-
PDF
- Split View
-
Views
-
Cite
Cite
Jeanette M. van den Goor, Rienk Nieuwland, Peter M. Rutten, Jan G. Tijssen, Chi Hau, Augueste Sturk, León Eijsman, Bas A. de Mol, Retransfusion of pericardial blood does not trigger systemic coagulation during cardiopulmonary bypass, European Journal of Cardio-Thoracic Surgery, Volume 31, Issue 6, June 2007, Pages 1029–1036, https://doi.org/10.1016/j.ejcts.2007.02.016
- Share Icon Share
Abstract
Objective: During cardiopulmonary bypass (CPB), systemic coagulation is believed to become activated by blood contact with the extracorporeal circuit and by retransfusion of pericardial blood. To which extent retransfusion activates systemic coagulation, however, is unknown. We investigated to which extent retransfusion of pericardial blood triggers systemic coagulation during CPB. Methods: Thirteen patients undergoing elective coronary artery bypass grafting surgery were included. Pericardial blood was retransfused into nine patients and retained in four patients. Systemic samples were collected before, during and after CPB, and pericardial samples before retransfusion. Levels of prothrombin fragment F1+2 (ELISA), microparticles (flow cytometry) and non-cell bound (soluble) tissue factor (sTF; ELISA) were determined. Results: Compared to systemic blood, pericardial blood contained elevated levels of F1+2, microparticles and sTF. During CPB, systemic levels of F1+2 increased from 0.28 (0.25–0.37; median, interquartile range) to 1.10 (0.49–1.55) nmol/l (p = 0.001). This observed increase was similar to the estimated (calculated) increase (p = 0.424), and differed significantly between retransfused and non-retransfused patients (1.12 nmol/l vs 0.02 nmol/l, p = 0.001). Also, the observed systemic increases of platelet- and erythrocyte-derived microparticles and sTF were in line with predicted increases (p = 0.868, p = 0.778 and p = 0.205, respectively). Before neutralization of heparin, microparticles and other coagulant phospholipids decreased from 464 μg/ml (287–701) to 163 μg/ml (121–389) in retransfused patients (p = 0.001), indicating rapid clearance after retransfusion. Conclusion: Retransfusion of pericardial blood does not activate systemic coagulation under heparinization. The observed increases in systemic levels of F1+2, microparticles and sTF during CPB are explained by dilution of retransfused pericardial blood.
1 Introduction
Blood becomes activated in patients undergoing cardiac surgery assisted by cardiopulmonary bypass (CPB). Initially, this activation was predominantly attributed to contact of blood with the body foreign surface of the extracorporeal bypass circuit of the heart lung machine [1]. More recently, smaller extracorporeal circuits and improved surface coatings have been developed with improved biocompatibility [2–4]. Despite these improvements, however, blood is still activated during the CPB. For instance, the levels of prothrombin fragment F1+2 (F1+2) and thrombin–antithrombin complexes, which both reflect coagulation activation, increase in systemic blood during bypass [3–12]. These increases occur despite extensive heparinization, suggesting that systemic coagulation activation is incompletely blocked.
It is well established that pericardial blood is highly activated, including coagulation and fibrinolysis [3,4,8–13]. In the literature, at least eight independent studies concluded that retransfusion of pericardial (wound) blood contributes to systemic coagulation activation [3,4,7–12]. Pericardial blood is retransfused to reduce the use of blood products, particularly in patients undergoing complex and prolonged heart surgery, whose volume of pericardial blood is too large to be discarded or to be processed by a cell saver [7,13].
At present, pericardial blood is generally believed to be the main initiator of systemic coagulation activation during bypass. Despite this paradigm, however, direct evidence that systemic coagulation activation is caused by retransfusion of pericardial blood is lacking. Therefore, the aim of the present study was to investigate whether or not retransfusion of pericardial blood triggers systemic coagulation activation.
2 Methods
2.1 Patients
Prospectively, 13 patients who underwent elective coronary artery bypass grafting assisted by CPB were included after signed informed consent had been obtained. Inclusion criteria were elective coronary artery bypass surgery, body surface area > 1.66 m2 and preoperative hemoglobin levels > 7.5 mmol/l. Exclusion criteria were combined valve surgery or aneurysmectomy, redo operations, insulin-dependent diabetes mellitus, renal or hepatic dysfunction, preoperative coagulopathics, preoperative intra-aortic balloon pumping and protocol violation (complications). This study was approved by the Medical Ethics Committee of the Academic Medical Center of Amsterdam. The first eight patients were randomized. The last five patients all received pericardial blood.
