-
PDF
- Split View
-
Views
-
Cite
Cite
Sidney Levitsky, Jari Laurikka, Robert D. Stewart, Christian T. Campos, Steven J. Lahey, James D. McCully, Mitochondrial DNA deletions in coronary artery bypass grafting patients, European Journal of Cardio-Thoracic Surgery, Volume 24, Issue 5, November 2003, Pages 777–784, https://doi.org/10.1016/S1010-7940(03)00501-3
- Share Icon Share
Abstract
Objective: Mitochondrial DNA (mitoDNA) deletions have been shown to increase with aging and ischemia and have been suggested to contribute to myocardial dysfunction. The purpose of this study was to determine the prevalence and specificity of mitoDNA deletions in coronary artery bypass patients. Methods: Right atrial appendix tissue from 51 cardiac surgical patients (30–93 years; mean 64±14 years) was obtained during cardiopulmonary bypass cannulation (Control), just prior to the removal of the venous cannula (Ischemia, 169±38 min) and following removal of the cannula (Reperfusion) and used for polymerase chain reaction (PCR) and sequence analysis. Results: A novel mitoDNA deletion (approximately 7.3 kb, mitoDNA7.3) was found in three unrelated, male patients (53, 67, 75 years old). All mitoDNA7.3 deletion breakpoints were found downstream of the ATP synthase 8 genes and at the 3′ end of the cytochrome b genes. The prevalence of the mitoDNA7.3 deletion was significantly increased (P<0.05) following ischemia and reperfusion. Clinical data indicated that postoperative left ventricular ejection fraction was lower (38.3 vs. 46.4%), and the incidence of previous myocardial infarction higher (1.7 vs. 0.6) in patients exhibiting mitoDNA deletions. Conclusion: Our results reveal a novel mitochondrial DNA deletion occurring within the genome region coding for the mitochondrial genes of oxidative phosphorylation that is significantly increased during ischemia and reperfusion. The incidence and prevalence of mitoDNA7.3 deletions in patients with clinical indications of poor recovery suggests that mitoDNA7.3 deletions may provide an important indicator to surgical outcome in the cardiac surgical patient.
1 Introduction
Myocardial tissue is primarily aerobic and its metabolism is closely dependent upon oxygen, as confirmed by the abundance of mitochondria. The contractile process represents more than 75% of myocardial energy requirements, the remainder being mostly ionic homeostasis, which is essential for the contraction cycle. The high energy requirements for these processes are almost exclusively met by mitochondrial oxidative phosphorylation [1]. This leads to a high sensitivity of the myocardial cell to oxygen deficiency, and mitochondrial function is likely to play a central role in the molecular events leading to tissue damage occurring following ischemia and reperfusion [1].
Mitochondria are unique organelles, containing their own DNA encoding the mitochondrial subunits of the oxidative phosphorylation system. It has been speculated that damage to mitoDNA could be associated with the age-associated reduction in oxidation rate and energy production. A body of work now exists, based on animal models and cadaveric heart tissue samples, to suggest that mitochondrial DNA defects occurring either through inherited or acquired pathways are associated with cardiovascular abnormalities [2,3]. At present 12 predominant deletion types have been characterized in the human mitochondria, with all but one producing damage to the genes of oxidative phosphorylation (cytochrome oxidase, F0-F1 ATPase and the NADH-CoQ oxido-reductase genes). The most prevalent deletion occurring in various tissues, in human mitoDNA, is a 4977 bp deletion (mitoDNA4977), affecting 3–9% of the mitoDNA in 80–90-year-old patients [4].
Our previous work showing that mitochondrial enzyme and mRNA expression are decreased during global ischemia and are associated with decreased preservation and resynthesis of high energy phosphates suggested that alteration of mitochondrial genomic fidelity may compromise post-ischemic functional recovery [5,6]. To investigate this hypothesis right atrial tissue samples from cardiac surgical patients undergoing coronary artery bypass grafting were analyzed for mitoDNA deletions at various times during surgery and the specificity and sequence breakpoints of mitoDNA deletions determined. Our results indicate the presence of a novel large scale mitoDNA deletion (approximately 7.3 kb) occurring within the mitochondrial genomic region coding for the mitochondrial genes of oxidative phosphorylation. We also show that these deletions were apparent following ischemia and reperfusion and occur within specific breakpoints suggesting the presence of specific hotspots in mitoDNA. Our results indicate that this large-scale mitoDNA deletion is increased in patients with clinical indications of poor recovery, suggesting that mitoDNA deletions may provide an important indicator to surgical outcome in the aged cardiac surgical patient.
