-
PDF
- Split View
-
Views
-
Cite
Cite
Mikko Mäyränpää, Jarmo Simpanen, Michael W. Hess, Kalervo Werkkala, Petri T. Kovanen, Arterial endothelial denudation by intraluminal use of papaverine–NaCl solution in coronary bypass surgery, European Journal of Cardio-Thoracic Surgery, Volume 25, Issue 4, April 2004, Pages 560–566, https://doi.org/10.1016/j.ejcts.2004.01.006
- Share Icon Share
Abstract
Objectives: Preservation of endothelial integrity during coronary artery bypass grafting is considered important for short- and long-term patency of the graft. In current literature there are controversial data about the safety of papaverine, a vasorelaxant widely used in coronary artery bypass grafting. Accordingly, we examined the immediate effects of papaverine on the endothelial morphology of arterial grafts and on viability of cultured human coronary artery endothelial cells. Methods: Totally 22 segments of the radial artery were collected from 11 patients undergoing coronary artery bypass grafting. The segments were cut from the distal redundant parts of the arteries, which were not required for bypass grafting. A small distal segment of the artery was excised and placed into autologous heparinized whole blood (control segment). The rest of the graft, still remaining proximally attached to the circulation, was treated intraluminally with papaverine–NaCl solution (pH 4.2) for 5 min, after which another small distal segment was excised for the study (papaverine-treated segment). Segments were then perfusion-fixed and the endothelial surface was examined by scanning electron microscopy. Endothelial denudation was assessed and its severity graded. Additionally, viability of cultured human coronary artery endothelial cells after treatment with papaverine was assessed with trypan blue staining. Results: Treatment with papaverine solution markedly increased the endothelial denudation (grades from 6.2±1.7 to 10.0±3.5; mean±SD; P=0.004). Papaverine-treatment increased trypan blue staining of cultured human coronary artery endothelial cells regardless of papaverine diluent (normal saline of pH 4.5 or 7.4 or blood). Conclusions: Treatment of arterial grafts with papaverine solutions apparently damages endothelial cells. Thus, the clinical practice of dilating arterial bypass grafts with papaverine should be reassessed, and alternative methods of vasodilatation should be considered.
1 Introduction
The radial artery is one of the arterial grafts most commonly used for myocardial revascularization, despite its high tendency to spasm [1]. An intact endothelium is essential for the normal regulation of arterial tone and actively prevents platelet aggregation and thrombus formation. In contrast, damage to the endothelium predisposes the vessels to vasospasm, formation of intraluminal thrombi, and ultimately to formation of a thickened intima as a result of smooth muscle cell proliferation. Thus, damage to the endothelium in coronary bypass grafts is considered potentially hazardous for both short- and long-term graft patency [2–4], and should be avoided.
In coronary bypass surgery, endothelial damage may be caused by mechanical stress [5], anoxic damage [6], and reperfusion damage [7], by unphysiological pH and osmolarity of the storage solution, or by direct enzymatic or toxic effects of substances used during the procedure [6,8]. It has also been proposed that the patency of bypass grafts may be compromised because of lack of functional vasa vasorum, which could lead to medial hypoxia and ultimately to accelerated graft atherosclerosis [4]. Heparinized autologous blood seems to be the most suitable solution for storing human arterial grafts and samples, as indicated by both morphological and functional studies [3,9].
Mechanical and hydrostatic dilatation of vessel grafts as means to overcome graft spasm have proved to be detrimental to the integrity of the vessel, and thus are not recommended [10]. Accordingly, numerous vasoactive substances have been used over the years to counteract the graft spasm, and their pharmacology and the vasoconstrictive mechanisms responsible for the spasm of CABG grafts have recently been reviewed [1]. Among the vasoactive compounds, one of the most commonly used is papaverine. It is a benzylisoquinoline alkaloid with non-selective smooth muscle relaxant properties [1,11].