2.2 Cardiopulmonary bypass
All extracorporeal bypass circuits contained a flat sheet membrane oxygenator (Cobe Duo, Cobe Laboratories, Inc., Arvada, CO), a venous hard shell reservoir with an integrated cardiotomy reservoir, an additional reservoir to collect the pericardial suction blood, an arterial line filter, polyvinyl chloride tubing (Cobe Laboratories) and a roller pump as arterial blood pump (Terumo/Sarns, Ann Arbor, MI). The extracorporeal system was primed with 500 ml lactated Ringer’s solution, 1 l Haemaccell (Behring, Malburg, Germany), 100 ml mannitol 20% (w/v), 50 ml sodium bicarbonate 8.4% (w/v) and 200 ml aprotinin (2 × 106 KIU Trasylol; Bayer, Leverkusen, Germany). Magnesium sulphate (1 g/10 kg) and 10,000 IU bovine heparin (Leo Pharmaceutical Products, Weesp, The Netherlands) were added to the priming solution.
All patients received 300 IU/kg heparin (Leo Pharmaceutical Products, Weesp, The Netherlands) before cannulation of the aorta. Systemic heparinization was monitored (ACT-Junior, ITC, Edison, NY) at fixed time intervals before onset and during CPB, additional heparin was administered when required. CPB was performed with moderate hypothermia (30–34 °C) and a non-pulsatile flow rate of 2.4 l m−2 min−1, maintaining a mean arterial pressure of 50–80 mmHg. Myocardial protection was achieved using cold (4–8 °C) crystalloid cardioplegia solution (St. Thomas). After weaning from CPB and decannulation, heparin was neutralized with protamine sulphate at a 1:1 ratio.
In the retransfusion group, the contents of the additional reservoir with pericardial suction blood were retransfused via the cardiotomy reservoir (after removing the aortic cross-clamp, before the end of the CPB). Two of these patients received homologous blood products (packed red cells) during CPB. In the retainment group, the contents of the additional reservoir with pericardial blood was not retransfused but discarded after CPB.
2.3 Collection of blood samples
Arterial blood samples were obtained before induction of anesthesia (1), 15 min after start of CPB (2), at termination of CPB (3), 30 min after protamine sulphate administration (4) and at the first postoperative day (5). Between release of the aortic cross-clamp and the end of bypass, pericardial blood samples were collected from the extra reservoir used for the collection of pericardial suction blood. For analysis of the plasma samples, concentrations of F1+2, soluble tissue factor (sTF) and microparticle (MP) numbers were corrected for plasma dilution by hematocrit values (1-hematocrit).
2.4 Cell count
Blood samples for hemoglobin, hematocrit, white blood cell and platelet counts were collected in 5 ml glass vacutainer tubes containing EDTA (Becton Dickinson (BD), San Jose, CA), and analyzed on a Celldyn 4000 (Abbot, Mijdrecht, The Netherlands).
Blood samples (4.5 ml) for F1+2, sTF and MP numbers were collected into 0.5 ml 3.2% trisodium citrate (BD). Cells were removed by centrifugation (20 min at 1550 × g and room temperature) and plasma aliquots (250 μl) were snap frozen in liquid nitrogen and stored at −80 °C until use.
2.5 F1+2 and sTF
Plasma concentrations of F1+2 (Enzygnost F1+2; Dade Behring, Marburg GmbH, Germany) and sTF (Imubind Tissue Factor ELISA; American Diagnostics, Inc., Stamford, CT) were determined by ELISA, according to manufacturer’s instructions.
2.6 Platelet-derived and erythrocyte-derived microparticles (PMP and EryMP)
After thawing plasma aliquots on melting ice, plasma was centrifuged for 30 min (17,570 × g, 20 °C) to pellet the MP as described previously [14–17]. After removal of 225 μl of (MP-free) plasma, the 25 μl MP-enriched plasma was diluted with 225 μl of phosphate-buffered saline (PBS; 154 mmol/l NaCl, 1.4 mmol/l phosphate, pH 7.4), containing 10.9 mmol/l trisodium citrate. MP were resuspended and centrifuged (30 min at 17,570 × g and 20 °C). Again, 225 μl of the supernatant was removed and MP were resuspended in the remaining 25 μl and diluted fourfold with PBS/citrate buffer, of which 5 μl was used per flow cytometric determination.
