-
PDF
- Split View
-
Views
-
Cite
Cite
A. Dodge-Khatami, H.W.M. Niessen, A. Baidoshvili, T.M. van Gulik, M.G. Klein, L. Eijsman, B.A.J.M. de Mol, Topical vascular endothelial growth factor in rabbit tracheal surgery: comparative effect on healing using various reconstruction materials and intraluminal stents, European Journal of Cardio-Thoracic Surgery, Volume 23, Issue 1, January 2003, Pages 6–14, https://doi.org/10.1016/S1010-7940(02)00722-4
- Share Icon Share
Abstract
Objectives: The effect of topical vascular endothelial growth factor (VEGF) on post-surgical tracheal healing using various reconstruction materials was studied, with particular regard to prevention of granulation tissue or fibrosis. Methods: Twenty-four New Zealand White rabbits underwent survival surgery using autograft patches (n=6), xenopericardium patches (n=6), intraluminal Palmaz wire stents (n=6), and controls (n=6). Autograft and pericardial half-patches were soaked in topical VEGF (5 μg/ml over 30 min) and saline before reimplantation. Stents and controls received circumferential injections of VEGF and saline in the tracheal wall. At 1–4 months postoperatively, specimens of sacrificed animals were stained with anti-VEGF antibody, followed by morphological and immunohistochemical examination. Results: Rabbits with autografts and controls fared well until planned sacrifice. After xenopericardium repair, obstructive intraluminal granulation tissue led to early sacrifice in three rabbits. Stent insertion led to earlier death from airway obstruction in all six rabbits. Topical VEGF reduced granulation tissue after pericardial repair and fibrosis in all repairs except in stents. Remarkably, VEGF-pretreated half-patches and saline half-patches stained similarly high for VEGF, suggesting also local production of VEGF, probably in plasmacells, and in submucosal glands. Conclusions: Autograft repair induces the least granulation tissue and fibrosis, and the best healing pattern. Stents rapidly induced critical airway obstruction, unhindered by VEGF, leading to premature death. Tracheal pretreatment with topical VEGF reduces postoperative fibrosis after autograft and pericardial patch repairs, and reduces granulation tissue after xenopericardium repair. In time, VEGF is probably locally produced, although its potential role in tracheal healing remains to be established.
1 Introduction
To date, healing of the major airways after any type of insult, be it traumatic, chemical or surgical, has been marred by the formation of excessive granulation tissue at the site of epithelial disruption. Clinically, this presents as progressive or acute respiratory distress from various degrees of intraluminal obstruction, ranging from mild stenosis to complete obliteration of the airway.
Histologically, healing of the respiratory epithelium usually occurs through migration of adjacent secretory and squamous epithelial cells to cover the defect, followed by hyperplasia and stratification, metaplasia of the epithelium to pseudostratified columnar epithelium, and differentiation into ciliated respiratory epithelium [1,2]. Any mechanism disrupting this sequence of events will hinder appropriate inner coating of the airway lumen with functional specialized respiratory cells, and hence represents a matrix for granulation tissue, consisting of fibroblasts, inflammatory cells, and interstitial fluid [2].
Vascular endothelial growth factor (VEGF) is an endogenous protein, secreted mainly by macrophages and fibroblasts, whose local production is enhanced in the setting of ischemia, hypoxia, and anemia [3]. It is a potent angiogenic factor, increases vascular permeability, is involved in tissue healing, and has been found in tracheal granulation tissue in children [4,5]. Recent evidence using this same rabbit model revealed the enhancing effect of topical recombinant human VEGF on tracheal autograft healing, although this was performed with a concentration of VEGF (5 μg/ml), an intraoperative exposure time (15 min), and postoperative sacrifice interval (2 months) that were arbitrary [6]. Potential mechanisms favoring tissue healing at the time of surgical insult include VEGF-induced vasodilation, which creates a more favorable local oxygen/hemoglobin supply, and increases permeability towards reparatory inflammatory cells [6]. The angiogenesis induced by VEGF in a more intermediate time frame is a second presumed positive effect [6]. However, the potential for uncontrolled angiogenesis and increased tissue edema that may occur in time by exogenous VEGF currently needs more investigation, before its clinical application is judged safe.