2 Materials and methods
2.1 Human atrial appendage samples
Tissue samples from right atrial appendage from 51 cardiac surgical patients undergoing coronary artery bypass surgery were obtained as discarded tissue during cardiopulmonary bypass cannulation. Both male and female patients were included. All patients received regional hypothermia through the application of ice slush to the pericardial cradle and received hypothermic (5 °C) cardioplegia and tepid (28–32 °C) perfusate. Three samples from the right atrial appendage (3×5 mm each) were obtained from each patient. Control samples were obtained at the time of insertion of a single venous cannula into the right atrium before snaring the purse-string suture around the right appendage. Ischemia samples were obtained just prior to removal of venous cannula, proximal to purse-string suture. Reperfusion samples were obtained after removal of venous cannula, proximal to purse-string suture. All specimens were obtained aseptically and placed in a sterile tube, in phosphate-buffered saline on ice (4 °C), and then immediately frozen in liquid nitrogen, prior to mitoDNA isolation and detection of mitoDNA deletions by polymerase chain reaction (PCR) analysis. All tissue samples were obtained under the approval of the Beth Israel Deaconess Medical Center Institutional Review Board (IRB protocol 96-0826-095E).
2.2 DNA isolation
Mitochondrial DNA was isolated according to the procedure as described by Tanaka and Ozawa [7]. Quantitation of DNA for PCR reactions was determined by fluorometry (Dynacount 200, Hoefer Scientific Instruments, San Francisco, CA).
2.3 PCR analysis
PCR analysis of mitoDNA was performed in a 100-μl reaction in thin-walled PCR reaction tubes containing 10× LA-PCR Buffer II (with 2.5mM Mg2+) (TaKaRa Shuzo Co. Ltd, Otsu, Japan), 2.5 mM of each dNTP, 1 nM of each primer, 70–100 ng of template DNA and 2.5 U of Taq polymerase (TaKaRa La-Taq, TaKaRa Shuzo) in a thermocycler (MJ Research Inc., Watertown, MA). After an 80 °C preincubation of all other reaction components for 10 min, and cooling to 4 °C, the DNA polymerase was added and the reaction continued for 40 cycles (denaturation 93 °C for 15 s, annealing 50 °C for 30 s, and extension 72 °C for 10 min) with each cycle increased by 1 s. A final extension at 72 °C for 10 min was performed and the reaction stored at 4 °C. The reaction products were resolved by 0.8% agarose gel electrophoresis and identified by ethidium bromide staining. The proportion of deleted mitoDNA as compared to the total mitoDNA was determined by scanning laser densitometry (LKB Ultrascan XL laser densitometer, Pharmacia LKB) using the LKB GelScan XL software program for 1-D analysis, to compare sample band densities against density values obtained from serial dilution of standards of known concentrations of DNA according to the method of Soong and Arnheim [8].
2.4 mitoDNA oligomers used for PCR analysis
mitoDNA oligomers were derived from reported human mitoDNA sequences [9]. Primers were obtained from Oligos Etc. (Wilsonville, OR). Primer sequences and locations in human mitoDNA are shown in Table 1 .
