-
PDF
- Split View
-
Views
-
Cite
Cite
Caroline Van De Wauwer, Arne P. Neyrinck, Nele Geudens, Filip R. Rega, Geert M. Verleden, Erik Verbeken, Toni E. Lerut, Dirk E.M. Van Raemdonck, Retrograde flush following topical cooling is superior to preserve the non-heart-beating donor lung , , European Journal of Cardio-Thoracic Surgery, Volume 31, Issue 6, June 2007, Pages 1125–1133, https://doi.org/10.1016/j.ejcts.2007.01.070
- Share Icon Share
Abstract
Objective: The use of non-heart-beating donors (NHBD) has been propagated as an alternative to overcome the scarcity of pulmonary grafts. Formation of microthrombi after circulatory arrest, however, is a major concern for the development of reperfusion injury. We looked at the effect and the best route of pulmonary flush following topical cooling in NHBD. Methods: Non-heparinized pigs were sacrificed by ventricular fibrillation and divided into three groups (n = 6 per group). After 1 h of in situ warm ischaemia and 2.5 h of topical cooling, lungs in group I were retrieved unflushed (NF). In group II, lungs were explanted following an anterograde flush (AF) through the pulmonary artery with 50 ml/kg Perfadex® (6 °C). Finally, in group III, lungs were retrieved after an identical but retrograde flush (RF) via the left atrium. Flush effluent was sampled at intervals to measure haemoglobin concentration. Performance of the left lung was assessed during 60 min in our ex vivo reperfusion model. Wet-to-dry weight ratio (W/D) of both lungs was calculated as an index of pulmonary oedema. IL-1ß and TNF-α protein levels in bronchial lavage fluid from both lungs were compared between groups. Results: Haemoglobin concentration (g/dl) was higher in the first effluent in RF versus AF (3.4 ± 1.1 vs 0.6 ± 0.1; p ≪ 0.05). Pulmonary vascular resistance (dynes × s × cm−5) was 975 ± 85 RF versus 1567 ± 98 AF and 1576 ± 88 NF at 60 min of reperfusion (p ≪ 0.001). Oxygenation (mmHg) and compliance (ml/cmH2O) were higher (491 ± 44 vs 472 ± 61 and 430 ± 33 NS, 22 ± 3 vs 19 ± 3 and 14 ± 1 NS, respectively) and plateau airway pressure (cmH2O) was lower (11 ± 1 vs 13 ± 1 and 13 ± 1 NS) after RF versus AF and NF, respectively. No differences in cytokine levels or in W/D ratios were observed between groups after reperfusion. Histology demonstrated microthrombi more often present after AF and NF compared to RF. Conclusion: Retrograde flush of the lung following topical cooling in the NHBD results in a better washout of residual blood and microthrombi and subsequent reduced pulmonary vascular resistance upon reperfusion.
1 Introduction
The scarcity of suitable donor organs is the main limiting factor for widespread application of lung transplantation. Only 15–30% of the brain dead donors have lungs that are deemed transplantable [1]. As a result of the disparity between the growth in demand and the inadequate organ supply, a renewed interest in the use of lungs from non-heart-beating donors (NHBD) is emerging [2]. There is now experimental [3–6] and clinical [7,8] evidence that a limited period of warm ischaemia does not compromise the performance of the pulmonary graft from the NHBD and that topical cooling is an effective method to protect the pulmonary graft inside the cadaver [5].
The formation of microthrombi after circulatory arrest and the subsequent development of primary graft dysfunction resulting from ischaemia–reperfusion injury, however, are still a concern for the use of lungs from NHBD. From a recent study, we have data suggesting that retrograde flush of the lungs after 1 h of warm ischaemia is better to preserve graft performance compared to anterograde pulmonary flush or no flush [9]. However, no experimental data on the effect of pulmonary flush following additional topical cooling in the cadaver are available in the literature.
The aim of this study was to investigate the benefit and the most effective route (anterograde vs retrograde) of pulmonary flush following topical cooling after warm ischaemia using our isolated porcine lung reperfusion model.
2 Material and methods
2.1 Animal preparation
Domestic pigs (n = 6 per group; weight: 37.2 ± 1.1 kg) were used, given their physiological and anatomical similarity to man. All animals received humane care in compliance with the Principles of Laboratory Animal Care, formulated by the National Society for Medical Research and the Guide for the Care and Use of Laboratory Animals, prepared by the Institute of Laboratory Animal Resources, National Research Council, and published by the National Academy Press, revised 1996 (NIH Publication No. 85-23, Revised 1996). The study was approved by the institutional review board on animal research at the Katholieke Universiteit Leuven.
