-
PDF
- Split View
-
Views
-
Cite
Cite
Zhong-Kai Wu, Tiina Iivainen, Erkki Pehkonen, Jari Laurikka, Matti R. Tarkka, Perioperative and postoperative arrhythmia in three-vessel coronary artery disease patients and antiarrhythmic effects of ischemic preconditioning, European Journal of Cardio-Thoracic Surgery, Volume 23, Issue 4, April 2003, Pages 578–584, https://doi.org/10.1016/S1010-7940(03)00007-1
- Share Icon Share
Abstract
Objective: Sudden cardiac death caused by arrhythmia remains a major unsolved problem after coronary artery bypass grafting (CABG). Ischemic preconditioning (IP) has proved effective in suppressing ischemia reperfusion arrhythmias in animals and humans. The purpose of the present study was to establish whether IP reduces postoperative arrhythmias in three-vessel coronary artery disease patients undergoing CABG. Methods: Forty-five patients with stable angina and three main coronary artery stenosis admitted for primary CABG surgery were randomized into an IP and a control group. The IP protocol entailed twice occluding the ascending aorta by cross-clamping for 2 min, followed by 3 min of reperfusion. Electrocardiography was continuously recorded from the day before surgery to the second postoperative day. Results: During the recording, all patients developed SVES and VES after the operation. The incidences of SVT and ventricular tachycardia (VT) after surgery were 73.3 and 77.8%, respectively. IP significantly reduced VES events per hour during 2 h after reperfusion. The SVT and VT incidence and events per hour were significantly lower in the IP group during 2 h after reperfusion and 24 h later. Conclusions: IP significantly reduced VES, SVT, and VT after surgery. The antiarrhythmic effect 24 h after surgery indicates a delayed antiarrhythmic IP phenomenon in these patients. These findings would indicate that IP constitutes a potential additional myocardial protective strategy in multi-vessel diseased patients undergoing CABG.
1 Introduction
Sudden cardiac death remains a major unsolved clinical problem after cardiac surgery. Most cases are caused by acute ischemia or reperfusion-induced ventricular tachycardia (VT) or fibrillation (VF) [1]. Ischemic heart disease carries an increased risk of malignant VT and VF and sudden cardiac death [2,3]. Relief of underlying ischemia by coronary artery bypass grafting (CABG) eliminates susceptibility to arrhythmias, whereas CABG also appears to exert effects on the arrhythmogenic substrates [2]. Most postcardioplegic reperfusion arrhythmias are generally considered to be benign transient events. However, severe arrhythmias may be associated with some serious complication with an unsatisfactory prognosis [2–5]. Though postoperative arrhythmias have been thought to be multifactoral, incomplete myocardial protection is a major cause [6,7]. Postoperative arrhythmias are, therefore, considered to be a manifestation of ischemia reperfusion injury and are often used in comparisons of myocardial protection strategies in cardiac surgery [3].
Single or repeated brief periods of myocardial ischemia followed by reperfusion increases myocardial tolerance to subsequent prolonged ischemia insult. This paradoxical phenomenon was first described and termed ischemic preconditioning (IP) by Murry and colleagues [8]. IP has also proved effective in preserving high-energy phosphate [9], improving heart performance [10,11] and reducing cardiac troponin T and isoenzyme of creatine kinase containing M and B subunits (CK-MB) release [12] during open-heart surgery. The protective effect might be triggered by free radicals which are generated during the IP protocol [13]. However, the precise mechanism involved is as yet unclear; as derived from an animal model it might also not totally fit the clinical setting. Studies of IP in protecting against ischemia reperfusion injury in cardiac surgery are still controversial [14].
In fact, before the definition of IP by Murry and colleagues, Gulker and colleagues have reported that a regional brief coronary occlusion and reperfusion may increase the VF threshold and exert a protective effect against ischemia reperfusion arrhythmia [15]. Recent experimental studies have further proved that IP reduces ischemia reperfusion arrhythmias in different animal models [16–21]. There is also evidence of antiarrhythmic effects of IP in the human heart [22–24]. Whether IP protects the heart from ischemia reperfusion arrhythmias in open-heart surgery has not been studied.