Most experimental studies on graft vasospasm and its pharmacologic prevention have investigated either intraoperative flow or responses of vessel rings in an organ bath system [3,11–13]. Intraluminal or intrapedicular papaverine administration has been found to be more effective in preventing vasoconstriction than administration of papaverine to the external surface of the graft or submersion of the graft in papaverine solution [11,14]. Although the results of some earlier studies are inconsistent and difficult to interpret because of differences in methodology and reporting [11–14], they nevertheless suggest that papaverine causes little or no damage to the contractile mechanisms of smooth muscle cells of human arteries. However, papaverine may damage the endothelium and thus predispose the arterial grafts to postoperative vasospasm after cessation of its vasodilatative effect, which lasts up to 2 h [11]. Since knowledge regarding the effect of papaverine on the morphologic integrity of the arterial graft endothelium is very limited, we designed this study to examine the effects of intraluminally administered papaverine–NaCl solution on the morphology of the endothelium of human radial artery grafts which were used for CABG.
2 Materials and methods
2.1 Patients
Patients with histories of autoimmune disease, radiation therapy, insulin-dependent diabetes mellitus or cytostatic treatment were excluded from the study, since these conditions or treatments may cause severe damage to the endothelium.
All the patients were male, and the average age at the time of coronary artery bypass grafting was 64 years (range 53–74 years). The use of human samples was approved by the ethical review board of the hospital, and informed consent was obtained from all the patients.
2.2 Materials
Papaverine hydrochloride solution (0.1 M, 40 mg/ml) was purchased from Leiras Finland Ltd, Turku, Finland. Immediately before CABG, the solution was diluted in normal saline (0.15 M) to yield a final concentration of 4 mg/ml. The pH of the papaverine–NaCl solution used in this study was 4.2 prior to intraluminal injection. When injected intraluminally into the radial artery, the papaverine–NaCl solution became mixed with the residual volume of blood still present in the spastic artery. We measured the pH of this mixture of papaverine–NaCl solution and blood 5 min after the intraluminal injection. The measurements were performed with a Radiometer ABL 700 blood gas analyzer (Radiometer A/S, Brønshøj, Denmark) and confirmed with a WTW pH meter (Wissenschaftlich-Technische Werkstätten, Weilheim, Germany).
Tyrode's solution was prepared by adding 8 g NaCl, 0.2 g KCl, 0.2 g CaCl2·6H2O, 0.1 g MgCl2·6H2O, 1.0 g NaHCO3, 0.05 g NaH2PO4, and 1.0 g glucose to distilled water, the volume was adjusted to 1000 ml and the pH to 7.4 with 0.1 M HCl. The final concentrations of the Tyrode's solution were: sodium 135 mmol/l, potassium 2.4 mmol/l, calcium 0.80 mmol/l, and glucose 1.5 mmol/l. This solution was used as a diluent for the glutaraldehyde fixative. Glutaraldehyde was purchased from Merck, Darmstadt, Germany.
2.3 Surgical preparation of the samples
A total of 22 segments of radial artery were collected from 11 patients undergoing CABG. Segments were cut from the distal redundant parts of the harvested radial arteries, which were not required for bypass grafting. The radial arteries were harvested by the same surgeon with a standardized atraumatic technique previously described in detail [2]. An ultrasonic scalpel (Harmonic Scalpel, Ultracision Inc, Smithfield, RI) was used for side branch coagulation. All the patients had been heparinized (2–3 mg/kg heparin i.v.) prior to ligation and cutting of the distal end of the artery. The distal 1.5–2 cm of the artery was excised, cannulated from both ends with a Vessel cannula (Medtronic, Minneapolis, MN) and submerged in heparinized autologous blood (control segment). The rest of the now spastic radial artery was then filled with 5 ml of papaverine–NaCl solution carefully injected into the lumen with an olive-tipped Arteriotomy cannula (Medtronic). The harvested radial artery was left pulsating with a vascular clamp occluding the distal end. The intra-arterial papaverine–NaCl solution gradually became mixed with the patient's blood as the artery became dilated. After 5 min the papaverine–NaCl solution blood mixture was allowed to flow out, and then another 1.5–2 cm of the distal radial artery was excised (papaverine-treated segment) and handled in the same way as the control segment after excision. The incubation time of the segments in the heparinized autologous blood varied from 8 min to 1 h 47 min (mean 38 min), and was the same for the both control and the papaverine-treated segments of each patient. All incubations and fixations were performed at room temperature (+22 °C), which was also the temperature of all the solutions.