Animals were premedicated with an intramuscular injection of Xylazine (5 ml Xyl-M® 2%, V.M.D. nv/sa, Arendonk, Belgium) and Zolazepam/Tiletamine (3 ml Zoletil® 100, Virbac s.a., Carros, France). The animals were installed in a supine position and intubated with an endotracheal tube 7.5 (Portex Tracheal Tube, SIMS Portex, Ltd., Hythe, Kent, UK) and ventilated with a volume-controlled ventilator (Titus®, Dräger, Lübeck, Germany) with an inspiratory oxygen fraction (FiO2) of 0.5 and a tidal volume of 10 ml/kg body weight. Respiratory rate was adjusted to achieve an end-tidal CO2 of 40 mmHg. Positive end-expiratory pressure was set to 5 cmH2O. Anaesthesia was maintained with isoflurane 0.8–1% (Isoba® Vet, Schering – Plough Animal Health, Harefield, Uxbridge, UK) and muscle relaxation with intermittent boli of pancuronium bromide (Pavulon 2 mg/ml, Organon, Teknika, Boxtel, The Netherlands). A 14 G catheter (Secalon® T, Becton Dickinson Ltd., Singapore) was placed in the right common carotid artery for measurement of the systemic arterial pressure (SAP) and sampling of arterial blood. A 7.5 F Swan-Ganz thermodilution catheter (Baxter Healthcare Corp., Irvine, CA, USA) was inserted through the right external jugular vein into the pulmonary artery. With this catheter, haemodynamic parameters including pulmonary artery pressure (PAP) and pulmonary capillary wedge pressure (PCWP) were monitored. Haemodynamic parameters (SAP, PAP and PCWP) and aerodynamic parameters (plateau airway pressure and compliance) were continuously monitored and stored.
Pigs were sacrificed by inducing ventricular fibrillation with a subxyphoidal needle puncture using a square-pulse generator (amplitude range +15 to −15 V, current ≪300 mA, frequency 50 Hz). After cardiac arrest, the endotracheal tube was disconnected from the ventilator and left open to the air. The cadavers were left untouched for 1 h at room temperature followed by a 2.5-h interval of topical cooling (Fig. 1A ). Therefore, two chest drains were inserted in each pleural cavity (one superficial, one deep). Lungs were then cooled with cold saline in a closed circuit. To ensure that the lungs were well immersed in the fluid, the superficial drains were connected to an overflow system of 5 cmH2O. Temperature of the lung was measured via a probe in the endotracheal tube and rectal temperature was monitored. After that interval of topical cooling, sternotomy was performed. The thymic tissue was excised and the pericardium and pleural cavities were widely opened. The lungs were inspected. The pulmonary artery, ascending aorta and caval veins were encircled. Gross microthrombi in the pulmonary artery and left atrium were evacuated as much as possible.
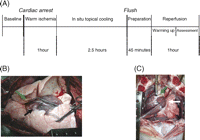
(A) Experimental protocol in all three study groups differing in flush: retrograde or anterograde or no flush. (B) Anterograde flush: a cannula is placed through the right ventricular outflow tract (black arrow) into the pulmonary artery isolated from the right ventricle by a ligature around the tip of the catheter (green arrow). (C) Retrograde flush: a cannula is placed in the left atrium through a purse-string (white arrow). The anterior aspect of the main pulmonary artery is incised for drainage of the flush solution (green arrow).
2.2 Experimental groups
Eighteen domestic pigs were randomly divided into three groups (n = 6 per group; weight: 37.2 ± 1.1 kg). In the first group the lungs were explanted without flush (NF). In the second group the pulmonary artery was cannulated (DLP Inc., Grand Rapids, MI, USA) through the right ventricular outflow tract and secured with a purse-string. The caval veins were ligated and the ascending aorta was clamped. The pulmonary artery was isolated from the right ventricle by a ligature around the tip of the catheter just distal to the pulmonary valve. The right and the left atria were incised for venting of the heart. The lungs were flushed in an anterograde (AF) (Fig. 1B) way with 50 ml/kg cold (6 °C) Perfadex® (Vitrolife, Göteborg, Sweden) buffered with Trometamol (0.3 ml/l, 2 g/5 ml, Addex-THAM) and CaCl2 (0.6 ml/l, 11 meq). During the flush, ventilation was restarted with a low tidal volume and a small frequency to avoid cold lung injury related to mechanical stress. Finally, in the third group the left atrium was cannulated (MOD Canule 18 Fr, International Medical Products NV/SA, Brussels, Belgium) through a purse-string and the lungs were flushed in an identical but retrograde manner (RF) (Fig. 1C). The anterior aspect of the main pulmonary artery was incised for drainage of the flush solution. The perfusion pressure during AF and RF was maintained at 15 mmHg by adjusting the height of the perfusion bag. During AF and RF, samples were taken from the outflowing flush solution at different time points (0, 30, 60, 90, 120, 150 s after the start of the flush) for measurement of the haemoglobin concentration.