This study set to establish a protocol for optimal penetration of topical VEGF, at safe concentrations and exposure intervals, with a pilot study using a rabbit model. Optimal penetration was defined as at least moderate staining of VEGF in the (sub)epithelial level, where microvessels are at their most dense, and where VEGF-induced angiogenesis exerts its maximal effect [6]. Following the pilot study, the in vivo characteristics of exogenous VEGF in the tracheal wall were studied in a rabbit survival model comparing various reconstruction materials and stents which are commonly used in clinical surgical practice.
Elaborating a safe long-term animal survival model may promote the use of topical VEGF in human trials. This may be achieved by establishing a VEGF treatment protocol which delivers the desired boost of blood nutrients and reparatory cells at the time of surgery, while demonstrating controlled tracheal wall angiogenesis and edema during the normal inflammatory and healing process afterwards. Through reduced anastomotic granulation tissue and fibrosis after pretreatment with topical VEGF, it is hoped to improve postoperative airway healing, and thereby reduce morbidity after tracheal surgery.
2 Materials and methods
The study was performed in two stages: an initial in vitro study in which an optimal exogenous VEGF concentration and exposure time were sought, and a second in vivo survival study which compared the effect of topical exogenous VEGF, at the concentration and exposure time determined by stage 1, on different reconstruction materials during tracheal surgery. The anesthetic and surgical protocol has been described elsewhere in detail by the first author, and was applied at both stages of the study [6]. The study protocol was reviewed and accepted by the Hospital Research Ethics Committee. All animals received humane care in compliance with the ‘Guide for the Care and Use of Laboratory Animals’ prepared by the Institute of Laboratory Animal Resources, and published by the National Academy Press, revised 1996.
2.1 Stage 1
Four New Zealand White rabbits (2.1–2.8 kg) underwent surgery for harvesting of tracheal specimens according to the study protocol. From each rabbit, 20 pieces of trachea were excised for a total of 80 pieces, measuring approximately 3×3 mm. These were soaked in solutions of either placebo saline or increasing concentrations of human recombinant VEGF (R&D Systems, Minneapolis, MN), arbitrarily at 2, 5, and 10 μg/ml. The duration of exposure to the topical treatment or placebo increased from 1, 5, 15, and 30 to 45 min, before being taken out of the solution for fixing and staining. As this stage was not a survival study, and the defects in the trachea were too long to be reconstructed, the animals were not awakened from anesthesia and sacrificed using intramuscular pentobarbital (100 mg/kg).
2.1.1 Processing of tissue specimens
The specimens were fixed in 4% formalin and subsequently embedded in paraffin. Paraffin-embedded tissue sections (4 μm) were mounted on microscope slides and were deparaffinized for 10 min in xylene at room temperature, and then rehydrated through descending concentrations of ethanol. Sections were then stained with hematoxylin–eosin.
2.1.2 Immunohistochemical detection of VEGF
For staining with VEGF, the sections were preincubated in citrate (pH 6) at 360 °C over 10 min. Thereafter, sections were treated with 0.3% H2O2 in methanol for 30 min to block endogenous peroxidase activity. Sections were then preincubated with normal rabbit serum (1:50 A/S, Glostrup, Denmark) for 10 min at room temperature, and incubated for 60 min with anti-human VEGF antibodies (1:50, total goat IgG, R&D Systems, Minneapolis, MN). After washing in phosphate-buffered saline (PBS), sections were incubated for 30 min with rabbit-anti-mouse biotin labeled antibody (1:500, Vector), and subsequently washed in PBS. After incubation with biotin labeled streptavidin-horseradish peroxidase (HRP) (1:200 Dako A/S) for 60 min at room temperature, HRP was visualized with 3,3-diamino-benzidine-tetrahydrochloride/H2O2 (Sigma, St. Louis, MO) for 3–5 min.
Two independent investigators (A.D.-K., H.W.M.N.) were blinded to the repair and treatment segments, each judging and scoring all slides for anatomical localization of specific antibody, as visualized by immunohistochemical staining. For the final scoring results, consensus was achieved by the two investigators.