2.4 Fixation of the samples
The cannulated arterial segments (both control- and papaverine-treated segments) were fixed for 70 min with freshly prepared 1% glutaraldehyde in Tyrode's solution in a perfusion fixation system at constant pressure [15]. The pressure was maintained by placing the glutaraldehyde container 167 cm above the sample and clamping the exit cannula sufficiently to allow a slow drip. Intraluminal pressure downstream from the sample segment was monitored with an invasive arterial blood pressure monitor and adjusted to 118–120 mmHg. During perfusion, the arterial segments were placed in Ringer's lactate solution to prevent drying of the outer vessel surface. After perfusion fixation, the segments were immersed in 2.5% glutaraldehyde for up to 1 week and kept at +4 °C.
2.5 Further sample preparation for scanning electron microscopy
After the immersion fixation in glutaraldehyde, the perfusion cannulas were carefully removed from the ends of the segments and the central 5 mm of each segment was taken for further analysis. The segments were then opened longitudinally under a Leica MZ6 stereomicroscope (Leica Microsystems AG, Wetzlar, Germany) and cut into 1.5×5.0 mm2 pieces suitable for scanning electron microscopy. Special care was taken not to damage the endothelial lining during preparation. After preparation, sample pieces were dehydrated with a graded series of ethanol, and subjected to Critical Point Drying (with a CPD 030, from BAL-TEC, Balzers, Principality of Liechtenstein). Finally, the samples were mounted on aluminium stubs, using graphite glue (Electron Microscopy Sciences, Graphite conductive adhesive 154) and sputter-coated with a 25 nm thick layer of platinum (Agar Sputter Coater, from Agar Scientific Ltd, Stansted, UK).
2.6 Morphological analysis of endothelial damage
The entire endothelial surface of each sample piece was examined with a DSM 962 scanning electron microscope (from Carl Zeiss, Oberkochen, Germany) operating at an acceleration voltage of 10 kV. In an initial screening step, areas containing side branches or atherosclerotic plaques were discarded, since the morphology of the endothelium was different in these areas as also reported for human coronary and carotid arteries, and would therefore hamper reliable judgement of the papaverine effects [15].
SEM analysis was performed blinded regarding the treatment of individual sample pieces. The degree of endothelial damage was assessed using a modification of a previously described grading system [9]. For grading the endothelial damage, we used two criteria, (i) the area of endothelial denudation and (ii) the occurrence of pore-like structures within individual endothelial cells, referred to as ‘porosity’ in the text (note that the exact nature of these pore-like structures could not be assessed by means of conventional scanning electron microscopy). A scale of 1–5 was used for both categories and the overall points of all the pieces from one segment were counted. The reproducibility of the scoring system was evaluated by analyzing three segments twice in a blinded manner. The variation between the two scores obtained for these segments was 1%. Other criteria of endothelial damage used in literature, such as the frequency of fractures of the intima, and loss and separation of endothelial cells were not used, since these parameters were considered not to be independent of endothelial denudation. Total damage scores and P values were calculated with paired Student's t-test using a one-tailed distribution.
2.7 Cell culture experiments
To clarify the roles of low pH and direct effects of papaverine on endothelial cell damage additional experiments were performed using cultured human coronary artery endothelial cells (HCAEC, Clonetics, San Diego, CA). The cells were cultured according to the recommendation of supplier in endothelial cell basal medium-2 (Clonetics) supplemented with 5% fetal bovine serum and growth factors (Clonetics) at +37 °C under 5% CO2 until subconfluent. Then the cultures were treated with the test solutions for 5 min at +37 °C as indicated in Table 1 . Adjustment of the pH of the each solution was accomplished with 0.01 M NaOH or 0.01 HCl as required. Finally, the viability of the cells was assessed by staining the cultures with trypan blue, and for cell counting the cover plates were photographed with a digital camera (Spot RT, program version 3.03, Diagnostic Instruments Inc, Sterling Heights, MI) attached to a Nikon Eclipse E600 phase contrast microscope. On average of about 500 cells per culture dish were counted.