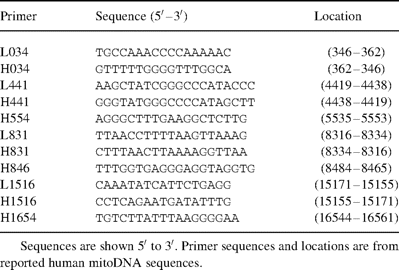
Oligonucleotide primers used for PCR amplification of human mitochondrial DNA
2.5 Identification of mitoDNA deletions
Preliminary identification of deleted mitoDNA was determined by using the primer shift PCR method of Tanaka and Ozawa [7]. Primer pairs used were: L034 (346–362) and H441 (4432–4419) to yield a 4086 bp product; L441 (4419–4432) and H554 (5553–5538) to yield a 1134 bp product; L441 (4419–4432) and H846 (8484–8465) to yield a 4.065 bp product; L831 (8316–8334) and H1516 (15 171–15 155) to yield a 6855 bp product; L831 (8316–8334) and H1654 (16 561–16 544) to yield a 8245 bp product; L1516 (15 155–15 171) and H034 (362–346) to yield a 1760 bp product; L831 (8316–8334) and H034 (362–346) to yield a 8615 bp product; and L1516 (15 155–15 171) and H831 (8334–8316) to yield a 9748 bp product. Quantitative determination of deleted mitoDNA relative to the total amount of mitoDNA was determined using the primer shift PCR protocol and serial dilution as described by Zhang et al. [10]. Confirmation and identification of mitoDNA deletion breakpoints was determined by subcloning of mitoDNA deletion products into the T/A vector (Invitrogen, San Diego, CA).
Following antibiotic and enzymatic selection, plasmids containing the mitoDNA deletion products were sequenced using standard techniques. Sequence analysis was performed using the DNASIS Analysis Program (Hitachi, San Bruno, CA).
2.6 Statistical analysis
Statistical analysis was performed using SAS (version 6.12) software package (SAS Institute, Cary, NC). The mean±standard deviation for all data was calculated for all variables. Unequal variance was controlled by appropriate data transformation. A two-way analysis of variance (with repeated measures, where appropriate) to compare grouped data. Post hoc testing was done after residual analysis using the Tukey test. Simple pre- vs. postinterventional comparisons were made using either a paired or unpaired two tailed Student's t-test and Chi square. Statistical significance was claimed only at P<0.05.
3 Results
3.1 Patient population and preoperative profiles
Right atrial appendix tissue was obtained from 51 unrelated cardiac surgical patients (30–93 years; mean 64±14 years). All patients undergoing coronary bypass grafting were accepted into the study. Patient preoperative profiles are shown in Fig. 1 .

Age distribution and preoperative profiles of cardiac surgery patients undergoing coronary artery bypass surgery enrolled in the study.
3.2 mitoDNA deletions
Mitochondrial primers were used for the complete amplification of mitoDNA (Fig. 2) . Only one patient had a mitoDNA deletion in a Control sample. This patient, a 67-year-old male, was found to have an approximately 7.3 kb mitoDNA deletion located within the primer locations at bp 8316–8334 (L831) and bp 16 544–16 561 (H1654). The prevalence of this deletion was determined to be 0.0025% of total mitoDNA. No other mitoDNA deletions were observed in any of the Control samples (results not shown).
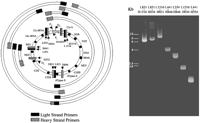
The mitochondrial genome is shown on the left. Mitochondrial genes are indicated. D-Loop, displacement loop; Cyt b, cytochrome b; ND6, NADH dehydrogenase-6; ND5, NADH dehydrogenase-5; ND4, NADH dehydrogenase-4; ND4L, NADH dehydrogenase-4L; ND3, NADH dehydrogenase-3; COIII, cytochrome c oxidase III; ATPase 6, F0-F1 ATPase 6; ATPase 8, F0-F1 ATPase 8; COII, cytochrome c oxidase II; COI, cytochrome c oxidase I; ND2, NADH dehydrogenase-2; ND1, NADH dehydrogenase-1; OL, light strand origin; OH, heavy strand origin; PL, light strand promoter; PH, heavy strand promoter. Light (L) and heavy (H) strand primers are shown in their relative locations. Amplified PCR products are shown. A representative gel showing amplified PCR products from atrial tissue obtained from a representative patient undergoing coronary artery bypass grafting is shown on the right. The light (L) and heavy (H) chain oligonucleotide primers used in each reaction are noted above each lane. Molecular size markers are indicated.
mitoDNA deletions were present in three unrelated male patients aged 53, 67 and 75 years of age following global ischemia (Ischemia; Fig. 3) . The prevalence of these deletions was significantly increased (P<0.05 vs. ischemia) during reperfusion (Fig. 4) . The apparent size of these deletions was the same in all cases being approximately 7.3 kb in size and located within the primer locations at bp 8316–8334 (L831) and bp 16 544–16 561 (H1654). No other mitoDNA deletions were observed in any of the patients investigated.
