-
PDF
- Split View
-
Views
-
Cite
Cite
Knut E. Kjørstad, Dag Ole Nordhaug, Christian Korvald, Stig Müller, Tor Steensrud, Truls Myrmel, Mechanical restitution curves — a possible load independent assessment of contractile function, European Journal of Cardio-Thoracic Surgery, Volume 31, Issue 4, April 2007, Pages 677–684, https://doi.org/10.1016/j.ejcts.2007.01.013
- Share Icon Share
Abstract
Objective: The time constant of mechanical restitution (T(MRC)), proposed to reflect changes in calcium release and uptake, has been shown to increase in left ventricular (LV) failure, and might have a potential as an index of contractile function. However, in vivo studies of the effect on T(MRC) of changing loading conditions in the normal and failing heart have not been reported. Consequently, in this study, we tested the hypothesis that the increase in T(MRC) in vivo is independent of preload and afterload. Methods: Left ventricular pressure–volume loops were assessed at baseline in eight open chest pigs using the combined pressure–volume conductance catheter technique during right atrial pacing at 120 b/min. Mechanical restitution curves (MRC) were constructed during four different loading conditions in all eight animals: uninfluenced load, reduced preload (balloon catheter in v. cava inferior), increased afterload (balloon catheter in descending aorta), and increased preload combined with reduced afterload (aortocaval shunting). Acute LV failure was then induced by microembolization through the left main coronary artery, and the experimental protocol was repeated. Contractile response was defined as the maximal first derivative of pressure (dP/dtmax), and T(MRC) was calculated using a least square approximation algorithm. Results: Hemodynamic data 30 min after microembolization showed decreased mean arterial pressure (98 ± 14–67 ± 10 mmHg, (mean ± SD) P ≪ 0.0001) and dP/dtmax (1482 ± 193–1001 ± 125 mmHg/s, P = 0.001). Stroke volume decreased from 30 ± 5 to 20 ± 5 ml (P ≪ 0.0001) compared to baseline, and preload recruitable stroke work decreased from 52 ± 7 to 31 ± 10 mmHg (P = 0.002). T(MRC) increased in all eight animals after induction of LV failure at all loading conditions. There was no difference between the different loading conditions at baseline, nor at LV heart failure, but T(MRC) increased significantly after the induction of heart failure (ANOVA, two ways). Conclusions: We have shown that the left ventricular T(MRC) increases after developed heart failure. The increase in T(MRC) was independent on loading conditions and thus have a potential for a contractility index.
1 Introduction
The interpretation of the force-interval relationship of cardiac muscle as a compartmental model of Ca2+ transport has gained increasing evidence during the last 25 years [1–6]. The essence of the model was formulated by Yue et al. [4] and proposes two functional calcium compartments, an uptake and a release compartment within the sarcoplasmatic reticulum. From these compartments, variable amounts of calcium are made available to the myofilaments as a function of beat-to-beat intervals. The plot of the contractile response (CR) of beats at different intervals can then be described by a monoexponential function, the mechanical restitution curve (MRC), where the slope is given by the time constant of mechanical restitution (T(MRC)) [3]. In an experimental setting, the MRC is reflecting the amount of calcium made available for release during the different test pulse intervals (TPI), and the myofilament response to this Ca2+. The myofilament response is in essence cardiac contractility.
The model has mainly been developed using empirical studies on isolated, isovolumically beating hearts where variations in preload as a function of TPI are eliminated. Under these experimental conditions, Burkhoff et al. [3] demonstrated that T(MRC) was independent of priming frequency and ventricular volume. However, in an in vivo mechanical restitution protocol, pre- and afterload vary depending on variations in filling periods: preload increases and afterload decreases with increasing TPI (time varying load). Hardman et al. [7] addressed this issue by comparing mechanical restitution curves from patients during atrial fibrillation with corresponding curves derived from isolated cardiac muscle. Their results support the concept that mechanical restitution is independent from time varying load, i.e. that variation in contractile force is not a function of filling, but a function of time and cardiac contractile function per se.