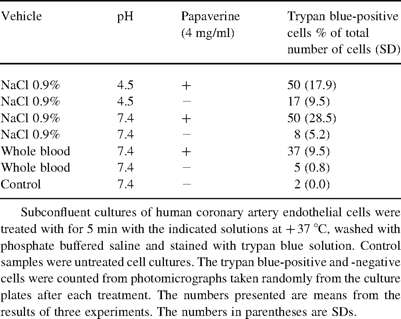
The effect of papaverine treatment on the viability of cultured human coronary artery endothelial cells
3 Results
3.1 Results of scanning electron microscopy
Total damage scores with medians, SD and P values are shown in Table 2 . We found that intraluminal papaverine treatment caused significant endothelial denudation (P<0.05), and a significantly increased number of intracellular pore-like structures (porosity) in the remaining endothelial cells (P<0.05). Some endothelial cells seemed disrupted and others seemed to be desquamating as intact cells, as if only the anchoring of the cell had been lost.
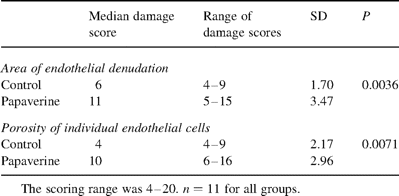
Effect of intraluminal papaverine treatment on endothelial denudation and on the porosity of individual endothelial cells
3.2 General methodological aspects
Incubation in heparinized autologous whole blood was well tolerated by the endothelium of all the segments studied. Control samples fixed within a few minutes after excision and control samples stored for a longer time in heparinized blood did not show any differences in the integrity of the endothelial layer or in cell morphology (Fig. 1 , Panels A–C). Damage scores of control segments and papaverine-treated segments did not change as a function of incubation time in heparinized blood. Tyrode's solution preserved endothelial cells in good morphological condition during fixation.
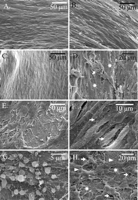
Scanning electron micrographs of human radial artery endothelium. Control samples were incubated only with heparinized blood and papaverine-treated samples were treated with intraluminal papaverine–NaCl solution for 5 min prior to incubation in heparinized blood. Control samples. Panel A: Morphologically intact endothelium. The sample was incubated in heparinized blood for 13 min. Patient number 2. Panel B: Morphologically intact endothelium. The sample was incubated in heparinized blood for 65 min. Patient number 11. Panel C: Morphologically intact endothelium. The sample was incubated in heparinized blood for 54 min. Patient number 10. Papaverine-treated samples. Panel D: Severely damaged endothelium with detaching endothelial cells (arrow) in the middle of a denuded area (asterisks). The sample was incubated in heparinized blood for 54 min after papaverine treatment. Patient number 10. Panel E: There were numerous large denuded areas and widened intercellular spaces in the papaverine-treated samples. The sample was incubated in heparinized blood for 65 min after papaverine treatment. Patient number 11. Panel F: Pore-like structures in individual endothelial cell (arrows). The sample was incubated in heparinized blood for 54 min after papaverine treatment. Patient number 10. Panel G: Platelets attached to the exposed subendothelial collagen. The sample was incubated in heparinized blood for 65 min after papaverine treatment. Patient number 11. Panel H: Damaged endothelium with locally denuded areas (asterisks), numerous widened intercellular spaces (arrows) and some fibrin strands (arrow-heads) attached to the exposed collagen. The sample was incubated in heparinized blood for 65 min after papaverine treatment. Patient number 11.
The pH measurement of the first 1 ml of outflowing mixture of blood and papaverine-solution was performed from three patients and the pH was 7.4 in each sample.
In general, the endothelial surface was flat and easy to evaluate, but many specimens showed longitudinal ridges and clefts that were most likely artifacts caused by vessel spasm, fixation and drying; they were easily detected and could be excluded from the analysis. In some of the samples, there were also significant atherosclerotic changes. The atherosclerotic areas, too, were excluded from the scoring, since the endothelial morphology in these areas was different from the healthy areas.
3.3 Morphology of the endothelium in control versus papaverine-treated samples
The endothelium in the control samples stored in autologous heparinized whole blood was mostly confluent throughout the whole sample (Fig. 1, Panels A–C). As in other arteries of similar size, two types of endothelial cell were present in the radial arteries: spindle-shaped and cobble stone-shaped cells. Occasionally, some small intercellular spaces as well as intracellular pore-like structures were observed in individual endothelial cells, and thrombocytes and fibrin strands were attached to the endothelial surface.