The proportion of arrhythmia detection depends on the patient's profile, the type of surgery, the method and duration of arrhythmia surveillance, and the definition of arrhythmia [6,7]. Twenty-four-hour continuous electrocardiography (24-h ECG) recording detects all arrhythmic events and increases the rate of detection compared with conventional ECG monitoring [6,7]. The purpose of the present study was to establish whether IP protects the heart from ischemia reperfusion-related arrhythmias recorded continuously by 24-h ECG in three-vessel disease patients undergoing CABG.
2 Material and methods
The study design was accepted by the Ethical Committee of Tampere University Hospital, Finland, and informed consent was obtained from all patients. Forty-eight patients with stable angina and three main coronary artery stenosis admitted for CABG were randomized into two groups: a control group using routine myocardial protection methods with cold blood cardioplegia, and a study (IP) group receiving IP before cross-clamping. Two patients in the control group and one in the IP group were excluded due to unnoticed accidental disconnection of the wires or malfunction of the recording apparatus in 24-h ECG. This left a final study cohort of 45 patients (23 cases in the IP and 22 in the control group). Patients with low ejection fraction (EF, <40%), unstable angina, recent myocardial infarction (<3 months), additional cardiac diseases, severe non-cardiac diseases and calcified or dilated ascending aorta were excluded.
The preoperative characteristics of the patients in the respective groups were similar; there was no statistically significant difference in patients' age, sex, NYHA class, preoperative left ventricular ejection fraction (LVEF), vessels diseased, history of myocardial infarction, and preoperative medications between the groups (Table 1 ).
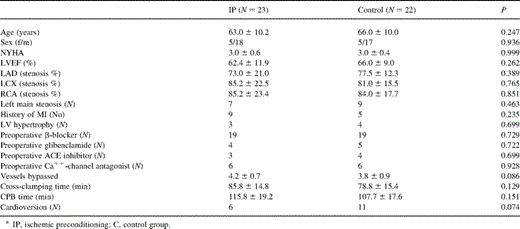
2.1 Preconditioning protocol
After creating cardiopulmonary bypass (CPB) and run pump to vent the heart, the ascending aorta was occluded by cross-clamping for 2 min, followed by 3 min reperfusion, the procedure being repeated once. The control group also had CPB for 10 min before the routine operation. The temperature was kept normothermic during this period.
2.2 Anesthesia, CPB, and surgical technique
A standardized anesthetic technique was used with sufentanil (7.8 μg/kg), midazolam (0.10–0.125 mg/kg for induction and 0.20 mg/kg for maintenance) and pancuronium (0.22 mg/kg). CPB with non-pulsatile perfusion flow (2.2–2.4 l/min/m2) was conducted using membrane oxygenators with arterial line filtration. Mild hypothermia (32°C) was maintained without topical cooling.
Surgical techniques were the same in all cases. Aortic root and two-stage single venous cannula were used for CPB. A retrograde, self-inflating coronary sinus cardioplegia cannule (RC014, Research Medical Inc., Utah) with a pressure monitoring port was guided into place. A nine-gauge cannule was placed in the aortic root for antegrade cardioplegia, or for venting. Distal anastomoses were made in the order right coronary artery (RCA) – circumflex coronary artery (CX) – left anterior descending coronary artery (LAD). The proximal anastomoses were constructed during cross-clamping. Left internal mammary artery (LIMA) to LAD was used in all patients. Electric cardioversion was performed after declamping when the sinus heart rate had not recovered.
Blood from the pump reservoir was mixed with crystalloid in a ratio of 4:1, yielding a cardioplegia solution with a 0.21 hematocrit value and 21 mmol/l potassium concentration in the initial and 9 mmol/l in subsequent doses. In antegrade delivery, cardioplegia was administered at a pressure of 80 mmHg and in retrograde 30–50 mmHg, with a flow of at least 200 ml/min. The initial high-potassium cardioplegia was given 1.5 min antegrade, then 2.5 min retrograde, at a temperature of 6–9°C. One minute was given retrograde and given to RCA and left circumflex (LCX) area grafts after each distal anastomosis. Warm cardioplegia (37°C) was given retrograde for 3 min before release of cross-clamping.