2.3 Preparation of the heart–lung block
The lungs in all three groups were then explanted and prepared in the same way for ex vivo evaluation in the isolated reperfusion system. The right lung was separated from the heart–lung block and used as a control for morphological and biochemical analysis. The pulmonary artery was cannulated through the right ventricular outflow tract using a 36 Fr cannula and isolated with a ligature around the catheter distal to the pulmonary valve. A small catheter was placed in the pulmonary artery for measurement of PAP. The ascending aorta was clamped. The left atrium was cannulated through the apex of the left ventricle with a second 36 Fr cannula and secured with a purse-string. Finally, an endotracheal tube nr. 8 was placed in the trachea for ventilation of the graft.
2.4 Preparation of the perfusate
Autologous blood (1200 ml) was rapidly withdrawn from each animal at the moment of sacrifice via the catheter in the right external jugular vein and collected in a sterile bag containing 5000 IU of heparin (Natrium Heparine B. Braun, 25000 IU/5 ml, B. Braun Medical SA, Jaén, Spain). This whole blood was centrifuged with a Cell Saver (Sequestra 1000, Medtronic Inc., Parker, CO, USA) and washed with saline for 12 min at 5600 rpm. Leukocytes were sequestered using a leukocyte filter (Imugard III-RC, Terumo Europe N.V., Haasrode, Belgium). The remaining red blood cells (350 ml) were then diluted to a haematocrit of 15% with a low potassium dextran solution (Perfadex®) and human albumine (final concentration: 8%, CAF-DCF, Brussels, Belgium). The perfusate was finalized by adding CaCl2 (2.4 ml/l, 100 mg/ml), heparin (10000 IU/l) and sodium bicarbonate (45 ml/l, 16.8 g/250 ml, Baxter, Lessines, Belgium). The total volume of the perfusate was 1400 ml.
2.5 Isolated reperfusion circuit
The ex vivo reperfusion system consisted of a hardshell reservoir (Minimax® Hardshell reservoir, Medtronic, Minneapolis, MN, USA), a centrifugal pump (Bio-medicus, Medtronic), a heater/cooler system (Bio-Cal, Heater Cooler Model 370, Medtronic, Minneapolis, MN, USA) and a hollow fibre oxygenator (Capiox®SX, Terumo, MI, USA) with integrated heat exchanger. The heating element of the gas exchanger was connected to the heater/cooler system. The left lung and the heart were then placed in a specially designed evaluation box and mounted in the reperfusion system. The cannula in the pulmonary artery was connected to the inflow tubing and the outflow tubing was connected to the cannula in the left atrium.
2.6 Technique of controlled reperfusion and ventilation
Reperfusion of the left lung was started with normothermic (37 °C) oxygenated perfusate (O2: 0.4 l/min) after de-airing of the inflow tubing. Pulmonary artery pressure was gradually increased to a maximum of 15 mmHg and the left atrial pressure on the outflow was kept at 0 mmHg by adjusting the height of the blood reservoir. This resulted in warming up of the lung and a gradual increase in pulmonary artery flow (PAF). Ventilation with a FiO2 0.5 was started when the temperature of the outflowing perfusate reached 34 °C and slowly increased to a tidal volume of 140 ml, a frequency of 14 breaths/min and PEEP of 5 cmH2O. At that moment, the perfusate was partially deoxygenated to a PO2 of 50–60 mmHg with a gas mixture of CO2 (8%), O2 (6%) and N2 (86%).
2.7 Assessment of the graft
Thirty-five minutes after the onset of reperfusion the temperature of the lung parenchyma reached 37.5 °C. At this moment functional graft parameters were recorded up to 1 h (Fig. 1). Pulmonary artery pressure (mmHg) was measured via an 18 Gauge catheter inserted in the main pulmonary artery. The pressure in the left atrium (LAP) (mmHg) was measured on the outflow line. An electromagnetic flow probe (FF 100T 10 mm probe, Nihon Kohden, Tokyo, Japan) was inserted in the tubing on the inflow line for continuous measurement of the pulmonary artery flow (l/min). Pulmonary vascular resistance (PVR) was calculated using the formula: PVR = [(PAP − LAP) × 80]/PAF and expressed in dynes × s × cm−5. Dynamic lung compliance (Compl) (ml/cmH2O) and plateau airway pressure (Plat AwP) (cmH2O) were recorded. PO2 and PCO2 were continuously measured in the perfusate via probes (Terumo CDI™, 500 shunt sensor, Leuven, Belgium) on the outflow tubing using an inline blood gas analyser (CDI™ 500, Terumo, Borken, Germany). Oxygenation capacity was calculated using the formula PO2/FiO2 (mmHg).