The MRC concept was further explored by Prabhu and Freeman [8] who showed that the time constant increased after tachycardia induced heart failure in dogs using single beat elastance as contractile response (CR). Neuman et al. [9] measured systolic wall thickening as CR, and also found T(MRC) to be increased after induction of heart failure, unaffected by heart rate and autonomic blockade. Finally, in a recent paper we have shown that T(MRC) is sensitive enough to detect reversibly impaired left ventricular (LV) contractile function or stunning [10].
These results make the T(MRC) potentially useful as an index of LV contractile status in a clinical context. The concept seems especially attractive as a monitoring adjunct in cardiac surgery where the patients are equipped with pacing electrodes, and where optimal frequency and impulse conduction are paramount to adequate hemodynamics. In these patients, MRCs can easily be created as part of optimizing their hemodynamic and clinical condition in a postoperative setting. However, the perioperative loading conditions in these patients vary considerably requiring the index to be pre- and afterload independent, i.e. independent of changing steady state loading conditions.
Consequently, to further assess the potential for mechanical restitution as a contractile index, we evaluated whether T(MRC) based on maximal first derivative of pressure (dP/dtmax) as CR is independent of changing loading conditions in vivo. Furthermore, we explored whether failing myocardial function can be discriminated from load effects in hearts with acute ischemic heart failure.
2 Materials and methods
The investigation conforms with the European Convention on Animal Care, and the experimental protocol was approved by the local steering committee of the Norwegian Experimental Animal Board. We used 10 Norwegian landrace pigs of either sex. Pig body weights were 33 ± 5 kg (range: 25–39 kg). They were habituated to the animal facilities over 5–7 days and fasted overnight with free access to water before experiments. Intramuscular ketamine (20 mg/kg) and atropine (1 mg) were used as premedication. Anesthesia was induced with boluses of pentobarbital-Na and fentanyl, and maintained by continuous intravenous infusions of pentobarbital-Na, fentanyl and midazolam as described in previous papers from our laboratory [11–13]. The pigs were tracheotomized and intubated, and ventilation was maintained with an air–oxygen mixture ( ) on a volume-controlled respirator (Servo 900, Elema-Schönander, Stockholm, Sweden). Tidal volume was adjusted according to arterial blood gas samples ( 4.0–5.0 kPa).
2.1 Surgical preparation and experimental setup
Mean arterial pressure (MAP) was measured in the thoracic descending aorta, with a catheter inserted from the right femoral artery. Central venous pressure (CVP) was recorded in the right atrium via a catheter from the right external jugular vein. Urine was drained via a cystotomy, and diuresis was kept over 3 ml/kg/h by infusion of NaCl 0.9%. After sternotomy, a transit-time flow probe (Medi-Stim ASA, Oslo, Norway) was placed on the left anterior descending coronary artery (LAD). Pacemaker electrodes were sutured to the right atrium. The different loading perturbations were accomplished by the use of the following equipment: (1) A balloon catheter was inserted through the right femoral vein and advanced into the inferior caval vein (2 cm below the right atrium). (2) Another balloon catheter was placed in the abdominal aorta through the left femoral artery, and (3) an aortocaval shunt with an in-line 8 mm transit-time flow probe (Medi-Stim ASA, Oslo, Norway) was advanced via a midaxillary incision and the left subclavian artery into the aorta, and the other end of the shunt was inserted through the right auricle. Air was evacuated through a 3-way valve. The shunt was then filled with saline and heparin, and clamped.
