to the simultaneous peak in the systemic lactate concentration. There was no statistical significance to the dynamics of the pulmonary vascular resistance throughout the study period. The evolution of the pulmonary lactate flux, transpulmonary lactate gradient, A-a O2 gradient, total arterial lactate, veno-arterial pCO2 difference, and PVR from the values obtained prior to CPB to those found at 18 h postoperatively are presented in more detail in Table 3 .
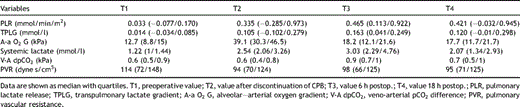
3.2 Clinical correlations
The amplification of the pulmonary lactate release correlated with the simultaneously observed increase in the systemic arterial lactate concentration 6 h (T3) after surgery. The PLR calculated from samples immediately upon discontinuation of CPB (T2) correlated with the appropriate A-a O2 gradient. The peak PLR also correlated with the A-a O2 gradient observed 18 h after surgery. The PLRT4 correlated with the veno-arterial pCO2 difference, which served as another index of systemic hypoperfusion. A lower A-a O2 gradient prior to surgery was predictive of quicker extubation. The alveolar–arterial oxygen gradient seen at 18 h correlated with the duration of cardiopulmonary bypass. The A-a oxygen gradientT4 correlated with the corresponding pHT4 in addition to having an inverse correlation with the partial pressure of carbon dioxide in arterial blood sampled at the same time (T4). The peak increase in the systemic arterial lactate concentration (T3) correlated with the duration of CPB as well as the duration of mechanical ventilation. Additionally, the duration of CPB influenced the PVR and V-A dpCO2 18 h after CABG. The correlations between the above-noted measurements and clinical parameters are presented in greater detail in Table 4 .
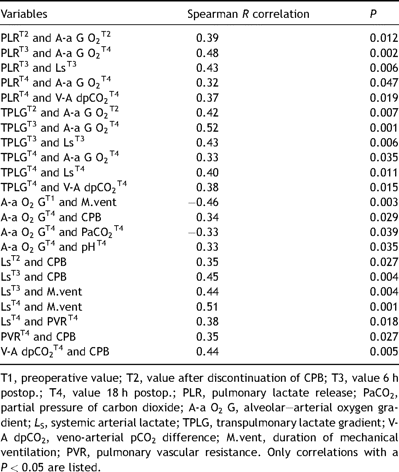
Clinical relevance of laboratory data tested with a nonparametric correlation analysis
The subgroup of patients in whom mechanical ventilation for more than 72 h was required had a higher pulmonary lactate release 6 h after surgery in comparison to patients weaned from the ventilator before that time (5.15 (range 0.991–5.956) vs 0.392 mmol/min/m2 (range 0.096–0.766), Mann–Whitney U-test, P = 0.005). Additionally, this subgroup of patients had higher A-a O2 gradients at 18 h after surgery (32.5 (range 24.1–33.5) vs 17.6 (range 11.3–20.5), P < 0.001), higher serum lactate concentrations at T3 and T4 (13.63 (range 8.36–15.4) vs 2.85 mmol/l (range 2.27–4.04), P < 0.001, and 7.42 (range 4.26–8.2) vs 1.97 mmol/l (range 1.3–2.71), P < 0.001, respectively) as well as higher pulmonary vascular resistance 6 h after surgery (172 (range 121–200) vs 92–dyne s/cm5 (range 65–123), P = 0.048). Higher EuroScore and CPB times were also noted in patients requiring prolonged ventilatory support (9 ± 1 vs 4.4 ± 2.5, P = 0.008; and 105 ± 37 vs 66 ± 26 min, P = 0.035). Patients in whom an intra-aortic balloon pump was inserted had higher peak lactate levels (8.73 (range 5.28–15.4) vs 2.85 (range 2.27–4.04), P = 0.005).
4 Discussion
The genesis of respiratory complications following cardiac surgery is complex and may stem from inherent pulmonary disease, depressed cardiac function with resultant pulmonary edema, post-perfusion inflammatory response, or inadequate diaphragmatic function associated with phrenic nerve injury [2,5]. The functional and clinical changes have been corroborated by histological evidence of lung injury consisting of alveolar edema, extravasation of red blood cells and neutrophils, as well as necrosis and swelling of pneumocytes and endothelial cells [1].
Cardiopulmonary bypass is responsible for the production and release of a plethora of markers, which serve to quantify both local organ dysfunctions and systemic perfusion aberrancies.