Temperature (°C) of the inflowing and outflowing perfusate was continuously measured, the last being considered as the graft temperature. All data were recorded online and stored on a central server (Datex AS/3 and S5 collect 3.0 Software, respectively, Datex-Ohmedia, Helsinki, Finland).
At the end of the reperfusion, both right and left lungs were dried in an oven at 80 °C for 48 h to a constant weight and their wet-to-dry ratio (W/D) was calculated and used as a parameter of pulmonary oedema.
2.8 Measurement of IL-1ß and TNF-α
Bronchial lavage was performed in the right lung after explantation and in the left lung immediately at the end of the reperfusion. Twenty-five millilitre of sterile saline at room temperature was instilled in the bronchus and aspirated with gentle suction after 1 min in a standardized way. The returned fraction was centrifuged at 3500 rpm for 10 min at 4 °C. The supernatant was collected for further analysis and stored at −80 °C.
Swine IL-1ß and TNF-α protein levels were measured in bronchial lavage supernatant from both lungs using the commercially available ELISA kits (BioSource Europe SA, Nivelles, Belgium). The sensitivity was 15 pg/ml for IL-1ß and 3 pg/ml for TNF-α.
2.9 Histology
Tissue samples were obtained from the non-perfused right lung and from the perfused left lung. Specimens were fixed in 6% formaldehyde, dehydrated and stained with phosphotungstic acid–haematoxylin (PTAH) to detect fibrin deposits. Histological analysis was performed by one experienced pathologist (E.V.) who was blinded to the experimental set-up.
2.10 Statistical analysis
Data were analysed using Graphpad Prism 4 (San Diego, CA, USA). All data are expressed as mean ± standard error of the mean (SEM). Graft parameters between study groups were compared using a one-way analysis of variance with multiple comparisons. An unpaired t-test was used to look for significant differences in flush time and in haemoglobin concentration between AF and RF. A p-value ≪0.05 was considered as significant.
3 Results
3.1 Study groups
Baseline parameters in the three animal groups prior to sacrifice are listed in Table 1 . There were no significant differences in animal weight, PAP, Plat AwP, Compl and PaO2/FiO2 between the three groups.
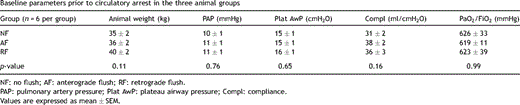
Baseline parameters prior to circulatory arrest in the three animal groups
Graft characteristics in the three study groups are compared in Table 2 . There were no statistically significant differences among the three groups regarding warm and cold ischaemic intervals, graft temperature at the end of the cold ischaemic period and time needed to complete the flush. Warming up the lung to 34 °C in the ex vivo circuit took significantly longer in NF compared to RF and AF (p = 0.011).
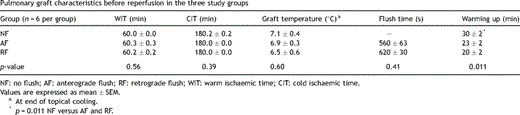
Pulmonary graft characteristics before reperfusion in the three study groups
3.2 Pulmonary graft function
3.2.1 Pulmonary vascular resistance
During the whole evaluation period, PVR (dynes × s × cm−5) of the left lung was lower in RF compared to AF and NF becoming significant at 50 min (947 ± 67 vs 1614 ± 110 and 1665 ± 121, respectively; p = 0.0002) until the end of the reperfusion (975 ± 85 vs 1567 ± 97 and 1576 ± 88, respectively; p = 0.0003) (Fig. 2A ). There was no significant difference in PVR between AF and NF.
