-
PDF
- Split View
-
Views
-
Cite
Cite
David T. Lai, Tomasz A. Timek, Frederick A. Tibayan, G. Randall Green, George T. Daughters, David Liang, Neil B. Ingels, D. Craig Miller, The effects of mitral annuloplasty rings on mitral valve complex 3-D geometry during acute left ventricular ischemia, European Journal of Cardio-Thoracic Surgery, Volume 22, Issue 5, November 2002, Pages 808–816, https://doi.org/10.1016/S1010-7940(02)00530-4
- Share Icon Share
Abstract
Objective: Annuloplasty rings are used to treat ischemic mitral regurgitation (IMR), but their exact effects on 3-D geometry of the overall mitral valve complex during acute left ventricular (LV) ischemia remain unknown. Methods: Radiopaque markers were sutured to the mitral leaflet edges, annulus, papillary muscle tips, and ventricle in three groups of sheep. One group served as control (n=5), and the others underwent Duran (n=6) or Physio (n=5) ring annuloplasty. One week later, 3-D marker coordinates at end-systole were obtained before and during balloon occlusion of the circumflex artery. Results: In all control animals, acute LV ischemia was associated with: (i) septal-lateral separation of the leaflet edges, which was predicted by lateral displacement of the lateral annulus during septal-lateral mitral annular dilatation; (ii) apical restriction of the posterior leaflet edge, which was predicted by displacement of the lateral annulus away from the non-ischemic anterior papillary muscle; (iii) displacement of the posterior papillary muscle, which was not predictive of either septal-lateral leaflet separation or leaflet restriction; and (iv) mitral regurgitation. In the Duran group during ischemia, the posterior leaflet edge shifted posteriorly due to posterior movement of the lateral annulus, but no IMR occurred. In the Physio group during ischemia, neither the posterior leaflet edge nor the lateral annulus changed positions, and there was no IMR. In both the Duran and Physio groups, displacement of the posterior papillary muscle did not lead to IMR. Conclusions: Either annuloplasty ring prevented the perturbations of mitral leaflet and annular – but not papillary muscle tip – 3-D geometry during acute LV ischemia. By fixing the septal-lateral annular dimension and preventing lateral displacement of the lateral annulus, annuloplasty rings prevented systolic septal-lateral leaflet separation and posterior leaflet restriction, and no acute IMR occurred. The flexible ring allowed posterior displacement of the posterior leaflet edge and the lateral annulus, which was not observed with a semi-rigid ring.
1 Introduction
Mitral annuloplasty does not consistently correct chronic ischemic mitral regurgitation (IMR) in patients [1], but we do not know much about why annuloplasty rings sometimes fail to work [2]. In contrast, ring annuloplasty uniformly prevents IMR [3–5] in ovine models of acute left ventricular (LV) ischemia. This discordance between clinical and experimental results is not well understood. Further mechanistic insights into the means by which ring annuloplasty preserves mitral valve competence during acute LV ischemia may spawn new surgical approaches in the treatment of IMR. Prior experimental studies of the effects of ring annuloplasty on the mitral valve complex, however, were obfuscated by internal coordinate reference systems not oriented with respect to a stable mitral annular plane [3–5]. This limitation hindered interpretation of high temporal and spatial resolution geometric data derived using radiopaque marker technology [6]. Further, these earlier experiments [3–5] focused on scalar measurements of mitral valve geometry; three-dimensional (3-D) vector data of the entire mitral valve complex, i.e. the magnitude and direction of mitral annular, leaflet and papillary muscle tip displacements in 3-D space were not defined nor thoroughly integrated. To this end, we used an internal coordinate system based on the mitral annular plane to analyze 3-D geometric data of the overall mitral valve complex [3–5] in an ovine model of acute LV ischemia with and without annuloplasty rings.