2.2 Stage 2
In a survival model, 24 New Zealand White rabbits underwent surgery according to our protocol. At the end of the procedure, they were extubated and cared for until the predetermined sacrifice interval of 4 months. Surgical repair included two groups of six rabbits. In each group, surgery simulated anterior patch plasty repair of the trachea. The first group (n=6) underwent autograft repair (AUTO), consisting of excision of an anterior ellipse of tracheal wall, measuring four to six cartilage rings in length and one-third of the tracheal circumference. The harvested autograft was then cut in two halves, one soaked in exogenous VEGF, and the other in control placebo saline. Multiple interrupted 6-0 polydioxanone (PDS) sutures were circumferentially placed during this topical treatment interval. The two half-patches were then sutured together (VEGF-half superiorly) and into place to fill the tracheal defect. The second group (n=6) underwent pericardial patch repair (PERIC), using bovine pericardium (Shelhigh Incorporated No-React, Milburn, NJ). In the same fashion and size as the autograft group, this consisted of an anterior upper half-patch of VEGF-treated bovine pericardium, and a lower half-patch of saline placebo pericardial patch. Group 3 (n=6) underwent intraluminal stent placement (STENT), using balloon-expandable stainless steel wire Corinthean-Palmaz stents (Johnson & Johnson Interventional Systems, Warren, NJ), measuring 5×17 mm. After exposure of the trachea, a horizontal anterior incision was made into the trachea, two to three rings below the vocal cords. Under direct surgical vision, the undeployed stent with its sheath was introduced distally into the trachea, and deployed until firm attachment to the inner tracheal wall was evident. The stent was secured in place from the outside with two transfixing 6-0 PDS sutures at each stent extremity, to avoid stent migration. Half a milliliter of topical VEGF (5 μg/ml) was circumferentially injected into the tracheal wall at the level of the superior securing stitch, which was used as a marker for the subsequent histological studies. The lower end of the tracheal wall and indwelling stent was also circumferentially injected with 0.5 cc of saline. Finally, a control group (n=6) was used to represent no surgical or stent-related trauma of the inner lumen respiratory epithelium. These animals were induced with intramuscular acepromazine and ketamine-xylazine as per protocol, but were not intubated or anesthetized. With supplementary mask oxygen in a spontaneously breathing rabbit, surgical exposure of the trachea was obtained, and topical VEGF (5 μg/ml) and saline were circumferentially injected into the tracheal wall at the level of external marking sutures. The wound was closed and the animals awakened.
At a predetermined survival interval of 4 months, animals were sacrificed with intramuscular pentobarbital (100 mg/kg). The trachea were harvested from cricoid to carina, and were opened posteriorly in a longitudinal fashion, so as to leave the anterior tracheal patch repairs intact. The stents were initially cut through posteriorly, and carefully peeled away from the intraluminal tracheal surface, so as to leave the underlying tissue relatively undisturbed.
Fixation of tracheal specimens and VEGF staining was performed as described after stage 1. Microscoping evaluation was performed with regards to the intensity of the VEGF density in the epithelium, microvessel walls, plasmacells, and submucosal glands, using a semi-quantitative grading system from 0 to 4 (0, none; 1, trace; 2, weak; 3, moderate; 4, strong). Granulation tissue was scored from 0 to 2 (0, none; 1, (sub)mucosal; 2, intraluminal protrusion). At a magnification of 400×, the area of fibrosis was scored as a percentage of the entire surface visible within that high power field. Considering the length of the tissue cuts in relation to a point zero, being the interface between VEGF pretreated half-patches and saline half-patches, 4 mm thick zones were considered and labeled +1 and +2 as the distance from point 0 increased. Thus, ‘contamination’ from pretreatment of one half-patch to another (VEGF versus saline) would be minimal at the two extremity +2 zones. Initially, mean scores were determined for each zone and compared across the various animals and repairs. As there was no significant difference between any of the zones, mean values including zones 0, +1 and +2 with standard deviations were calculated and introduced in the statistical analysis, which used a paired Student's t-test.