2.3 Measurements and data collections
Data from 24-h ECG were recorded continuously 1 day before the operation, during the operation, and up to the second postoperative morning. Recordings of 24-h ECG were made with two-channel tape recorders (Oxford Medilog MR 4500-3) with recording speed of 1 mm/s and sampling rate 0.045–70 Hz. The recordings were analyzed by software of the Oxford Medilog Holter Management System Excel 2 (Rel 8.5 version, UK). Software-analyzed classification of QRS complexes and events were checked on the computer screen and corrected manually. The analysis was blinded to the results of other investigations. The data were preserved and analyzed in the same period with the same standard. Analysis of the recording tapes was divided into five periods: (T1): preoperative data from the preoperative day to the morning of operation; (T2): during IP protocol or 10 min of CPB before cross-clamping; (T3): 2 h after 10 min of reperfusion; (T4): in the ICU, from 2 h after declamping till the first postoperative morning (T5): in the ICU, from the first to the second postoperative morning.
Supraventricular rhythm disturbances were noted as extra systoles (SVES) or tachycardia (SVT); SVT was not further divided into atrial fibrillation, atrial flutter or non-sinus atrial tachycardia. VT was defined as extra systoles (VES) and tachycardia (VT). The criterion for both SVT and VT was at least three abnormal complexes in sequence with a rate exceeding 100/min. Arrhythmia was registered as positive if any event appeared. The arrhythmic events per hour were calculated quantitatively to evaluate the severity of arrhythmia in each patient.
Volume infusion was aimed to maintain filling pressure at least at preoperative level. Pharmacological therapy with inotropes was used to keep the CI greater than 2.0 l/min/m2; this was not interrupted at the time points when hemodynamic data were measured. Dopexamine or adrenaline was used as first choice inotrope and amrinone with noradrenaline if necessary. β-Blocker was continued after weaning from the respirator. The cardiologist on duty undertook antiarrhythmic intervention, including medication and pacing when needed. Perioperative infarction was diagnosed if any new Q wave appeared with one-third QRS height and for longer than 0.04 s or CK-MB passed beyond 100 μg/l. The ICU team and cardiologists were blinded in the management of postoperative care.
2.4 Statistics
The severity (events per hour) and incidence (patients with such episodes) of arrhythmias were analyzed between the two groups. Unpaired Student's t-test was used for continuous data (two-tails) and Chi-square test or Fisher's exact test for categorical data when comparing variables between the groups. Mann–Whitney U-test was used for skewed distributions. Data are presented as mean±standard deviation (SD). Level of significance was set at 0.05. The statistical analyses were performed using a SPSS/Win (version 9.0) statistical package program.
3 Results
3.1 Outcome of surgery
There was no perioperative myocardial infarction or early postoperative death in either group. Intra-aortic balloon pump was not required in any case. The period of mechanical ventilation and the length of stay in ICU were similar in both groups. More patients in the IP group were free of inotropes with marginal statistical significance. The period of inotropic medication was also marginally shorter in the IP patients than in the controls. There were one IP and three control patients needing antiarrhythmic intervention. One control patient accepted amiodarone during T4 and was successfully converted into sinus heart rate. Other patients accepted antiarrhythmic interventions during T5 (Table 2 ). Patients with longer mechanical ventilation periods (>24 h) showed more postoperative VES events per hour (44.3±60.3 vs. 11.2±27.0, P=0.014) and SVT events per hour (0.15±9.90 vs. 0.03±0.04, P=0.003), but were similar in postoperative SVES and VT events. Postoperative arrhythmic events per hour were similar in patients with and without inotropic supports.
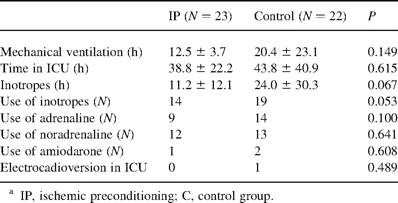
3.2 24-h ECG data
The mean continuous ECG was recorded for 14.1±2.5 h during T1, 18.7±2.0 h during T4 and 23.4±2.0 h during T5. IP protocol, CPB, and cardioplegic ischemia reperfusion injury were significant causes of elevation of HR. There was no statistically significant differences in HR between the groups (Fig. 1 ).