2.7 Flow cytometric analysis
MP samples were analyzed in a FACSCalibur flow cytometer with CellQuest software (BD). To distinguish PMP and EryMP from events due to noise, they were identified on forward scatter (FSC), side scatter (SSC) and binding of APC-labeled annexin V (Caltag Laboratories; Burlinghame, CA) and either FITC-labeled anti-CD61 (glycoprotein IIIA; clone Y2/51, IgG1 from Dako A/S (Glostrup, Denmark)) or CD235a (FITC-labeled anti-glycophorine A (Dako; Glostrup, Denmark)). To identify annexin V-positive MP, a fluorescence threshold was placed in a MP sample prepared without addition of calcium to correct for autofluorescence. To identify CD61- or CD235a-positive events, MP were incubated with a similar concentration of isotype matched control antibody (FITC-labeled IgG1; BD) to set the fluorescence threshold. MP (5 μl) were diluted in 35 μl PBS containing 2.5 mmol/l CaCl2 (pH 7.4) plus annexin V (5 μl) and either CD235a (5 μl) or anti-CD61 (5 μl) or IgG1-control antibody. The mixtures were incubated in the dark (15 min, room temperature). Subsequently, 900 μl PBS/calcium buffer was added before flow cytometry, and all samples were analyzed for 1 min. To estimate the number of PMP and EryMP per ml plasma, the number of events (N) found in the upper right (marker- and annexin V-positive) quadrant of the flow cytometry analysis (FL1 vs FL2) was used in the formula: Numbers/ml = N × [(955/5)/(59 × 2.5)] × 1000.
2.8 XACT procoagulant phospholipid (PPL) test
The XACT–PPL test was performed as described by Exner et al. [18]. using prototype kits from Diagnostica Stago R&D. Time for clotting was determined on a ACL Top (Instrumentation Laboratory Company; Lexington, MA).
2.9 Calculation of the estimated F1+2, sTF and MP numbers
We calculated the estimated total increase in the amount of F1+2 (nmol) in individual patients in systemic plasma. This total increase was estimated as follows: ([F1+2]sample time 3 − [F1+2]sample time 2) × (hematocritsample time 1/hematocritsample time 3) × systemic plasma volume. The systemic plasma volume was determined by calculating the circulating blood volume of individual patients by the linear regression equations for males (0.700H3 (m) + 0.042W (kg) − 0.691) and females (0.075H3 (m) + 0.038W (kg) + 2.002), in which H = height (m) and W = weight (kg) × (1 − [hematocritsample time 2 + hematocritsample time 3/2]) [19,20]. Subsequently, the total amount of F1+2 (nmol) present in the pericardial plasma of individual patients was calculated: ([F1+2] (nmol/l) × volumepericardial blood (l) × (1 − hematocritpericardial blood).
2.10 Statistics
Data were analyzed using SPSS, release 11.0 (Chicago, IL). Demographic and CPB data are reported as numbers or as medians with interquartile ranges. Outcome data (F1+2, sTF and MP numbers) were corrected for plasma concentrations (1-hematocrit) and are presented as medians with interquartile ranges. Statistical analyses were performed on the change (Δ) of that variable during CPB, i.e. between termination of CPB (sample time 3) minus 15 min after start of CPB (sample time 2) per individual patient. Differences are considered to be statistically significant when p ≪ 0.05 (two-sided). Data between retransfused and non-retransfused patients were compared by the independent-samples t-test. Within the two patient groups, data from different sample times were compared with the paired-samples t-test. For all patients, the calculated amounts of F1+2 (nmol), PMP (107), EryMP (107) and sTF (ng) in pericardial blood and the total increase in these parameters in systemic blood during bypass were compared with the paired-samples t-test. Differences between the patient groups with regard to the total increase in systemic blood – the total amounts present in pericardial blood of individual patients were determined by the two-samples t-test. Linear regression analysis was used to measure the relationship between F1+2 and the volume of retransfused pericardial blood. The sample size was on the basis of preliminary observations, indicating that approximately eight patients would be sufficient to achieve statistically significant differences in coagulation activation.