3 Results
3.1 Stage 1
Tracheal specimens soaked in saline placebo did not stain for VEGF at any exposure time, suggesting that local production of VEGF does not occur after complete devascularization after such a short interval before fixation in formalin. At the lower concentration of 2 μg/ml, no detectable staining was noted until 30 min, and then only lightly in the superficial epithelial layer. This was comparable to the superficial and light staining at 10 μg/ml which had already occurred after 5 min of treatment.
Deeper and more intense staining in the (sub)epithelial layer was similarly achieved with 5 μg/ml at 30 min of exposure or longer, and with 10 μg/ml at 15 min or longer.
According to these results, we decided to expose the two surgical repair groups (AUTO and PERIC) to a topical treatment with exogenous VEGF at concentrations of 5 μg/ml during an exposure interval of 30 min. Exposure intervals of 30 min were not rational in the STENT group, as this inert material was not expected to soak up any VEGF. Also, in the control group, a prolonged injection of VEGF lasting 30 min was not possible, and hence the exposure to VEGF was of the pulse type. These two groups constitute a difference with regards to study design relating to the stage 1 in vitro study, even if the same concentration of 5 μg/ml was used.
3.2 Stage 2
3.2.1 Clinical aspect
All six rabbits in the AUTO group fared well until the scheduled sacrificed date at 4 months postoperatively, as did five rabbits in the VEGF control group, and three rabbits in the PERIC group. One rabbit in the VEGF injection control group died. As this operation entailed no surgical trauma to the airway, the animal's demise was probably related to excessive anesthesia and hypoxia, as it never woke up. Progressive respiratory distress resulted in premature sacrifice in three rabbits from the PERIC group at 3 months. This was even more striking in the STENT group. Amongst the six rabbits who had received intraluminal stents, three expired at 1 month, and another three expired at 2 months.
3.2.2 Macroscopic aspect
In concordance with the clinical outcomes, both in the AUTO group and in controls, the inner aspect of the tracheal lumen was smooth and widely patent. Interestingly, there was no visible difference between the upper half of the patch, which had been pretreated with VEGF, and the lower control half-patch. In the PERIC group, a thin protruding ridge into the tracheal lumen was present along the entire length of the anastomotic line, and again, equally at both pretreated and control half-patch suture lines. The lumen of the six rabbits after stent placement was grossly stenosed, with granulation tissue bulging through the stent meshwork along its entire inner surface.
3.2.3 Microscopic aspect
In contrast with the morphological characteristics described above, no statistical difference in the intensity of immunohistochemical staining was found between the VEGF pretreated zones and the saline zones, although VEGF staining was consistently more intense in the VEGF-treated zones. Accordingly, for the grading system and statistical analysis, we decided to combine the results with regards to the VEGF density of zones 0, +1 or +2 on both the VEGF and saline extremities. These are presented as a mean value of the VEGF and saline results.
With regards to fibrosis, however, the saline zones systematically contained a higher percentage compared to the VEGF-pretreated zones, suggesting an inhibitory effect of topical VEGF on the inflammatory response (Table 1) . This was statistically significant when considering fibrosis after pericardial repair in non-treated zones as compared with autograft repair or controls (P<0.05 and P<0.04, respectively). Also, granulation tissue was less in the VEGF-pretreated zones after pericardial repair and stent insertion, as compared to the saline zones, although this did not reach statistical significance (Table 1).
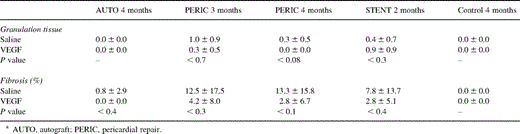
Amongst each group where a difference in sacrifice interval occurred (PERIC and STENT), mean values were calculated confounding the different time end-points. First and foremost, at all time intervals and for each type of repair material utilized, VEGF was present in granulation tissue, in the epithelium (Fig. 1a,b) , in blood vessels, plasmacells, and submucosal glands, in both VEGF-treated and non-treated saline zones.
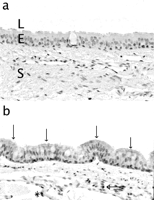
(a,b) Immunohistochemical detection of VEGF at 4 months postoperatively. Trachea from a control rabbit (a) and after autograft repair (b). Original magnification 400×. Open arrows indicate strong positivity of the epithelium for VEGF, and a closed arrow indicates strong VEGF positivity in plasmacells. * indicates trace positivity of the endothelium for VEGF. L, lumen; E, epithelium; S, subepithelium.