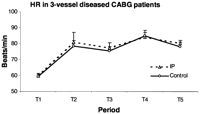
Mean heart rate during each time period in the IP and the control group. IP, ischemic preconditioning; T1, from the preoperative day to the morning of operation; T2, during IP protocol or 10 min of CPB before cross-clamping; T3, 2 h after reperfusion; T4, in the ICU, 2 h after reperfusion till first postoperative morning; T5, in the ICU, from first to second postoperative morning. Data presented as mean±SEM.
There was a high incidence of patients with SVES or VES before (100 and 86.7%) and after (100 and 100%) surgery. The incidence of SVT and VT was lower before surgery but significantly increased after surgery (28.9–73.3%, P<0.001 and 31.1–77.8%, P<0.001). IP significantly suppressed the incidence of SVT and VT at 2 and 24–48 h after reperfusion (Table 3 ).
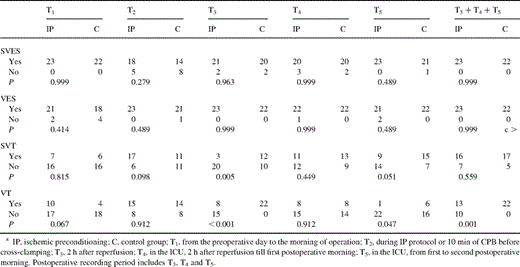
IP induced more SVES, VES, and SVT events per hour during T2 without statistically significant difference (Figs. 2–4 ). VT events per hour during T2 were marginally more frequent (P=0.051, Fig. 5 ). Similar postoperative SVES events per hour were found between the groups (P=0.446, analysis with repeated measures, Fig. 2). Postoperative VES events per hour in IP patients were significantly reduced during T3 (Fig. 3). IP significantly suppressed postoperative SVT and VT events per hour during T3 and T5 but not during T4 (Figs. 4 and 5).
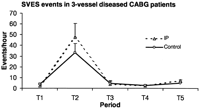
The severity of SVES in three-vessel diseased CABG patients is shown as SVES events per hour during each time period. IP, ischemic preconditioning; T1, from the preoperative day to the morning of operation; T2, during IP protocol or 10 min of CPB before cross-clamping; T3, 2 h after reperfusion; T4, in the ICU, 2 h after reperfusion till first postoperative morning; T5, in the ICU, from first to second postoperative morning. Data presented as mean±SEM.
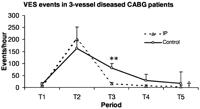
The severity of VES in three-vessel diseased CABG patients is shown as VES events per hour during each time period. IP, ischemic preconditioning; T1, from the preoperative day to the morning of operation; T2, during IP protocol or 10 min of CPB before cross-clamping; T3, 2 h after reperfusion; T4, in the ICU, 2 h after reperfusion till first postoperative morning; T5, in the ICU, from first to second postoperative morning. Data presented as mean±SEM. Significance of difference between the groups by Student's t-test, the symbol (**) represents P<0.01. Significance of difference between the groups by GLM analysis with repeated measures, the symbol (††) represents P<0.01.

The severity of SVT in three-vessel diseased CABG patients is shown as SVT events per hour during each time period. IP, ischemic preconditioning; T1, from the preoperative day to the morning of operation; T2, during IP protocol or 10 min of CPB before cross-clamping; T3, 2 h after reperfusion; T4, in the ICU, 2 h after reperfusion till first postoperative morning; T5, in the ICU, from first to second postoperative morning. Nature logarithm (LN) of values during IP protocol (T2) was used to fit the scale of the figure. Data presented as mean±SEM. Significance of difference between the groups by Student's t-test, the symbol (*) represents P<0.05 and (**) represents P<0.01. Significance of difference between the groups by GLM analysis with repeated measures, represented by (††) P<0.01.