Left ventricular pressure–volume relations were assessed using the combined pressure–volume conductance catheter technique [14]. The catheter, a 6-Fr, 12-electrode, dual field conductance catheter with pigtail (Millar Instruments, Houston, TX, USA), was inserted via the left common carotid artery. Recorded volumes were adjusted for blood resistivity (ρ) using blood samples taken from the abdominal aorta via the right femoral artery catheter, and parallel conductance (Vp, non-LV cavity derived conductance) was assessed by the hypertonic saline technique (injection of 4 ml NaCl 10% in the pulmonary artery). The gain correction factor (α) [14] was calculated from stroke volume (SV) measured with a transit-time flow probe (Medi-Stim ASA, Oslo, Norway) on the trunk of the pulmonary artery. Beat-to-beat LV conductance and pressure signals were sampled and stored at 200 Hz (Leycom Sigma 5 conditioner and Conduct PC software, both from CD Leycom, Holland).
Heparin was administered twice intravenously, 2500 IU during instrumentation and 2500 IU before induction of acute heart failure (see below). Autonomic blockade was accomplished by giving Hexamethonium Chloride (Sigma Chemical Co., St. Louis, MO, USA), 30 mg/kg in NaCl 0.9% (50 ml) as an i.v. bolus before starting the experimental protocol. In order to reduce the risk of ventricular fibrillation during heart failure, 150 mg of amiodarone (Cordarone, Sanofi Synthelabo Inc., New York, NY, USA) in 100 ml Glucose 5% was given as an i.v. infusion over 20 min during instrumentation.
2.2 Induction of left ventricular failure
We have shown earlier that in pigs, the area supplied by the left coronary artery constitutes more than 80% of the left ventricle, including the septum [13]. Consequently, microembolization through the left coronary main stem results in a global LV failure, and acute heart failure was therefore induced as described previously [15]. Briefly, a 6 Fr. catheter was placed in the left coronary main stem via the right common carotid artery under fluoroscopic guidance, and 2.5–5.0 mg boluses of 50 μm polystyrene microspheres (NEM-500, NEN Life science Products, Boston, MA, USA) dissolved in 0.9% NaCl and 0.01% Tween 80 (Sigma Chemical Co., St. Louis, MO, USA) were injected every 5 min until a 25% decrease in MAP was obtained. An average of 25 ± 5 mg microspheres given over approximately 40 min was needed to achieve this level of LV failure. The same catheter was also used for pressure monitoring in the ascending aorta during increased afterload (inflated aortic balloon, see below).
2.3 Experimental protocol
In this study, each animal served as its own control, i.e. assessment and comparison of mechanical restitution (T(MRC)) was done before and after induction of LV heart failure. After completion of the surgical instrumentation, right atrial pacing was initiated at a cycle length of 500 ms in seven pigs with intrinsic frequencies ranging from 100 to 106 beats/min. Pig number 6 had an intrinsic frequency of 120 beats/min, and was therefore paced at a shorter cycle length (450 ms). The preparation was then allowed 20 min of stabilization before baseline registrations. These included assessment of two Vp files, and two transient vena cava occlusions files (VCO, caval balloon) for estimation of linear end systolic elastance (Ees) and preload recruitable stroke work (PRSW). Thereafter, the MRC protocol was conducted. The protocol was performed by successively decreasing the TPI from 500 ms by 30–10 ms steps (decreasing decrements with decreasing TPI) until measured R–R interval was more than 10 ms longer than the TPI (TPI0). The test pulse interval was then successively increased from 530 ms by 30 ms steps until an escape beat occurred. Each test pulse and the following extrasystole were preceded by at least 10 steady state beats, sampled during apnea and stored as separate 5 s long computer files comprising pressure, volume and ECG (Fig. 1 ). The aortocaval shunt was then opened, and after approximately 5 min of stabilization, the MRC protocol was repeated. The shunt was then closed and Heparin/NaCl was added to prevent clotting. The caval balloon was then inflated until a stable reduction in MAP of 25%, and the MRC protocol was run again. After deflating the caval balloon followed by approximately 5 min of stabilization, the intraaortic balloon was inflated. A stable 20% increase in MAP was established (measured via the catheter in the ascending aorta) and the MRC protocol was run again.