In the AUTO group, no granulation tissue was found at the 4 month sacrifice date (0.0±0.0), and fibrosis was absent in the VEGF-treated zones (0.0±0.0%) (Fig. 2a) . The intensity of epithelial VEGF was graded at 2.7±1.0, while vascular wall density was 0.4±0.6, plasmacell density 1.9±0.9, and glandular density 1.8±1.2.
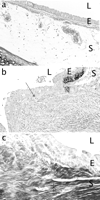
(a–c) Trachea from a rabbit 4 months after autograft repair (a) with a wide open lumen (L), no granulation tissue and minimal fibrosis in the epithelium (E) and subepithelium (S) (250×), as compared to that of a rabbit 2 months after stent insertion with granulation tissue (closed arrow) protruding into the lumen (b), and subepithelial fibrosis (*) in the saline zone (c) (400×). Elastica von Gieson staining.
In the control group, no granulation tissue or fibrosis was observed at 4 months (0.0±0.0). Epithelial VEGF intensity was a low 0.4±0.6, and measured 0.2±0.4 in the vascular wall, 0.2±0.4 in plasmacells, and 0.1±0.2 in the glands.
In the PERIC group, epithelial VEGF was 2.2±0.5, VEGF vascular wall density 0.4±0.7, plasmacell VEGF 1.9±0.7, and glandular VEGF 1.7±0.5.
In the STENT group, there were large amounts of granulation tissue, which protruded through the stent meshwork, partially reducing the airway lumen (Fig. 2b). Fibrosis reached a high 40% in some specimens but averaged 7.8±13.7% over time, and probably contributed to the early demise of all six rabbits from airway obstruction (Fig. 2c). Epithelial VEGF was 1.1±0.2, glandular VEGF 1.0±0.3, vascular wall VEGF 0.3±0.4, and plasmacell VEGF 0.8±0.6.
Comparative results of immunohistochemical staining for VEGF between the various reconstruction materials are shown in Fig. 3 .
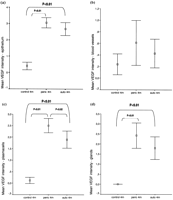
(a–d) Comparative densities of VEGF at 4 months in the epithelium, blood vessels, plasmacells, and glands. Peric, pericardial repair; auto, autograft repair; m, months.
4 Discussion
Minimizing surgical trauma, avoiding anastomotic tension, and preventing infection have invariably been promoted in an attempt to create a favorable environment for postoperative anastomotic healing of the major airway. This applies to the repairs of congenital tracheal stenosis, post-intubation injury, inhalation stricture, stenosis in the setting of neoplastic disease, or after lung transplantation [4,6–8]. Unfortunately, and in spite of all current efforts, granulation tissue formation, fibrosis, and resultant stenosis of the airway remain unpredictable, and still account for unfavorable outcomes after what appears to be successful surgical repair [8–11].
Not only does recurrent granulation tissue frequently require repeat bronchoscopy for therapeutic dilation or debridement, it progresses to fibrosis and fixed narrowing of the airway, which may lead to recurrent respiratory distress, the need for intubation and mechanical ventilation, and even repeat surgical intervention [7–10]. Debridement and/or reoperation for recurrent stenosis from obstructive granulation tissue has been reported after all types of surgical repair. After anterior pericardial patch tracheoplasty, Bando et al. reported only minor granulation tissue in two patients from a series of 12 (16.7%), who did not require reoperation, but simple bronchoscopic debridement [9]. Backer et al. reoperated on 25% of their patients who had initially undergone pericardial patch tracheoplasty, at a mean interval of 4.7±1.9 months after the first operation [11]. Granulation tissue has been particularly troublesome after cartilage grafting [7,10], especially if the cartilage graft represents more than 30% of the circumference of the airway [10]. Finally, granulation tissue formation is one of the major concerns after intraluminal stent insertion, occurring in 50–100% of cases [12,13]. The use of newer intraluminal expandable metallic covered stents has given initial promising short-term results, as the covered layer theoretically prevents protrusion of ingrowing granulation tissue through the stent meshwork [14]. In contrast, after tracheal autograft repair, the experienced group from Children's Memorial Hospital in Chicago report only one reintervention for insertion of a Palmaz intraluminal stent in a series of 15 patients (6.7%), which was for recurrent stenosis of the pericardial patch portion of a composite autograft/pericardium repair [8]. These excellent clinical results and relative freedom from recurrent granulation tissue after autograft repair concord with our current results, as well as with the first author's previous experience using the same rabbit model of autograft reconstruction [6].