![Pulmonary graft function (mean ± SEM) during 60 min of reperfusion in an isolated circuit. NF: no flush; AF: anterograde flush; RF: retrograde flush. (A) Pulmonary vascular resistance [(*) p ≪ 0.001: RF vs AF, (+) p ≪ 0.001: RF vs NF]. (B) Dynamic lung compliance (NS). (C) Plateau airway pressure (NS). (D) Oxygenation capacity (PO2/FiO2) (NS).](https://oup.silverchair-cdn.com/oup/backfile/Content_public/Journal/ejcts/31/6/10.1016_j.ejcts.2007.01.070/1/m_1125.S1010794007001285.gr2.gif?Expires=1750244352&Signature=e0zIWihdX7EfRKqyz7Mykt6r3wvLP8E2Z-taHlQqtqVQcIF~M6qtgR3VFn5pXPiEdwEcbMycdz1ijCnfS312cW6acC7MNIXAZhsub9PBWtWqHTtbbyxPR3jhGHGAWQZsS5I~owzWWfDHCatUFzjx9SWBad-ZJhhpaHBmk74XaRIvA6wu5nPxulC3sgdVjEbwLbxNAhapfFCs26Z-g~svxbOKTc84kRPkELz-3XeYJwqJMGvKy4rP3Eq1khnAMQXyK3OMQgirVliDMVAzbZ9xLqd0WdIY4xMGEEYOFLev7z5irtNYSmfmm~7IWD48vJN8IOTVfSxc-nD8DupE9VBN8A__&Key-Pair-Id=APKAIE5G5CRDK6RD3PGA)
Pulmonary graft function (mean ± SEM) during 60 min of reperfusion in an isolated circuit. NF: no flush; AF: anterograde flush; RF: retrograde flush. (A) Pulmonary vascular resistance [(*) p ≪ 0.001: RF vs AF, (+) p ≪ 0.001: RF vs NF]. (B) Dynamic lung compliance (NS). (C) Plateau airway pressure (NS). (D) Oxygenation capacity (PO2/FiO2) (NS).
3.2.2 Dynamic lung compliance
During the assessment period, dynamic lung compliance (ml/cmH2O) was higher after RF compared to AF and NF (20 ± 3 vs 16 ± 3 and 13 ± 2 at 35 min; p = 0.26 and 22 ± 3 vs 19 ± 3 and 14 ± 1 at 60 min; p = 0.096, respectively) (Fig. 2B).
3.2.3 Plateau airway pressure
There were no significant differences in plateau airway pressure. However, Plat AwP (cmH2O) was lowest in RF compared to AF and NF (11 ± 0 vs 13 ± 1 and 13 ± 1 at 60 min; p = 0.38, respectively) (Fig. 2C).
3.2.4 Oxygenation capacity
During reperfusion, PO2/FiO2 (mmHg) was lower in RF compared to AF and NF at the start of the assessment. Towards the end, oxygenation capacity improved in RF. The difference between the three groups was not significant (Fig. 2D).
3.3 Wet-to-dry weight ratio
The W/D ratio in the non-perfused right lung and in the left lung after reperfusion is shown in Fig. 3 , respectively. There was no significant difference between the three groups prior (p = 0.12) and after (p = 0.27) reperfusion. W/D ratio in RF and AF, however, was significantly lower after reperfusion (left lung) than before reperfusion (right lung) (4.9 ± 0.1 vs 5.7 ± 0.1; p = 0.0011 and 5.0 ± 0.1 vs 5.6 ± 0.1; p = 0.0007, respectively). There was no significant difference in NF (5.2 ± 0.1 vs 5.5 ± 0.1; p = 0.13, respectively).
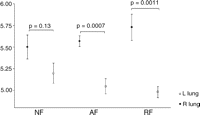
Wet-to-dry weight (W/D) ratio before (right lung, closed squares) and after reperfusion (left lung, open squares). There was no significant difference between the three groups. There was a significant difference between the right and left lung in AF and RF. NF: no flush; AF: anterograde flush; RF: retrograde flush.
3.4 Haemoglobin concentration
Haemoglobin concentration (g/dl) in the outflowing flush solution over time in RF and AF is depicted in Fig. 4 . At time point 0 the concentration was higher in RF compared to AF (3.4 ± 1.1 vs 0.6 ± 0.1; p = 0.04), but decreased quickly during the flush.
![Haemoglobin concentration (g/dl) in the outflowing flush solution. There was a significant difference between RF and AF at time point 0 [(*) p = 0.04]. AF: anterograde flush; RF: retrograde flush.](https://oup.silverchair-cdn.com/oup/backfile/Content_public/Journal/ejcts/31/6/10.1016_j.ejcts.2007.01.070/1/m_1125.S1010794007001285.gr4.gif?Expires=1750244352&Signature=4gNGsZoz9oIjpH9d971hE~51TRI8cdXGuRpVw1YHXE1EDklcMEVcb3~KWL7gJGX6KDavM~cRcxKXcMPk4ByPdzXZAPclOujEtW12bVbORGoJuszTO1e8U2Obg1LaRkmsi9z~VpGn4z8bNuDetgPQAW1OIf9jwE~b-scJcL5Ghu9bQb6DDe28deKKpFPUyqWVLvWPlM7X0YTTAjz~pfnhgTIqE-gmvotU~ZcxUuCVHYqvaqigL5e~ed1CYzM1NMhAXup8nPMUKDbrlyQd0Qxahvw0QG8jY8qjBv9GwPgdvFxZt6xufXu6QzcyjwEYPGtO35Mo6bO6aeWyOJwh0cHg1g__&Key-Pair-Id=APKAIE5G5CRDK6RD3PGA)
Haemoglobin concentration (g/dl) in the outflowing flush solution. There was a significant difference between RF and AF at time point 0 [(*) p = 0.04]. AF: anterograde flush; RF: retrograde flush.