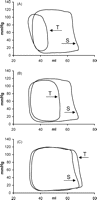
Pressure–volume loops following three different test pulse intervals, and their preceding steady state beats. Baseline data from one experiment: (A) test pulse interval of 360 ms; (B) 390 ms and (C) 590 ms. T, test beat; S, steady state beat.
After the induction of LV failure and 30 min of subsequent stabilization, the data settings were recalibrated with respect to ρ, Vp and α, and both the hemodynamic measurements and the MRC protocols were repeated. At the end of the experiment, the animal was killed by an overdose of pentobarbital-Na and an intracardial injection of KCl during aortic clamping.
2.4 Analyses and statistics

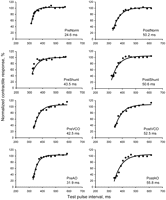
Mechanical restitution curves from one experiment (pig number 7) obtained during uninfluenced load (Norm), increased preload combined with reduced afterload (Shunt), reduced preload (VCO) and increased afterload (AO) at baseline (Pre) and after induction of heart failure (Post). The time constant of mechanical restitution is given in each panel.
3 Results
The experimental protocol included 10 pigs. Two of these were excluded because of progressive heart failure and irreversible cardiogenic shock after microembolization. The remaining eight pigs were used in the final analysis
3.1 Effect of LV heart failure on hemodynamic data
Data at baseline and 30 min after completion of the microembolization protocol are presented in Table 1 . At uninfluenced load (deflated balloon catheters and closed aortocaval shunt) MAP, left ventricular developed pressure (LVDP), SV, left anterior descending artery blood flow (LADBF), SW and dP/dtmax decreased significantly after induction of LV heart failure (P ≪ 0.0001 for all parameters). Afterload, i.e. systemic vascular resistance (SVR), remained unaltered. The contractility index PRSW was reduced (decreased slope of ) from 52.2 ± 7.4 to 30.5 ± 9.6 mmHg (P = 0.002) whereas the linear slope of Ees remained unaltered after microembolization (1.8 ± 0.4 and 1.7 ± 0.8 mmHg, respectively, P = 0.8).
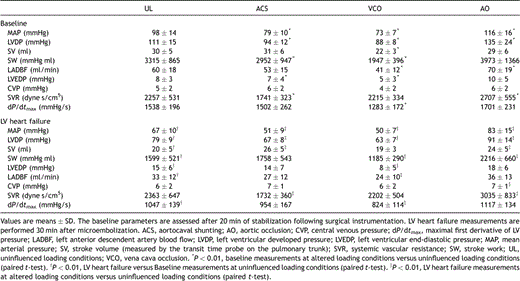
Because the changes in hemodynamic parameters were so extensive after microembolization, we limited the repeated measure statistics to one factor (load), and tested the data for variance only within groups (baseline and LV heart failure). The analysis revealed P values less than 0.001 for all parameters except for CVP at baseline (P = 0.01), and we therefore included contrasts (paired t-tests with uninfluenced load as reference) in the analysis (Table 1). With the aortocaval shunt open, 23 ± 8% of the cardiac output was shunted back to the right side of the heart at baseline and 17 ± 7% at LV heart failure. This resulted in a significant reduction in MAP, LVDP, SW and SVR both at baseline and after microembolization, and increased SV at LV failure. The main changes during reduced preload (inflated caval balloon) were reduced MAP, LVDP, SW, LADBF and dP/dtmax, whereas SVR remained unaltered. Increased afterload (inflated intraaortic balloon) resulted in increased MAP, LVDP and SVR compared to uninfluenced loading conditions both at baseline and LV failure. At LV failure, SV increased during aortic occlusion compared to uninfluenced load. Considering the substantially increased SVR and MAP, this last finding was quite unexpected because it suggests increased inotropy which is not supported by any significant changes in T(MRC) or dP/dtmax. However, it might be explained within the frames of Sarnoff’s [17] description of an intrinsic adaptation termed homeometric autoregulation, a phenomenon that has been extensively investigated by other groups since then [18].