Exogenous topical VEGF is a proposed treatment modality, attempting favorably to influence tracheal anastomotic healing. At the time of surgical insult, an improved balance in local supply and demand for oxygen/hemoglobin may be achieved, through a one-time boost of VEGF-induced release of endothelial nitric oxide and resultant vasodilation [15]. Postoperatively in a more timely fashion, through its local angiogenic effect and enhancement of vascular permeability with the afflux of reparative inflammatory cells, the healing process may further be promoted. In a previous study by the first author [6] using the same surgical model of tracheal autograft anterior patch plasty in 16 VEGF-treated and 16 control rabbits, topical VEGF (5 μg/ml during 15 min) accelerated autograft revascularization, reduced submucosal fibrosis and inflammation, and preserved normal tracheal architecture, as compared to controls, at a sacrifice interval of 2 months postoperatively. In the current study, after a 4 month sacrifice interval, minimal concentration (5 μg/ml) and exposure time (30 min) to topical VEGF were found to enhance healing in the AUTO group, and are judged to be safe in rabbits. Indeed, the rabbits were clinically asymptomatic and tracheal wall angiogenesis and edema were controlled. Longer exposure or higher doses are unnecessary, expensive, and may have unwanted side effects. By elaborating a safe animal model with long-term survival, it is hoped to promote the use of topical VEGF in human trials.
Through reduced anastomotic granulation and fibrous tissue after pretreatment with topical VEGF, postoperative airway healing is enhanced and morbidity reduced. Fibrous tissue at the repair site diminished with all repair materials except after stent insertion. We found autograft repair to have the best healing characteristics and clinical course, as confirmed histologically by the absence of granulation tissue and minimal fibrosis. This may be due to the effect of topical VEGF, but also to the intrinsic advantage in using autologous tracheal tissue for reconstruction. As mentioned previously, excellent clinical results using the autograft repair without the adjunct of VEGF are reported, making this the procedure of choice for some [8]. Pericardial patch repair gave clinically satisfactory early results, yet all rabbits did not reach the predetermined sacrifice date, owing to increasing respiratory distress. Histologically, this was illustrated by a relatively higher degree of intraluminal fibrosis and granulation tissue, as compared to autograft repair. Xenopericardium was used in our experiment, and issues pertaining to foreign body rejection and immunology may have played a role. A direct comparison between our findings and the use of autologous pericardium for tracheal reconstruction, as is performed in many centers with good results [9,11], is therefore not totally straightforward. Intraluminal stents performed the worst in our study, both clinically with a 100% precocious mortality from respiratory distress, and histologically with maximal obstructing intraluminal granulation tissue and fibrosis. This concurs with the mediocre results in the literature, where stents are mostly used for either inoperable lesions [14], difficult reoperations [12], or as bail out procedures [13,16]. Although the mean degree of fibrosis observed was only slightly higher in stents as compared to xenopericardial repair, the sacrifice interval was much shorter for rabbits with indwelling stents (1–2 months versus 3–4 months). One could speculate that a longer sacrifice interval in the STENT group would have allowed for more time to develop fibrosis, had the rabbits survived the progressive airway obstruction.