3 Results
3.1 Systemic coagulation activation during clinical CPB
In Table 1 , the descriptive demographic and CPB data are summarized. No complications were observed in any of the included patients. On average, 500 ml pericardial blood (275–725 ml) was collected. There was no difference between the volume of pericardial blood between retransfused and non-retransfused patients (500 ml [238–725 ml] vs 475 ml [313–863 ml]; p = 0.704) (Table 2 ).
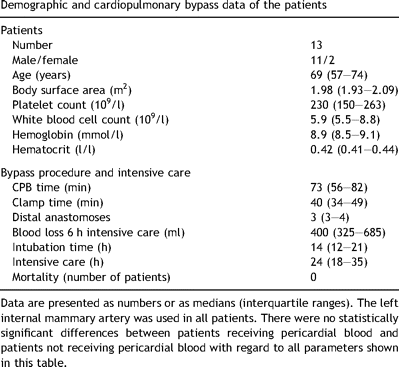
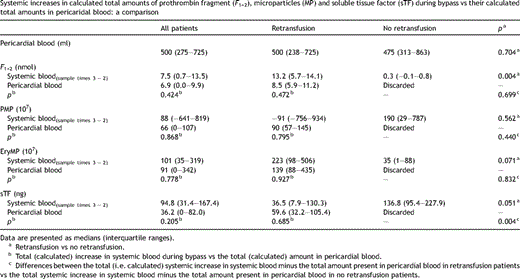
Systemic increases in calculated total amounts of prothrombin fragment (F1+2), microparticles (MP) and soluble tissue factor (sTF) during bypass vs their calculated total amounts in pericaridal blood: a comparison
Fig. 1A shows the concentrations of prothrombin fragment F1+2 in systemic blood samples collected before (1), during (2 and 3) and after CPB (4), and at the first day at the ICU (5). For comparison also the levels of F1+2 in pericardial blood samples are shown. Compared to systemic blood, coagulation is highly activated in pericardial blood. Since the scaling of the Y-axis of Fig. 1A is too large to visualize any changes in systemic levels of F1+2 during bypass, only the systemic concentrations of F1+2 are shown in Fig. 1B. During bypass, the systemic concentrations of F1+2 increased from 0.28 (0.25–0.37; median, interquartile range) to 1.10 (0.49–1.55) nmol/l (p = 0.001). These data seem to confirm several studies which indicated that retransfusion of pericardial blood triggers systemic coagulation. This conclusion, however, depends on the extent of increases in systemic coagulation activation products versus the quantity of pericardial coagulation activation products transfused into the patient. Since the observed increase in systemic F1+2 during bypass was comparable to the estimated (calculated) increase (p = 0.424), the observed increase is explained on basis of dilution of pericardial blood (Table 2). Thus, the observed increase in systemic levels of F1+2 during bypass in patients who receive pericardial blood can be explained on basis of retransfusion and dilution and not on basis of de novo synthesis.
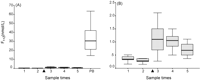
Coagulation activation during bypass. (A) Concentrations of prothrombin fragment F1+2 in systemic (sample times 1–5) and pericardial blood (PB) samples (n = 13). Systemic blood was collected before (sample time 1) and during (sample times 2 and 3) bypass, after protamine sulphate administration (sample time 4) and on the first postoperative day at the intensive care unit (sample time 5). The closed triangle indicates retransfusion of pericardial blood. (B) Similar to panel A, but with a different scaling of the Y-axis to visualize the changes in systemic concentrations of F1+2 (n = 13). Data are shown as medians (interquartile ranges).
In Fig. 2A and B , the concentrations of F1+2 are shown for both the retransfused (Fig. 2A; n = 9) and non-retransfused patients (Fig. 2B; n = 4). In the patients who received pericardial blood during bypass (Fig. 2A), the concentration of F1+2 increased by 1.12 nmol/l (p ≪ 0.001). In contrast, patients who did not receive pericardial blood (Fig. 2B) showed no significant change in levels of F1+2 (0.02 nmol/l; p = 0.250). The observed changes in systemic F1+2 differed between the retransfused and non-retransfused patients (p = 0.001). Thus, only in the retransfused patients the systemic concentrations of F1+2 increased significantly during bypass. The observed increase in systemic concentrations of F1+2 correlated with the volume of retransfused (pericardial) blood (r = 0.798, p = 0.001; Fig. 2C), confirming the relationship between the amount of retransfused F1+2, which is present in the pericardial blood, and the increase in systemic levels of F1+2.