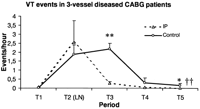
The severity of VT in three-vessel diseased CABG patients is shown as VT events per hour during each time period. IP, ischemic preconditioning; T1, from the preoperative day to the morning of operation; T2, during IP protocol or 10 min of CPB before cross-clamping; T3, 2 h after reperfusion; T4, in the ICU, 2 h after reperfusion till first postoperative morning; T5, in the ICU, from first to second postoperative morning. Nature logarithm (LN) of values during IP protocol (T2) was used to fit the scale of the figure. Data presented as mean±SEM. Significance of difference between the groups by Student's t-test, the symbol (*) represents P<0.05, (**) represents P<0.01. Significance of difference between the groups by GLM analysis with repeated measures, represented by (††) denoting P<0.01.
4 Discussion
The mechanism of postoperative arrhythmias includes reentry, enhanced automacity and triggered activity [17]. Transmural reentry is the most likely mechanism underlying ischemia reperfusion arrhythmias [17]. Evidence suggests that both oxygen-derived free radicals and transient calcium overload play important pathologic roles in arrhythmogenesis after cardiac surgery [1]. Though postoperative arrhythmias have been thought to be multifactoral, incomplete myocardial protection is a major cause [6,7]. The vulnerability to reperfusion-induced arrhythmias is critically dependent on the duration of the preceding ischemia [18]. Because myocardial ischemia may be an important factor in the genesis of arrhythmias, postcardioplegic arrhythmias have been considered one of the variables in comparing strategies for myocardial protection [3]. Hence the search for better myocardial protective strategies in order to reduce postoperative arrhythmias is both realistic and necessary.
The present results show that a variety of arrhythmias appeared immediately after reperfusion, a finding in agreement with previous knowledge [25]. All patients here developed SVES and VES during the first 48 h after declamping. The cumulative incidence of SVT and VT after surgery was 73.3 and 77.8%, respectively. These figures are much higher than those in previous reports [24,25], but similar to our previous studies [6,7]. Supraventricular arrhythmias increased but ventricular arrhythmias decreased from the early to late reperfusion period. VES events were more common than SVES events. This is at odds with previous findings showing supraventricular arrhythmias to be more common than ventricular arrhythmias [25]. The high incidence in the present study may reflect the existence of RCA stenosis and the use of retrograde cardioplegia which are known to predispose to tachycardia, and the detection method of 24-h ECG [7]. Even though a high percentage of the patients here had tachyarrhythmias, the numbers of episodes in each patient were small. This may be one reason why postoperative tachycardia easily goes undetected if continuous ECG recording is not used [6].
IP has been found to have favorable effects on inhomogeneous reduction of action potential and refractory period duration, or change conduction velocity so as to reduce reentrant arrhythmia [23]. In animal models, IP did not affect action potential, but attenuated the depression of transmural conduction caused by ischemia and early reperfusion and thereby prevented conduction delays necessary for transmural reentry. The inward calcium channel responsible for conduction during acute ischemia could be attenuated by IP and in turn suppress abnormal automacity [17]. Change in ATP-dependent potassium and sodium channel excitability may be the biocellular basis of antiarrhythmic IP effects [19]. In the present study, IP reduced the incidence of SVT and VT. VES, SVT, and VT events were also suppressed by IP. Our result was similar to those of previous animal studies and in humans [16–23].
The early antiarrhythmic preconditioning effect occurs within minutes and lasts for 1–3 h. Delayed antiarrhythmic preconditioning occurs 24 h after preconditioning stimuli and may extend to 72 h [16,19,21]. It is interesting to note here that the IP effect in reducing SVT and VT occurred during the early reperfusion period (within 2 h) and also during the delayed period (from first to second postoperative morning). These time-dependent effects fit the time-course of early and delayed IP phenomena shown in previous studies [16,19,21]. Tachyarrhythmia episodes have been proved to be related to recent myocardial ischemia or infarction [2,3,6,7]. Some studies have also shown that myocardial ischemia remains common even after successful revascularization, and current techniques cannot rule out ischemia of small foci in the myocardium [2,3]. The higher occurrence of tachyarrhythmias after the operation suggested that ischemic myocardial injury existed in the present patients. This left room for a delayed IP protective effect.