Interestingly, in the same animal, VEGF-pretreated zones and control saline zones did not significantly differ in VEGF staining at the (sub)epithelial level, although there was a trend towards a higher density in the VEGF-pretreated zones. This may suggest either one of two things or both: (1) that the observed VEGF density is actually additional locally secreted VEGF in the setting of postoperative inflammation and healing, rather than solely the pretreatment of VEGF at the time of surgery; or (2) that the topical VEGF treatment was ‘washed over’ to the saline control zone, as a result of the to-and-fro motion of respiration and carried air-VEGF particles. The first phenomenon is suggested by the high concentration of VEGF found in plasmacells. High plasmacell VEGF concentrations have been found in various chronic inflammatory disorders, such as human periodontal disease [17], nasal, uterine, and gastric polyps, human B cell leukemia and plasmacytoma [18]. In these diseases, it is speculated that plasmacell production of VEGF plays an important role in the development of edema [18], either as a pathological response, or as a part of a healing process [17]. Inversely, diseases such as diabetes, smoking, or simply older age, which are associated with impaired wound healing, have been associated with low tissue VEGF levels [17,19]. Local production of VEGF, mainly by plasmacells, but also by submucosal gland cells and microvascular endothelial cells, rather than topical pretreatment with exogenous VEGF alone, may play an important role in postoperative tracheal healing, although this remains speculative. The time interval at which this occurs remains unknown, and further investigation may elucidate this. The significance of increasing levels of epithelial glandular VEGF with time is also unknown, and only speculation may be made as to its role in promoting local healing.
In conclusion, the postoperative healing of rabbit trachea was favorably influenced by exogenous topical VEGF, given as a pulse treatment at the time of surgery, by favorably influencing the local tissue supply-and-demand balance for nutrients and reparatory cells. After surgical repair, ongoing intrinsic local production of VEGF, probably by plasmacells, occurred in a more timely fashion, although its relative role in tracheal healing is yet to be determined. Autograft tracheal reconstruction gave the best clinical and histological healing results, when compared to xenopericardium reconstruction or insertion of intraluminal stents. This was seen even without the adjunct of exogenous topical VEGF, which nonetheless further enhanced the quality of local repair, as was histologically quantified during our study. The intense early inflammation and fibrosis induced by stents were unhindered by VEGF, and led to critical airway obstruction, respiratory insufficiency and ultimately to early death.
4.1 Study limitations
The small number of animals reduces statistical power and limits the value of the present study. A limitation pertaining to the study design lies in the difference of exposure to topical VEGF between the AUTO+PERIC groups and the STENT+control groups. Owing to obvious practical reasons relating to syringe injections, inert stents or the controls were not exposed to 30 min of VEGF, as preconized in the in vitro stage 1 study. Also, injecting topical VEGF along the entire tracheal length in animals with stents or controls was judged to potentially enhance any ‘washover effect’ with regards to the saline and VEGF zones. The circumferential injections at both tracheal extremities gave local information with regards to tissue reaction towards saline versus VEGF.
Dr Niessen is a recipient of the Dr E. Dekker program of the Netherlands Heart Foundation (D99025).
Mr B. Keogh (Birmingham,UK): Why do you think that the injection of saline produced a similar response to the injection of VEGF and do you think there are any parallels with the fact that in the heart, for example, if you simply do multiple injections you can provoke the production of VEGF to the same extent as if you inject the genes?
Dr Dodge-Khatami: I think one part of your question could be answered simply by the study design. The fact that we used half patches with one being treated and one not gives us a problem. There are two speculations to interpret this.
Number one, there is a to-and-fro motion of air, which could potentially carry particles from the VEGF-treated half patch towards the saline one. Nonetheless, although it wasn't significant, there was a difference at each point in each type of repair between the saline zones and the VEGF zones, in favor of the VEGF zones. Therefore, we do have reason to think that it is the VEGF pretreatment that showed the improved effect of the healing as opposed to the saline.
Secondly, this correlates with what is found in the literature concerning other inflammatory diseases and the presence of VEGF in plasmacells. There are other chronic inflammatory diseases, such as periodontal disease, gastric and nasal polyps, plasmocytomas, and some leukemias where there has been an increased VEGF production seen in the plasmacells. Some speculate that it is a normal reaction during healing, and others think it is an abnormal response with edema. The fact that we also found even in the nontreated zones, in other words, in the saline zones, a stronger presence of VEGF in the plasmacells makes us think that there is local production on top of the pretreatment. But that is very difficult to actually prove, and at what time it occurs during the healing process is also fairly unknown. I cannot make a parallel with the heart.