3.2 Mechanical restitution
The range of data points (TPI) was limited by the time lag of the atrioventricular node (TPI0), and the escape beat which was given by the intrinsic heart rate. No data sets (TPI/CR) acquired within this range were excluded from the calculations of the MRCs. Mechanical restitution curves could be produced for all pigs at baseline and after microembolization at all loading conditions except for pig no. 6 at baseline and increased afterload (due to insufficient technical data quality), and the corresponding T(MRC) value was set to mean in the analysis of variance. We compared the T(MRC) data during the same loading conditions at baseline and LV failure (normal load at baseline vs normal load at LV failure, Shunt at baseline vs Shunt at LV failure, VCO at baseline vs VCO at LV failure, and AO at baseline vs AO at LV failure), and found that T(MRC) increased in all animals at all loading conditions except in pig number 3 at increased afterload (AO) (Fig. 3 ). The time constant of mechanical restitution also increased in all animals at all four loading conditions at LV failure compared with baseline, uninfluenced load. Mean values for T(MRC) at baseline and after induction of acute heart failure are given in Table 2 . There was no difference between the different loading conditions at baseline, nor after inducing LV failure, but T(MRC) was increased at heart failure compared to baseline (ANOVA, two ways).
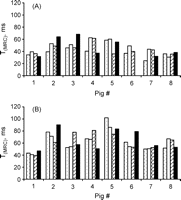
The time constant of mechanical restitution for all animals at all four loading conditions at baseline and after induction of acute heart failure. Panel A, baseline; panel B, LV heart failure. Columns from left to right for each pig: uninfluenced load, increased preload combined with reduced afterload (aortocaval shunting), reduced preload (v. cava balloon), increased afterload (intraaortic balloon).
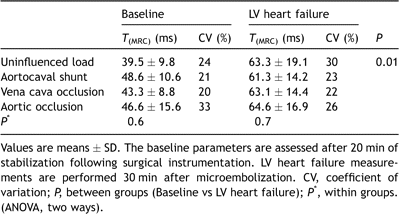
4 Discussion
The present study is the first to demonstrate that an increased time constant of mechanical restitution, as it is seen in acute left ventricular failure, is a load independent phenomenon in the in situ heart. Previous studies have indicated that a small intravenous infusion or leg raising maneuver will not influence mechanical restitution [2,19], but our study demonstrate that this aspect of the force-frequency relation is largely independent of extensively altered pre- and afterload.
The T(MRC) has previously been shown to satisfy essential criteria as an index of cardiac contractility: (1) it provides a measurement of the contractile status of the heart, i.e. it is capable of detecting a broad range of heart failure conditions [8–10], (2) it is independent of steady state heart rate (priming frequency) [3], (3) it reflects drug induced inotropic changes [20] and (4) the value of the index reflects the underlying mechanisms of myocardial contraction at a molecular level, i.e. the Ca2+-myofilament interaction [5,21]. In addition, the present paper demonstrates a crucial quality of T(MRC), namely its load independence or lack of influence from pre- and/or afterload both in the control state and after inducing contractile failure. The load independence makes the index unique among the several indexes that have been proposed to reflect the contractile state of the heart muscle in situ.
In addition to the above mentioned criteria for a useful index of LV function, in a clinical setting it is most desirable that the index is available without the need for invasive procedures such as acute preload reductions or LV catheterization. Neuman et al. [9] used ultrasonographically measured systolic wall thickening (ejecting measure) as CR, and found T(MRC) to be increased after induction of heart failure. However, an ejecting measure might be more sensitive to afterload variations than dP/dtmax[22] considering the tight relationship between stroke volume, peripheral pressure and systemic vascular resistance. Consequently, pre-ejection tissue Doppler measurements or strain rate could possibly add further merit to the usefulness of mechanical restitution in a clinical setting.