2 Methods
2.1 Surgical preparation
Three groups of adult castrated male sheep (n=16) comprising a control group without annuloplasty rings (n=5) and two ring groups of 11 sheep that were randomly assigned to undergo either Duran (Medtronic Heart Valve Division, Minneapolis, MN) flexible ring annuloplasty (n=6) or Carpentier-Edwards Physio (Baxter Cardiovascular Division, Santa Ana, CA) semi-rigid ring annuloplasty (n=5). Two sheep missing an anterior leaflet marker were excluded from the present control group, which accounts for the smaller number of control animals in the present reanalysis compared to our prior reports [3–5]. Details have been previously reported [3–5,7] and are only briefly reiterated here. Six subepicardial and two septal miniature tantalum markers (inner diameter 0.8 mm, outer diameter 1.3 mm, length 1.5–3.0 mm) were inserted along four equally spaced LV longitudinal meridians. After establishing cardiopulmonary bypass, eight markers were sutured around the mitral annulus (#1–8, Fig. 1) ; note that what we defined as the anatomic ‘lateral’ mitral annulus is that called clinically the ‘posterior’ annulus. Markers were placed at the LV apex, and tips of the anterior (APM) and posterior papillary muscles (PPM). A coapting pair of markers was sutured to the ventricular surface of the midline anterior (AMID) and posterior (PMID) mitral leaflet edges (Fig. 1). The animals in the Duran group received 31- (n=5) and 29-mm (n=1) rings and those in the Physio group a 28-mm ring, sized using standard clinical criteria (the area of the anterior mitral leaflet) [3–5].
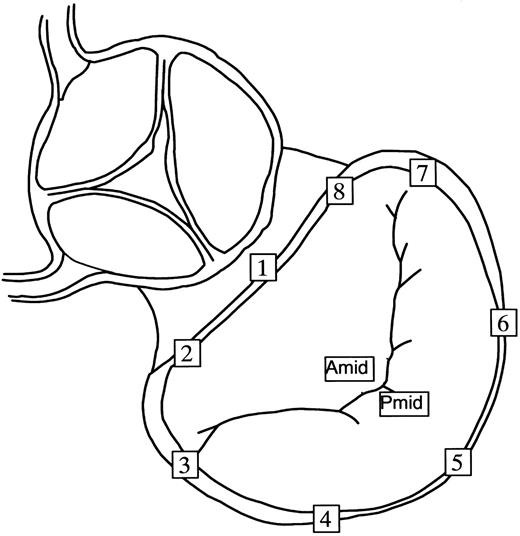
Radiopaque marker array used for the mitral annulus and leaflets. Markers were sutured to the mid-septal annulus (#1), left fibrous trigone (#2), anterior commissure (#3), left lateral annulus (#4), mid-lateral annulus (#5), right lateral annulus (#6), posterior commissure (#7) and right fibrous trigone (#8). What we defined here as the anatomic lateral mitral annulus is clinically termed the ‘posterior’ annulus. Markers were sutured to the AMID and PMID.
2.2 Experimental protocol
Following an 8±2 (mean±1 SD) day recovery period, each animal was taken to the cardiac catheterization laboratory, premedicated with ketamine, intubated and mechanically ventilated. A micromanometer-tipped catheter (Millar MPC-500, Millar Instruments, Houston, TX) was placed in the descending thoracic aorta to measure aortic pressure. With the animal in the right lateral decubitus position, simultaneous biplane videofluoroscopic and hemodynamic data were acquired under steady-state conditions and over a range of LV volumes using vena caval occluders for abrupt preload reduction. Control data were obtained before ischemia. Acute posterolateral LV ischemia was created by balloon occlusion of the left circumflex coronary artery distal to the first obtuse marginal artery [3–5]. Following 2–3 min of occlusion, ischemic data were acquired and the presence or absence of acute IMR was ascertained using transthoracic color Doppler echocardiography. Severity of IMR was graded as 0–4 plus.
All animals received humane care in compliance with the Principals of Laboratory Animal Care formulated by the National Society for Medical Research and the Guide for Care and Use of Laboratory Animals prepared by the National Academy of Sciences and published by the National Institutes of Health (DHEW [NIH] Publication 85-23, revised 1985). This study was approved by the Stanford Medical Center Laboratory Research Animal Review Committee and conducted according to Stanford University policy.