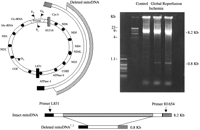
A representative gel showing amplified PCR products from atrial tissue obtained from a 75-year-old male. Samples were obtained at Control, obtained at the time of insertion of a single venous cannula to the right atrium before snaring the purse-string suture around the right appendage; Ischemia, obtained just prior to removal of venous cannula, distal to purse-string suture; and Reperfusion, obtained after removal of venous cannula, proximal to purse-string suture. Primers L831 and H1654 were used to detect the presence of mitoDNA deletions within the oxidative phosphorylation genes. In the case of no deletion the reaction yielded only an 8.2 kb reaction product. In the case of a mitoDNA deletion the 8.2 kb product and smaller reaction product would be evident. In this patient the 8.2 kb product is seen with an approximately 0.8 kb product indicating the presence of a mitoDNA deletion in Ischemia and Reperfusion samples. The 0.8 kb product indicates an approximate 7.3 kb mitoDNA deletion (mitoDNA7.3). Molecular weight markers are indicated. The deletion breakpoints and the deleted genes are shown on the left. The prevalence of the mitoDNA7.3 deletion in this patient was determined to be 0.0039% of total mitoDNA in ischemia and 0.0059% of total mitoDNA in reperfusion.
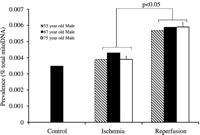
Prevalence of mitochondrial deletions (approximately 7.3 kb) shown as a percent of total mitoDNA in cardiac surgical patients undergoing coronary artery bypass surgery. A mitoDNA deletion (approximately 7.3 kb) was evident in one patient, a 67-year-old male, in Control, and was increased in Ischemia and Reperfusion. mitoDNA deletions (approximately 7.3 kb) were also found in two unrelated males, aged 53 and 75, and were increased in Ischemia and Reperfusion.
3.3 Identification of mitoDNA deletions
Sequence analysis of mitoDNA deletion products indicated that all mitoDNA deletion products were of mitochondrial origin and that mitoDNA breakpoints were located within the mitochondrial genome region coding for the mitochondrial genes of oxidative phosphorylation (Fig. 5) . mitoDNA deletion breakpoints were located in the ATP synthase 8 gene and in the cytochrome b gene. Four direct repeat sequences were found flanking the mitoDNA deletions. Also found flanking the mitoDNA breakpoints were AT-rich sequences. The 13 bp repeat sequence previously shown to be associated with Kearns Sayre syndrome (KSS), Chronic progressive external ophthalmoplegia (CPEO) and ocular myopathies was present but is not repeated in the sequence proximal to the 3′ end of the cytochrome b gene and the 5′ end of the tRNAThr gene [11].
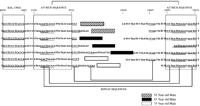
Sequence of the 0.8 kb reaction products from affected patients with mitoDNA7.3 deletions. Breakpoints occur in the ATP synthase 8 gene at bp 8516, 8517, 8524, 8534 and 8544 and in the cytochrome b gene at bp 15 829, 15 831, 15 836, 15 845 and 15 852). Four direct repeat sequences (AAATA, double underlined; CCCT, underlined; CCAA and AAC/TGAAAA, underlined) are found flanking the mitoDNA deletions. These repeat sequences are within or directly flank the AT rich sequences (shown in box) located at 8488–8510 (96% AT rich) containing the AATGAAAA and AAATA repeats and at 15 855–15 874 (88% AT rich) containing the CCCT, AATGAAAA and AAATA repeats. The 13 bp repeat sequence previously shown to be associated with KSS, CPEO and ocular myopathies (84700–8482) is shown in boxed outline.