Our study focused on the lactate release from the lungs and the dysfunction at the level of the alveolar–capillary membrane precipitated by extracorporeal circulation in patients undergoing surgical myocardial revascularization. In addition, we looked at systemic hypoperfusion indices, such as systemic lactate concentration and veno-arterial pCO2 gradient, and their correlation to cardiopulmonary bypass.
We have demonstrated a modest pulmonary lactate production in our patient population even at baseline, i.e. the lungs were a source of lactate even in the absence of a notable surgical insult. The pulmonary lactate contribution rose significantly upon exposure to surgical trauma and cardiopulmonary bypass. The occurrence of a positive transpulmonary lactate gradient after CPB has been corroborated by other authors [14,18].
The values continued to rise up to 6 h after the procedure, implying that the lactate washout from the lungs may be a protracted process. The clinical impact of the pulmonary lactate release was illustrated in its correlation with the need for prolonged mechanical ventilatory support. There was no correlation between the PLR and the duration of CPB. While the lactate produced in the lungs was not the sole factor responsible for systemic hyperlactatemia, it significantly contributed to the systemic arterial lactate content measured 6 h after the procedure. The dramatic augmentation of the alveolar–arterial oxygen gradient after surgery may corroborate the hypothesis that cardiopulmonary bypass caused a complex disturbance at the level of the alveolar–capillary membrane. One must, however, recognize that the presence of atelectasis after resumption of ventilation may have also contributed to this finding. Persistent widening of the alveolar–arterial O2 gradient was indicative of the requirement for prolonged mechanical ventilation. We were able to show a statistically significant correlation between the A-a O2 gradient and the PLR after weaning from CPB. Arterial hyperlactatemia reflects the undesirable effects of CPB at the systemic level. We have demonstrated a correlation of the peak systemic lactate concentration with the duration of CPB and mechanical ventilation. Not surprisingly, patients in whom an intra-aortic balloon pump for mechanical circulatory support was inserted had higher peak lactate levels. One should not neglect the possible confounding effect of catecholamine administration on the observed hyperlactatemia. Surprisingly, however, we found no statistically significant correlation between the use of inotropic support and systemic lactate concentrations. The evidence of global hypoperfusion during CPB in our study also included widening of the difference between the mixed venous and arterial pCO2 difference. The V-A dpCO2 correlated with the duration of CPB.
We did not maintain pulmonary artery (PA) perfusion during cardiopulmonary bypass in our patients, nor were the lungs ventilated. Maintaining physiologic pulmonary perfusion has been advocated by some authors in order to preserve the pulmonary clearance of certain proinflammatory cytokines [1]. The PA perfusion strategy has been linked to superior postoperative respiratory function in certain clinical scenarios, possibly due to attenuation of the ischemia–reperfusion injury, but has yet to gain wider acceptance [19,20]. The higher mortality and morbidity seen in our cohort is secondary to the patient profile referred to our center that accepts patients suffering from various complications from smaller regional hospitals, and is also reflective of the prospective nature of this study. Four patients in this cohort were referred for surgery suffering from various degrees of myocardial ischemia and hemodynamic compromise due to either failed PCI in the setting of an acute coronary syndrome or complications thereof.
There are certain limitations in this study that warrant mentioning. The pulmonary lactate release was calculated from the lactate gradient between the afferent and efferent circulations in relation to the lungs. The efferent sample, however, was not taken from the pulmonary veins but rather from the radial artery. Theoretical concern could therefore be raised whether shunting through Thebesian veins could compromise the validity of the data. This issue, however, has been addressed in the past and the mixed venous sample has been utilized for quantification of the pulmonary lactate production in several studies [14,18,21]. Additionally, the sample size is relatively small; with the subgroup of patients requiring still smaller prolonged ventilatory support.
In summary, our study is the first to offer a chronological perspective on the pulmonary lactate release after cardiopulmonary bypass in a relatively homogenous population of patients. The main findings of this study are that the pulmonary lactate release is accentuated by the surgical procedure employing cardiopulmonary bypass and that a higher PLR is predictive of prolonged mechanical ventilation. It also showed a correlation between the PLR and the disturbance at the level of the alveolar–capillary membrane, as well as the impact of pulmonary lactate production on systemic hyperlactatemia. While there is no doubt that cardiopulmonary bypass induces a violent disturbance of the normal cardiovascular physiology, one should not rush to condemn it as the sole etiologic factor responsible for the development of pulmonary dysfunction. One must also stress that the amplitude of pulmonary lactate production in our study is much less pronounced than that observed in patients with profound respiratory insufficiency documented in another study encompassing a wide range of etiologies [14].