3.5 IL-1ß and TNF-α
Concentrations of IL-1ß and TNF-α in the right lung after explantation and in the left lung after reperfusion are shown in Table 3 . There were no significant differences for both cytokines amongst the three groups.
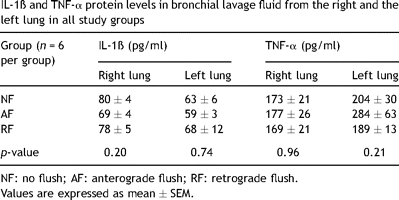
IL-1ß and TNF-α protein levels in bronchial lavage fluid from the right and the left lung in all study groups
3.6 Histology
Histological examination of biopsies from the right, non-perfused lung showed capillaries occluded with microthrombi in 6/6 NF and in 6/6 AF versus only 1/6 RF (Fig. 5A–C ). One biopsy from the left lung in NF was excluded from the analysis for technical reason. After reperfusion only a small amount of microthrombi were left (2/5 NF, 1/6 AF and 0/6 RF).

Histology of lung specimens obtained from the right lung (phosphotungistic acid–haematoxylin stain; original magnification ×200). Residual microthrombi (arrow) were observed more frequently in the capillaries after NF (A) and AF (B) compared to RF (C).
4 Discussion
In this study, we compared graft performance in NHBD lungs after retrograde flush versus anterograde flush and no flush. We found that pulmonary vascular resistance was significantly lower after retrograde flush. Haemoglobin concentration in the outflowing flush solution was also significantly higher in RF versus AF at the start of the flush. PTAH staining of lung biopsies revealed less microthrombi in the non-perfused lung after retrograde flush. We assume that these findings were directly related to each other and that a better washout of microthrombi resulted in a lower PVR upon reperfusion.
Lung transplantation, nowadays, is limited by a scarcity of suitable organ donors. Clinical series have recently suggested that the use of pulmonary grafts from NHBDs may be a valuable option to alleviate this shortage. In order to safely use the lung from a NHBD, the length of warm ischaemic period can be reduced by insertion of thoracic drains for topical cooling [5,8]. Microthrombi formed in NHBD lungs during the pre-flush ischaemic interval are considered to represent a risk for graft dysfunction following transplantation [2]. Administration of agents like heparin or fibrinolytics may help to better preserve organ function [2,12]. Flushing the lungs during procurement may be a strategy to remove residual microthrombi, thereby improving graft performance following transplantation. All these protective strategies in the NHBD, however, may raise ethical questions [10]. Furthermore, permission to intervene after death depends on the legislation for organ donation and harvesting (presumed consent vs explicit consent) and differs from country to country.
Several groups have previously investigated the preferred route of pulmonary flush after warm ischaemia in the NHBD [3,11–15]. Most of these reports, however, failed to compare retrograde pulmonary flush, anterograde pulmonary flush or no flush in non-heparinized animals in one experimental set-up. We recently already demonstrated that retrograde flush of the pulmonary graft immediately after 1 h of warm ischaemia resulted in a lower PVR during reperfusion [9].
The objective of the present study was to create a situation resembling an NHBD category I according to the Maastricht classification [16]. Therefore, the animals were sacrificed by ventricular fibrillation. There was no attempt for cardio-pulmonary resuscitation and animals were not heparinized. After 1 h of warm ischaemia preservation was started by means of topical cooling. The lungs were then harvested after a retrograde, anterograde or no flush and evaluated in the ex vivo model. During the flush ventilation was restarted in a controlled way with a low tidal volume and a low frequency to avoid cold lung injury related to mechanical stress. To our knowledge this study is the first to investigate the best route of pulmonary flush after additional topical cooling in a large animal model.
In the early days of lung transplantation prior to cold flush preservation, the lungs were cooled and stored by immersion in 4 °C Collins solution as initiated by the Toronto Lung Transplant Group [17].
The effect of topical cooling on NHBD graft performance has also been investigated in several animal studies. Steen and colleagues demonstrated in a pig transplant model that topical cooling is an excellent method to preserve the graft inside the cadaver [18]. Animals were heparinized and no pulmonary flush was performed. In a previous study, our group compared post-mortem ventilation with topical cooling as a method to protect the graft during the warm ischaemic period. We found that topical cooling was superior to preserve the graft inside the warm cadaver [5] and that this can safely be extended up to 6 h [19]. Kutschka and colleagues reported a study comparing anterograde flush in the heart-beating donor (HBD) versus topical cooling for 30 min in NHBD in a unilateral porcine lung transplant model [20]. In both groups, the lungs were stored for 24 h at 8 °C in a low potassium dextran solution. Surprisingly, haemodynamic function and animal survival time were superior in the topical cooling group compared to the flush group.