3.4 Postoperative profiles of patients with mitoDNA deletions
mitoDNA deletions were found only in men. In comparison with other men within the cohort there was no significant difference in age between patients with mitoDNA deletions (65±11) and patients without mitoDNA deletions (64.4±15.5). Coronary angiographic data did not refer to any typical pattern of coronary artery disease with one patient having had left main stenosis (50%), one patient having two-vessel disease (LAD, RCA), and one patient having three-vessel disease (left anterior descending coronary artery (LAD), left circumflex artery, right coronary artery (RCA)). However, the number of grafts was higher in men exhibiting the mitoDNA7.3 deletion (4.3±0.6 vs. 3.7±1.6); cardioplegia volume was higher (6740 vs. 6059 ml); aortic cross-clamp time was longer (112 vs. 100 min); postoperative left ventricular ejection fraction was lower (38.3 vs. 46.4%) and the number of myocardial infarctions was higher (1.7 vs. 0.6) as compared to men without the mitoDNA7.3 deletion (Fig. 6) . Length of stay was paradoxically shorter in the patient group exhibiting mitoDNA7.3 deletions (4.7±0.6 vs. 6.6±2.0 days). There was only one death in the patient cohort and this patient did not exhibit any mitoDNA deletions.
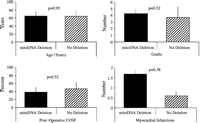
Postoperative profiles of patients with mitoDNA deletions as compared to patients with no deletions. Significance levels are shown for each parameter.
4 Discussion
Previous research by others, investigating the prevalence of mitoDNA deletions in the human myocardium have utilized autopsy tissue [10–12] cadaveric tissue [13–17], or have used sample tissue from patients exhibiting pre-existing pathological sequelae or degenerative diseases known to be associated with mitoDNA deletions [12,14,16,17]. Estimates of the prevalence of these deletions in the human range from 0.00015 to 25%. Corral-Debrinski et al. [12] have shown that the mitoDNA4977 deletion was apparent in control hearts by age 40 years; however this estimate was based on three of seven samples (0.00015, 0.00255 and 0.0035% of total mitoDNA) obtained from autopsy material. Hayakawa et al. [15] have reported that in cardiac tissue obtained from autopsy (n=17) that a mitoDNA7436 deletion with different breakpoints than that observed in our study was present in 7% of heart samples. Hattori et al. [18] have shown that in 47 human cadavers with a mean age of 57 years that the incidence of the mitoDNA7436 deletion increased with age occurring at an incidence of 25% in cardiac samples obtained from cadavers aged 31–40; 33% in cardiac samples obtained from cadavers aged 41–50; 63% in cardiac samples obtained from cadavers aged 51–60; and increasing to 75% in cardiac samples obtained from cadavers aged 61–70. Notably these authors found that a mitoDNA7436 deletion was present in all cardiac samples obtained from cadavers over 71 years of age. The breakpoints for this mitoDNA7436 deletion were located in the ATPase 6 gene and in the D-loop while all our mitoDNA deletions were found to have breakpoints in the ATP synthase 8 gene and in the cytochrome b gene.
In our study, using tissue from living patients only a novel mitoDNA7.3 deletion was detected. No other deletions were found in our sample population. The age associated mitoDNA4977 deletion was not detected in any of our patient group even though the mean age within our cohort was 64±14 years and included two patients aged 91 and 93 years of age. Our data would agree with Biagini et al. [19] who also failed to detect the mitoDNA4977 deletion in platelets from aged patients without pre-existing pathological sequelae or degenerative diseases associated with mitoDNA deletions. It should be noted that tissue procurement from living cardiac surgical patients, necessarily precludes specimens originating from regions which may deleteriously affect myocardial function. In a previous investigation using autopsy tissue the prevalence of mitoDNA deletions in the right atrium was estimated to be <left atrium<right ventricle<left ventricle [12]. The use of atrial tissue in our study may therefore underestimate the prevalence of this mitoDNA deletion.