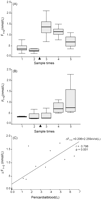
Effect of retransfusion of pericardial blood on systemic concentrations of prothrombin fragment F1+2. (A) Concentrations of F1+2 in systemic blood samples of nine patients receiving pericardial blood (between sample times 2 and 3, indicated by the black triangle). (B) Concentrations of F1+2 in systemic blood of four patients not receiving pericardial blood (between sample times 2 and 3, indicated by the black triangle). (C) Correlation between the volume of retransfused pericardial blood and the observed change in systemic concentrations of F1+2 during CPB (n = 13). Data are shown as medians (interquartile ranges).
3.2 MP and sTF
We also determined the numbers of both PMP (Fig. 3A ) and EryMP (Fig. 3B) and the level of sTF (Fig. 3C) in systemic and pericardial blood. All three parameters are elevated in pericardial blood compared to systemic blood. The numbers of MP (p = 0.645 and p = 0.005 for PMP and EryMP, respectively) and sTF (p = 0.006) increased or tended to increase during CPB. As shown in Table 2, also the observed changes in PMP, EryMP and sTF were similar to the estimated increases (p = 0.868, p = 0.778 and p = 0.205, respectively). Thus, like the systemic increases in F1+2 during bypass, also the observed increases in PMP, EryMP and sTF are similar to the estimated increases and therefore are due to dilution of the pericardial blood (Table 2).
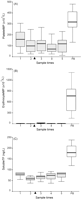
Microparticles and soluble tissue factor during bypass. (A) Numbers of platelet-derived microparticles, (B) erythrocyte-derived microparticles and (C) concentrations of soluble tissue factor in systemic (sample times 1–5) and in pericardial blood (PB) samples (n = 13). Data are shown as medians (interquartile ranges).
The observed changes in PMP and EryMP during bypass showed no significant differences between the retransfused and non-retransfused patients (p = 0.707 and p = 0.060, respectively). When we compared the estimated total increase in systemic blood minus the total amount present in pericardial blood of individual patients for both the retransfusion and no retransfusion group, no differences were found for both PMP and EryMP (p = 0.440 and p = 0.832). For sTF, however, we found a small difference between 15 min CPB and end CPB when comparing both patient groups (p = 0.039). We also found a difference between both groups when comparing the estimated total increase in systemic blood minus the total amount present in pericardial blood of individual patients (p = 0.004). However, whether or not these differences are of clinical relevance is doubtful, since these changes range only between 3 and 14% of (systemic) baseline values.
3.3 Procoagulant phospholipids
Despite the fact that pericardial blood contains high numbers of procoagulant MP (Fig. 3A and B), no de novo synthesis of F1+2 was observed in systemic blood after retransfusion of pericardial blood. Therefore, we hypothesized that MP and other procoagulant phospholipids may be cleared (after retransfusion) before heparin becomes neutralized. Fig. 4 shows minor and insignificant increases during bypass in all patients (Fig. 4A), in the patients who received retransfused blood (Fig. 4B) and in the patients who received no retransfused blood (Fig. 4C). No differences were present between the retransfused and non-retransfused patients at sample time 3; end CPB (p = 0.279). In the retransfused patients (Fig. 4B), however, a decrease was observed in PPL before and after neutralization of heparin (p = 0.001), whereas in the non-retransfused patients (Fig. 4C) no decrease was observed (p = 0.421). PPL values in both patient groups were similar at sample time 4; 30 min after administration of protamine sulphate (p = 0.996). Thus, MP and other procoagulant phospholipids are efficiently cleared from the systemic circulation before neutralization of heparin.

Procoagulant phospholipid (PPL). (A) Concentrations of PPL in systemic blood samples (sample times 1–5; n = 13). (B) Concentrations of PPL in systemic blood samples of nine patients receiving pericardial blood (between sample times 2 and 3, indicated by the black triangle). (C) Concentrations of PPL in systemic blood of four patients not receiving pericardial blood (between sample times 2 and 3, indicated by the black triangle). Data are shown as medians (interquartile ranges).