Only one recent study focused on the use of pulmonary flush following topical cooling. Snell and colleagues reported that anterograde flush of lungs in a dog model after 120 min of topical cooling preceded by 120 min of warm ischaemia is feasible [21]. Outcome was comparable with other strategies of NHBD lung preservation and evaluation. These authors also stated that a blood flush evaluation preceding flush cooling may have a role in the assessment of the allograft from the NHBD. A limitation of that study, however, was the limited number of experiments performed.
In all these previous studies, animals were heparinized. We did not administer heparine prior or after cessation of circulation since our first interest was to investigate solely the benefit of pulmonary flush.
Erasmus and colleagues compared AF followed by RF after a short warm ischaemic period versus topical cooling after 1 h of warm ischaemia in a pig model and concluded that lung function was impaired after topical cooling [22]. This was characterized by a large increase in the alveolar–arterial oxygen gradient, lung oedema and an increased maximum ventilation pressure. In contrast to the previously discussed studies, cardiac death was induced by ventilator switch off instead of cardiac fibrillation. These authors hypothesized that the hypertensive period preceding cardiac arrest might have caused endothelial damage and a release of pro-inflammatory cytokines. This, in combination with 1 h of warm ischaemia, might have been the cause of impaired lung function in the topical cooling group.
In this study all our animals were sacrificed by ventricular fibrillation since our first interest was to evaluate the effect of pulmonary flush after additional topical cooling preceded by 1 h of warm ischaemia. We acknowledge that our animals were only exposed to a short agonal phase that may explain the better outcome in our study.
The group from Varela in Madrid reported the first large series of lung transplantation from out-of-hospital NHBD [8,23]. After a warm ischaemic interval of maximum 120 min, donors are heparinized and lungs are preserved by means of topical cooling via chest drains. During harvesting, an anterograde flush with Perfadex® through the pulmonary artery is performed followed by a flush with blood for graft evaluation. The procedure is completed with a retrograde flush for further preservation. Lung transplantation was performed successfully in 16 patients and all were oxygen independent at discharge [23]. This group provided clinical evidence that pulmonary flush after topical cooling is feasible and results in good outcome.
Assessment of the graft was performed using our well-established model of isolated ex vivo reperfusion. In this model, reperfusion is performed in a controlled setting with a maximum inflow pressure of 15 mmHg. The maximum flow through the lung is therefore determined by a decrease in PVR. We noticed a significantly higher flow in RF compared to AF and NF, resulting from lower pulmonary vascular resistance. Oxygenation capacity tended to be lower in RF at the start of the assessment. The reaction time of oxygen to bind to haemoglobin and the time required for oxygen to diffuse through the alveolo-capillary membrane are considered to be important in blood oxygenation. Other investigators have demonstrated that pulmonary capillary transit time is decreased during a state of increased cardiac output reflected by a deficit in oxygen transport [24]. Wedging of microthrombi in the capillary vessels may well explain the lower flow in AF and NF and subsequent somewhat better oxygenation. However, it is likely that after transplantation the high PVR in AF and NF will persist resulting in pulmonary hypertension, hydrostatic oedema, impaired oxygenation and finally graft failure. These data are consistent with the findings at histological examination of lung biopsies showing more microthrombi in the right lung in NF and AF compared to RF. This may also explain the longer time that was needed to warm up the lung in NF (p ≪ 0.05) and AF (NS) compared to RF (Table 2). These findings need to be further confirmed in a transplant model. We speculate that the difference in graft performance between groups would have become more evident in a transplant model compared to our pressure-controlled reperfusion model.
The role of IL-1ß and TNF-α in ischaemia–reperfusion injury was elucidated in previous studies [25–27]. NHBD graft function deteriorates with increasing warm ischaemic intervals and this is reflected by an increase in IL-1ß protein levels in the bronchial lavage before [26] and after reperfusion [27]. In the present study, we could not observe any significant difference in IL-1ß and TNF-α protein levels between the three groups before (non-perfused right lung) and also not after reperfusion (left lung). We think this may be related to the short warm ischaemic period. The results in the present study confirm once more that a warm ischaemic period limited to 60 min followed by topical cooling is not detrimental for the graft and demonstrate that no additional inflammatory injury is provoked by performing a pulmonary flush under controlled ventilatory settings.