In our study the mitoDNA7.3 deletions all had breakpoints in the ATP synthase 8 gene and in the cytochrome b gene. These breakpoints are directly flanked by 23 and 18 bp sequences that are 96% and 72% AT-rich, respectively (Fig. 5). Four direct repeat sequences AAATA, CCCT, CCAA and AAC/TGAAAA, are found flanking the mitoDNA deletions. These repeat sequences are within (AAATA and AAC/TGAAAA) or directly flank (CCCT and CCAA) the AT-rich sequences. The AT-rich sequence located at 8488–8510 is located 125 bp 3′ of the tRNALys gene (8295–8364) with the direct repeat sequences located from 130 to 166 bp from the tRNALys gene. The AT-rich sequence located at 15 855–15 874 is located 14 bp from the tRNAThr gene (15 888–15 953) with the direct repeat sequences (CCAA; CCCT; AATGAAAA and AAATA) located from 40 to 15 bp from the tRNAThr gene. The specificity of the breakpoints in our study would suggest that these regions may be potential hotspots for mitoDNA deletion. This is in agreement with Van Tuyle et al. [20] who have previously shown that the junction between the 3′ end of the cytochrome b gene and the 5′ end of the tRNAThr gene is a major hotspot for mitoDNA deletion in the rat and the region surrounding the tRNALys-ATP synthase 8 genes is a minor hot spot. Our data confirm these earlier observations. Of interest is the presence of the 13 bp sequence (8470–8482) proximal to the 5′ deletion breakpoint located 106 bp from the tRNALys gene. This sequence has been previously identified to be associated with KSS, CPEO and ocular myopathies [11], while this sequence is not repeated proximal to the 3′ end of the cytochrome b gene and the 5′ end of the tRNAThr gene its presence lends further support that the region flanking the tRNALys-ATP synthase 8 genes is a hotspot.
4.1 Putative mechanism for mitoDNA deletions
Previous investigators have indicated that mitochondrial tRNA genes exist as single strand segments in early replicative intermediates, allowing for the formation of tRNA structures and that the formation of these structures plays an important role in the deletion process [21]. The location of our breakpoints and those previously described [22] suggest that proximity to tRNA coding regions may be important in the genesis of mitoDNA deletions in aging. Our data also suggest that AT-rich sequences containing repeat sequences directly flanking these breakpoints may be involved in the mechanism leading to mitoDNA deletion at these sites by promoting strand separation. These distortions in mitoDNA helix structure have been previously suggested to contribute to the generation of mitoDNA deletions [20].
4.2 Relevance of mitoDNA deletions to cardiac surgery
At present the technology to allow for immediate real time analysis of mitoDNA deletions to allow for perioperative management is unavailable. The rapid developments in all areas of molecular biology and the increasing relevance of genetics in surgery would suggest that these forms of analysis will play an important role in cardiac surgery in the future. The specificity of mitoDNA deletions in our study is particularly relevant to cardiac surgery. The mitoDNA7.3 deletion results in the deletion of the cytochrome b gene, the NADH dehydrogenase (ubiquinone) chain 6, 5, 4, 4L and 3 genes and the F0-F1 ATPase 6 and 8 genes. These genes are essential for oxidative phosphorylation and their deletion would be expected to decrease ATP resynthesis during reperfusion. In previous studies using serial 31P nuclear magnetic resonance we have shown that global ischemia is associated with significantly decreased high energy phosphate preservation and resynthesis [23]. In this study we were unable to acquire samples postoperatively to measure the effects of the mitoDNA7.3 deletion on oxidative phosphorylation and specific mitochondrial enzyme activity as compared to patients without of mitoDNA deletions. The large deletion of essential genes of mitochondrial oxidative phosphorylation would be expected to decrease high-energy phosphate preservation and resynthesis and detrimentally effect postischemic functional recovery. Our data suggest that the incidence and prevalence of mitoDNA deletions is small but is increased in patients with clinical indications of poor recovery. In total, these data suggest that this novel mitoDNA deletion may provide an important indicator to surgical outcome in the aged cardiac surgery patient.
This study was supported by the National Institutes of Health (HL 59542, HL 29077) and the American Heart Association.
References
Author notes
Present address: Department of Surgery, Tampere University Hospital, Tampere, Finland.
- aging
- myocardial infarction
- polymerase chain reaction
- ischemia
- left ventricular ejection fraction
- coronary artery bypass surgery
- cardiopulmonary bypass
- mitochondria
- atrium
- reperfusion therapy
- physiologic reperfusion
- myocardial dysfunction
- catheterization
- cytochromes b
- dna, mitochondrial
- genes
- genome
- oxidative phosphorylation
- sequence analysis
- surgical procedures, operative
- heart
- surgical outcome