The intraluminally administered papaverine–NaCl solution (pH 4.2) markedly damaged the endothelium of the human radial arteries, although it was rapidly neutralized by the patient's blood after the intra-arterial injection and resided in the lumen for only 5 min. Thus, large areas of endothelium were lost in the papaverine-treated samples, and the subendothelial collagen mesh became exposed (Panel D). Intercellular spaces as well as pore-like structures in the remaining endothelial cells (Panels E and F, respectively) were more numerous and larger in the papaverine-treated than in the control samples. Attached platelets (Panel G) and fibrin strands (Panel H) were more common in the papaverine-treated than in the control samples. There were also small islets of confluent, apparently healthy looking endothelium in the papaverine-treated samples. Older patients had, on average, higher damage scores than younger ones.
3.4 Results of cell culture experiments
The main results of cell culture experiments are presented in Table 1. Papaverine caused damage to cultured HCAEC in all tested solutions. Furthermore, physiological NaCl-solution (pH 7.4 and 4.5) increased trypan blue staining of cultured HCAEC. Thus, both the low pH and direct toxic effects of papaverine seem to be responsible for the endothelial cell damage in this experimental setting.
Notably, at a pH 5.3–6.0 papaverine precipitates and forms sharp needle-like crystals. The precipitation causes drop of pH when papaverine is diluted with blood as shown in Fig. 2 .
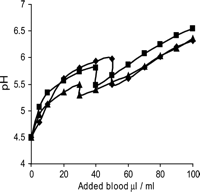
The change of pH of isotonic papaverine–NaCl solution (4 mg/ml) upon addition of heparinized blood. Each line represents one subject. The soluble papaverine precipitates and forms sharp needle like crystals at pH range of 5.3–6.0. The drop of pH in this pH range is caused by the precipitation. The same phenomenon applies also to crystalloid papaverine solutions at the same pH range.
4 Discussion
In the present study, we provide morphological evidence for endothelial damage of human radial artery grafts induced by papaverine–NaCl solution (pH 4.2) and also attempt to clarify the individual roles of low pH and direct papaverine cytotoxicy on the observed endothelial cell damage. Endothelial damage occurred in our experiments almost immediately after administration of the papaverine–NaCl solution and, therefore, this effect cannot be caused by the reported ability of papaverine to induce endothelial cell apoptosis [16]. Direct cytotoxic effects of papaverine itself [17], or the low pH of the solution [6,12], or their combined effect appear to explain the endothelial damage depending upon the solution used for diluting papaverine. Endothelial cells from rat arteries immediately suffered from plasma membrane disruptions when the cells were exposed to isotonic crystalloid solutions with a pH less than 4.2 [6], and the same may also apply to endothelial cells of human arteries. Acidic solutions may coagulate and precipitate the plasma membrane proteins. Once the cell membrane is ruptured, a rapid osmotic influx of the extracellular fluid into the cells ensues [6]. On the other hand, Rajasekaran et al. [17] reported significant, dose-dependent cytotoxicity of papaverine–0.9% NaCl to human smooth muscle cells in vitro (7.5–30 mg/ml, pH 4.1); however, no effects were detectable with lower papaverine concentrations at the same low pH of 4.1 [17].