In summary, we demonstrated that retrograde flush following topical cooling resulted in a better washout of blood and microthrombi and subsequent reduced pulmonary vascular resistance in our isolated lung reperfusion model. Based on these findings we would recommend to flush the NHBD lung in a retrograde manner.
Presented at the joint 20th Annual Meeting of the European Association for Cardio-thoracic Surgery and the 14th Annual Meeting of the European Society of Thoracic Surgeons, Stockholm, Sweden, September 10–13, 2006.
Winner of the 2006 Alessandro Ricchi Transplant Service Foundation Award of the European Association for Cardio-thoracic Surgery.
Acknowledgements
This work was supported by a grant from the Katholieke Universiteit Leuven OT/33/55. Dirk Van Raemdonck is a senior research fellow of the Fund for Scientific Research – Flanders (FWO G3C04.99). We acknowledge the administrative and technical help of Mrs N. Jannis.
Appendix A
Conference discussion
Dr W. Weder (Zurich, Switzerland): You mentioned that there were significantly less microthrombi after retrograde flush, which is not a surprise. Do you think all the other parameters like decreased pulmonary artery resistance or improved organ function are consequence of this?
Dr Van De Wauwer: We believe that the decrease in pulmonary vascular resistance is related to less microthrombi. In our model, the pressure in the pulmonary artery is fixed to 15 mmHg to avoid injury provoked by a higher pulmonary artery pressure. So it's a good model of controlled perfusion and controlled ventilation. The only difference between the three study groups was the flush that was performed. And so our hypothesis is that the decrease in microthrombi after retrograde flush is the reason for the lower pulmonary vascular resistance after reperfusion.
Dr Weder: So if you would be able to fully heparinize them prior, then you might not see this difference anymore?
Dr Van De Wauwer: We just finished some additional groups where we compared heparinization during the warm ischaemia with retrograde flush and no flush. In the three groups the warm ischaemia was followed by topical cooling. After heparinization, the results were better than in the group where we did not perform a flush. But the results were not better compared to the group where we performed a retrograde flush. In this study, retrograde flush was still better than heparinization.
Dr R.D. Davis (Durham, North Carolina, USA): I have a couple of questions regarding the study. Clinically, we do an antegrade and a retrograde. Is there really any harm in doing that in your experimental setting? I mean, this gets into the aspects of you’re trying to achieve cooling and you’re trying to eliminate microthrombi, which are pretty common in donors. And clearly, a donor who is in a vegetive state before support is withdrawn, you’re going to be dealing with micro-thrombo-emboli regardless if you heparinize them or not.
And the second question is probably a question that's not addressable strictly by the parameters that you have measured. Coagulation activation in almost all acute lung injury models will have a profound effect on a variety of cytokines. It actually has more of a demonstrable effect when you look at IL-6 and some of the other downstream endothelial activation markers than anything that you can measure on the coagulation side. Have you tried to assess that in your model? I would guess that you’ll actually get a better feedback in terms of a positive if you can use your retrograde as compared to antegrade and look at inflammatory markers as compared to just using physiologic measurements, which are pretty equivalent as you’ve already demonstrated.
Dr Van De Wauwer: In answering your first question, the antegrade flush was more harmful in this situation because you’re flushing a cold lung. It's important at that moment to control the pressure that you use during the flush. We try to keep the pressure during flush at 15 mmHg, in that way retrograde flush was better than antegrade flush.
Also, those are cold lungs and the lungs are not fully ventilated. What we saw is when we performed an antegrade flush at a higher pressure, there was a dilatation of the pulmonary artery with more damage to the lung than when we performed an antegrade flush with a lower pressure in the pulmonary artery or when we performed a retrograde flush.
In answering your second question, if I understand the question correctly, it was about the inflammatory marker parameters in those experiments. We evaluated TNF-alfa and interleukin-1ß, and there was no significant difference amongst the three groups.
Dr Davis: Again, is there a reason you can’t do both? Or is it deleterious if you combine antegrade and then follow with the retrograde? I’m just asking. Because in the U.S., when we do non-heart-beating donors, clinically, we tend to do both. And I’m just curious if there was something experimental that said that the combination was a bad combination.
Dr Van De Wauwer: You can do both when you perform a flush after 1 h of ischaemia, when the lungs are still warm. When the lungs are cold, there is more damage to the lung when you perform an antegrade flush because of the pressure of the flush that's higher than when you perform a retrograde flush. So when you want to combine both of them, you need to do almost the antegrade flush after the retrograde flush. But in my opinion and what I saw in the experiments, doing first an antegrade flush, and then followed by a retrograde flush, the damage to the lung is going to be higher than when only performing an antegrade flush or when only performing a retrograde flush.