The antiarrhythmic IP effect might share the same protective mechanism with that in the anti-necrosis IP mechanism, for example, the same triggers (adenosine, norepinephrine), activation of G-protein, and the opening of KATP channels [19,24]. However, the IP effect is not related to a reduction in either severity or duration of ischemia, suggesting that arrhythmic protection is a direct consequence of IP rather than an epiphenomenon of ischemic protection [20,22]. Some studies have shown that adenosine and the opening of KATP channels are not involved in the antiarrhythmic IP mechanism [19]. It is unlikely that the translocation of protein kinase C (PKC) to the membrane can explain the IP antiarrhythmic effect, since it is proarrhythmic rather than antiarrhythmic [21]. Endothelium-derived protective mediators such as bradykinin, prostacyclin, nitric oxide, and cyclic guanosine 3′,5′-mono-phosphate (cGMP), which do not seem to be involved in the anti-ischemic effect, are seen to be important in the antiarrhythmic effects of IP [19,21]. The role of an antiadrenergic and/or an increased vagal effect of preconditioning has been demonstrated [19,22]. Delayed antiarrhythmic IP effect involves the induction of cytoprotective heat shock proteins and other potentially protective enzymes such as nitric oxide synthase, and increased myocardial anti-oxidant status [19,21].
IP protection in reducing both ischemia and reperfusion-induced arrhythmia is highly dose-dependent [18,20,22]. IP involves release of multiple mediators and activation of multiple signal transduction pathways. These mediators act in concert to stimulate cell surface receptors to produce IP effects. Repeated cycles may lead to a cumulative increase in protection against arrhythmias [19,20]. An excess of cycles of IP may on the other hand, result in cumulative effects of ischemic damage and could be detrimental [19]. This was the reason why we chose to use two cycles of IP protocol in the present study. Our finding showed that the arrhythmic events during the IP procedure were not significantly higher than in the controls. Animal studies have also shown the incidences of arrhythmias during the effective IP protocol to be low [16]. This implies that a short ischemia period in IP protocol is sufficient for protection. Previously, we have reported that the same IP protocol might cause higher CTnI release during the IP period and result in better hemodynamic outcome [11]. Thus the IP protocol is effective as an additional myocardial protective method in three-vessel diseased CABG patients.
The surgical manipulation of the heart during the IP protocol and during the early reperfusion period may partly contribute to the high arrhythmic events during these two periods. We excluded the first 10 min after reperfusion because the sinus rate usually returned after this period. Previous studies suggested that antiarrhythmic IP phenomenon is a direct consequence of protective mechanism [20,22]. In the present study, we could not exclude the possibility that the reduced arrhythmic episodes might benefit from the better postoperative outcome of the IP patients, especially, relatively shorter mechanical ventilation period, earlier use of β-blocker and better left ventricular function as we presented previously [11,13]. Another limitation is the relatively small population investigated and the short postoperative period recorded. Postoperative VT might increase a risk of sudden cardiac death. Small samples could not derive a satisfactory statistical analysis of antiarrhythmic intervention and mortality. For ethical reasons, we limited recording to only 48 h postoperatively, in order to minimize patient inconvenience and discomfort. In the later recovery, the incidence of SVT might have been higher than during the first 2 postoperative days. We excluded patients with severe calcified ascending aorta; in clinical practice, the beneficial effects of IP might be considered to be potentially harmful for multiple aortic cross-clamping in such patients.
In summary, arrhythmias were common in three-vessel diseased patients before and after the operation. Two cycles of 2-min ischemia followed by 3-min reperfusion significantly suppressed the severity of VES, SVT, and VT. The protective effect during the period of 24–48 h after reperfusion indicated a delayed antiarrhythmic IP phenomenon in CABG surgery. The findings would imply that IP could provide an additional myocardial protective strategy in three-vessel diseased patients undergoing CABG.
The study was supported by the Research Foundation of Tampere University Hospital.
References
- anti-arrhythmia agents
- cardiac arrhythmia
- myocardium
- electrocardiogram
- ischemia
- angina, stable
- coronary artery bypass surgery
- coronary arteriosclerosis
- tachycardia, ventricular
- sudden cardiac death
- ischemic preconditioning
- supraventricular tachycardia
- ascending aorta
- reperfusion therapy
- physiologic reperfusion
- surgical procedures, operative