The results of present morphological and cell culture experiments are in good agreement with previous reports on the toxicity of saline solution to endothelial cells [3,9], and on the impairment of endothelial function by different papaverine solutions [12,13]. Hillier et al. found no indications for endothelial dysfunction after short (less than 5 min) administration of intraluminal papaverine solution (composition and pH of the solution not reported) and subsequent recovery in an organ bath system [18]. Tatoulis et al. [19] observed increased reactivity to vasoconstrictors after papaverine-blood treatment, but no differences in endothelium-dependent vasorelaxation to acetylcholine in samples stored either in papaverine-blood or in Kreb's solution. The authors therefore concluded that papaverine-blood solutions do not affect the endothelium [19]. However, a recent study showed Kreb's solution also to be a graft storage solution that diminishes endothelial function significantly, and that acetylcholine may induce attenuated relaxation even when the endothelium is injured [3]. Thus, for reliable evaluation of the effects of pharmacological agents, functional parameters should be complemented by other data [3]. Finally, concentration-dependent loss of viability and increased apoptosis of endothelial cells have been observed in cell culture experiments after incubation with papaverine–NaCl solution (pH 4.8) when compared with saline solution of the same pH [16,20], suggesting that papaverine itself can damage the endothelium. This is consistent with our data from the cell culture experiments, which showed even papaverine-blood solution to harm endothelial cells. Toxicity of papaverine to endothelial cells is further corroborated by the present results, which show that endothelial cells tolerate heparinized blood well. This result is in good agreement with previous reports [3,9].
An intact endothelium is essential for normal vascular function, since the endothelium serves as a permeability barrier, regulating vascular tone, thrombus formation and inflammation of subendothelial tissues. Intracoronary thrombus formation has been associated with CABG operations [7]. There are also data showing that erosion of endothelium on coronary plaque may lead to thrombus formation and occlusion of the coronary artery [21]. Thus, it is likely that the denuded areas in arterial grafts make the grafts more prone to early post-operative thrombus formation [4]. In addition to increased risks of early postoperative spasm and thrombus formation, vessels with damaged endothelium are prone to develop atherosclerotic lesions in later life [4,22]. Thus, the severity of the damage to the endothelium and vessel wall seems to be a crucial determinant of the reaction of the radial artery to the CABG procedure.
The endothelium is capable of rapidly regaining its functional integrity after transient non-denuding injury and even after small denuding injuries. Small denuded areas of endothelium regenerate rapidly within a few days by proliferation and migration [23]. Re-growth to a denuded area proceeds at a speed of up to 0.4 mm/day and the maximal duration and extent of this re-growth seems to be species-specific [22]. Unfortunately, little is known about the duration and extent of endothelial regeneration in human arteries, but circulating endothelial progenitor cells may attach to denuded areas in autologous grafts, thereby reducing the time needed for covering the denuded area with new endothelium [24]. After more severe injuries, however, such as large denudation or denudation with damage to the intima or media, the reparative processes seem to be hampered [22]. Moreover, endothelial integrity may not be fully restored even by rapid regeneration, since there are marked functional and structural differences between native and regenerated endothelium. Regenerated endothelium exhibits impaired endothelium-dependent relaxation, signs of apoptosis, increased uptake of modified LDL, and giant endothelial cells typical of the senescence of endothelial cells [25]. In addition to alterations in regenerated endothelial cells, arterial smooth muscle cells under areas of transient endothelial denudation may also continue to proliferate at an increased rate even after completion of endothelial repair [23]. Since the degree of myointimal thickening is strongly correlated with the duration of endothelial denudation [22], the completion of endothelial repair should occur rapidly or, at best, no endothelial damage should occur at all during graft preparation.
4.1 Potential clinical relevance of the study
Taken together, the data presented in this study and the functional data presented by other groups show that papaverine appears to damage the endothelium regardless of pH and of the solution used for diluting it. Minor endothelial damage during the actual CABG procedure may be of little clinical relevance, whereas large denuding endothelial injuries, such as may be caused by administration of intraluminal papaverine–NaCl solution, could have some clinical relevance. Accordingly, use of alternative vasodilating drugs, such as combinations of calcium antagonists and nitrates, as suggested in a review by Rosenfeldt et al. [1] might be better tolerated. However, to our knowledge, no morphological studies on the effects of these alternative vasodilating drugs are available.
The results of morphological studies cannot provide answers to the important question of short- and long-term graft patency after its insertion. Thus, further evaluation of the vasorelaxing solutions used in bypass surgery is needed, and for evaluation of the effect of these vasorelaxing solutions on the patency of arterial grafts, randomized short- and long-term clinical trials are required.
We thank Pirkko Leikas-Lazányi and Mervi Lindman for technical assistance in the preparation of arterial samples for scanning electron microscopy, Mari Jokinen for technical assistance with the cell culture studies, and Kristian Pfaller (University of Innsbruck) for helpful discussions.