4 Discussion
The present study shows that compared to systemic blood, coagulation is highly activated in the pericardial (wound) blood. This is in line with previous studies by us and others [3,4,8–13]. In addition, we showed that systemic concentrations of F1+2 only increase during bypass in patients who receive retransfused pericardial blood. This observed increase, however, is identical to the estimated (i.e. calculated) increase of F1+2. Thus, the observed increase in the systemic concentration of F1+2 is due to dilution of the pericardial blood. As a consequence, retransfusion of pericardial blood does not trigger systemic coagulation activation. We are well aware of the limitations of our present study due to the small number of patients. Still, our present data show that dilution of pericardial blood explains the observed systemic increases of F1+2 during bypass.
In patients who did not receive retransfused pericardial blood, the systemic concentrations of F1+2 did not increase. This implicates that the contact between blood and the extracorporeal circuit does not or hardly activates coagulation during bypass in these uncomplicated patients. From the present data, however, it cannot be excluded that administration of protamine sulphate affects coagulation. Likewise, it cannot be excluded that the extracorporeal circuit activates coagulation in patients who are undergoing prolonged and more complex surgery.
In previous studies, summarized in Table 3 , retransfusion of pericardial blood was considered to trigger additional and renewed systemic coagulation [3,4,7–12]. However, in none of these studies the estimated increases in systemic levels of F1+2 or thrombin–antithrombin complexes, based on the retransfusion (dilution) of pericardial blood, were calculated. In contrast, our present findings indicate that systemic coagulation is not activated upon retransfusion of pericardial blood. We also observed no differences between retransfused and non-retransfused patients with regard to systemic levels of F1+2 (p = 0.226), PMP (p = 0.274), EryMP (0.626) and sTF (p = 0.892) on the first postoperative day. In line with our present findings is a large observational study on 5000 coronary artery bypass grafting patients, from which it was concluded that retransfusion of pericardial blood and even of mediastinal blood ‘did not carry any clinical disadvantages or harmful effects’ [21]. The clinical relevance of our present findings is that with regard to (systemic) coagulation, retransfusion of autologous pericardial blood is not harmful to the patient. Since we only studied coagulation activation, we cannot exclude that other processes, including the inflammatory response, may be affected by retransfusion of pericardial blood [22,23].
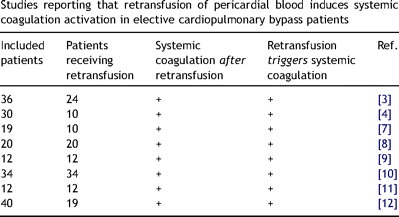
Studies reporting that retransfusion of pericardial blood induces systemic coagulation activation in elective cardiopulmonary bypass patients
Compared to systemic blood, pericardial blood is known to contain increased numbers of cell-derived MP [15]. These MP originate from various cell types and initiate coagulation via a tissue factor and factor VII(a)-dependent mechanism in vitro [2,15,24] and thrombus formation in vivo [25]. One could have expected that retransfusion of pericardial blood, i.e. blood which contains high numbers of procoagulant MP, triggers systemic coagulation. Our present data, however, demonstrate that retransfusion of pericardial blood does not trigger systemic coagulation activation. Most likely, no systemic coagulation activation is observed because MP are cleared from the circulation during the time interval (at least 15 min) before heparin is neutralized by protamine sulphate. Data from the PPL assay confirm this hypothesis. Thus, efficient clearance of MP and other procoagulant phospholipids is likely to explain the absence of de novo synthesis of systemic F1+2. From our previous studies we know that sTF in pericardial blood is associated for about 45–75% with cell-derived MP [24]. This TF is highly coagulant, but due to the clearance of MP, is also MP-associated (pericardial) TF may be removed from systemic blood and therefore is no longer expected to trigger (systemic) coagulation.
Taken together, our present data show that retransfusion of pericardial blood does not trigger systemic coagulation during uncomplicated elective CPB procedures.
Acknowledgment
The prototype kits for XACT tests were a kind gift of Dr B. Woodhams (Diagnostica Stago R&D; Asnieres, France).