Previous authors exploring the force/frequency relation in ejecting hearts have described a late descending limb of the mechanical restitution curve at long test pulse intervals [2,3,19,23]. In the present study we used a pacing frequency only slightly faster than the intrinsic heart rate, and therefore we did not observe this phenomenon. Burkhoff et al. described the MRC as a monoexponential function based on a curve with a flat plateau, and inclusion of a descending part of the curve in the calculations should probably be avoided because it might change the plateau of the monoexponential function (CRmax) and subsequently the T(MRC).
The need for a load independent index of the LV contractile function is obvious. The efforts to establish one have been numerous, and the main focus has been on elastance and preload recruitable stroke work. In the present study, the general hemodynamic parameters (MAP, LVDP, dP/dtmax and SW), and the mechanical restitution curves, confirm the presence of acute LV heart failure, but linear Ees showed no statistically significant change. This observation adds to the growing number of papers where Ees is found to be inconsistent and in some cases unreliable as a contractility index [10,15,24,25]. On the other hand, PRSW decreased in the present study. This index has been shown to be more stable than Ees, but also less sensitive to alterations in the contractile state, probably because it incorporates both systolic and diastolic function [10,25].
4.1 Model considerations
The experiments in this study were conducted on autonomically blocked preparations. We regarded the exclusion of the neurohumoral interference via the baroreflexes as advantageous because it facilitated stable loading interventions during the MRC protocols, and probably shortened the stabilization periods needed between them. Also, undesired variations in intrinsic heart frequency were avoided in this analytical setting.
In vivo models aiming at exploring mechanical restitution can involve either atrial or ventricular pacing. However, when the experiments are conducted in pigs, atrial pacing must be used because ventricular pacing gives inadequate hemodynamics with a MAP less than 50 mmHg. Atrial pacing is also preferable because it gives a more physiological myocardial pulse propagation, and allows to some extent for comparisons of results from non-pacing protocols with or without atrial fibrillation. When using atrial pacing, TPI0 is longer than during ventricular pacing [6]. This is due to the time lag of the atrioventricular node, and explains why the normalized contractile response at TPI0 is about 30–40%. The lack of data points below 30% may be a source of random errors. However, we have shown in a previous paper [10] that such an error probably do not occur.
4.2 Limitations
The T(MRC) was increased at the same loading conditions after inducing LV heart failure in all animals but one (pig number 3 at increased afterload). Moreover, it also increased in all animals at all four loading conditions at LV failure compared with uninfluenced loading conditions at baseline. However, T(MRC) showed considerable variability between animals making determination of absolute contractile performance difficult. Thus, the index, in its present form, can probably not be used as an absolute contractility measure, but serial measurements over time can probably be applied perioperatively as a monitoring adjunct in cardiac surgery. Most patients are already equipped with temporary pacing electrodes which allow for serial bedside measurement of the T(MRC). However, to do routine measurements in a clinical setting will require a non-invasive measure of the contractile response. Future clinical applicability will therefore depend upon further testing with, preferably, echocardiography and tissue Doppler. A semi-automated computer algorithm will also be needed to make the method practically useful.
Finally, it should be emphasized that the T(MRC) is an index of global function, i.e. regional wall motion abnormalities can not be detected per se, and should therefore be evaluated by echocardiography, tissue Doppler or magnetic resonance imaging.
None of the proposed load-independent indexes of contractility have been able to stand the test of time through experiments in different models. The ability of these indexes, including Ees and PRSW, to reflect the quality and quantity of the ventricular muscle function in an intact heart is limited. The time constant of mechanical restitution is mimicking the core process of contractility, namely the exposure and removal of calcium from the contractile elements, and could therefore well be the most appropriate index to evaluate the contractile muscle function in an in vivo heart.
Acknowledgements
The authors wish to thank Yngvar Larsen and Dr Erik R. Traasdahl for crucial assistance in computer programming.
The present work was supported in part by grants from the following federal and regional governmental institutions: Norwegian Council on Cardiovascular Diseases, the Norwegian Research Council and Helse Nord RHF.