2.3 Data acquisition and reduction
A Philips Optimus 2000 biplane Lateral ARC 2/poly DIAGNOST C2 system (Philips Medical Systems, North America Company, Irvine, CA) was used to obtain videofluoroscopic marker data at 60 Hz and 9-inch mode of image magnification [3–5]. Two-dimensional images from each of the two X-ray views (45° right anterior oblique and 45° left anterior oblique) were digitized and merged to yield 3-D coordinates for each radiopaque marker every 16.7 ms using custom-designed software [6]. The analog LV pressure and electrocardiogram voltage signals were digitized and recorded in real-time on the video images during data acquisition.
2.4 Data analysis
Data from two consecutive steady-state beats during control and acute ischemia were averaged. End-systole was defined as the videofluoroscopic frame immediately preceding the peak negative rate of LV pressure change (−dP/dtmax), and end-diastole was defined as the videofluoroscopic frame containing the peak of the R-wave on the electrocardiogram. All dimensional data reported here were measured at end systole.
2.4.1 LV volume
Instantaneous LV volume was estimated every 16.7 ms from the epicardial LV markers using a space filling multiple tetrahedral volume method, as previously described [8]. Although epicardial LV volume calculated in this manner overestimates the true chamber LV volume because it includes myocardial wall volume, the change in epicardial LV volume is an accurate measurement of the change in LV chamber volume [8].
2.4.2 Internal coordinate reference system
For each frame, a mitral annular plane was defined by least-squares fit to the 3-D coordinates of seven of the eight mitral annular markers (#2–8, Fig. 1), omitting the mid-septal annular marker #1 (Fig. 1) which, as the ‘saddle horn’, does not lie on the mitral annular plane [7]. In each frame, a plane parallel to the mitral annular plane was defined containing AMID (Figs. 1 and 2) . As shown in Fig. 2, AMID was defined as the origin of a Cartesian coordinate system with the x-axis located in the plane oriented by the projection of marker #1 onto the plane (assigning a positive direction towards the lateral annulus); y-axis located in the plane, perpendicular to the x-axis (positive direction towards the posterior commissure); and z-axis perpendicular to the plane and positive towards the left atrium.
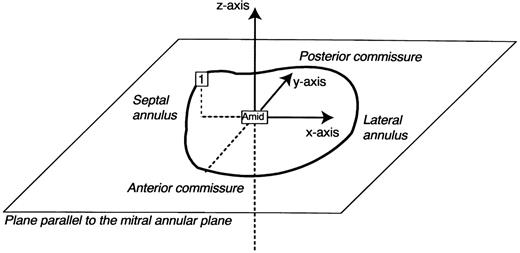
The internal Cartesian coordinate system employed in this reanalysis (see text).
2.4.3 Leaflet, annular and papillary muscle tip geometry
Using this coordinate system, the x, y and z coordinates of PMID, lateral annular markers (#4–6), APM and PPM markers were measured with respect to AMID at end-systole before and during LV ischemia. Displacement was measured in the septal-lateral direction (along the x-axis away or towards the lateral annulus, respectively), anterior-posterior direction (along the y-axis away or towards the posterior commissure), and apical-atrial direction (along the z-axis away or towards the left atrium).
2.4.4 Annular-papillary distances
The 3-D distance between the papillary tips (APM and PPM) and the lateral annular markers (#4–6) were measured at end-systole before and during LV ischemia.
2.5 Statistical analysis
All results are reported as mean±1 SD. The geometric variables measured before and during ischemia at end-systole were compared using a two-tailed Student's t-test for paired observations. The 3-D geometry of the mitral leaflets is ultimately governed by the 3-D geometry of the structures attached to the leaflets, i.e. mitral annulus and papillary muscle tips. Thus, any change in the 3-D geometry of the mitral annulus and papillary muscle tips during acute LV ischemia in the control group may produce changes in leaflet geometry that could lead to IMR. Conversely, ring annuloplasty may prevent changes in 3-D geometry of the mitral annulus and papillary muscle tips and consequently 3-D leaflet geometry associated with acute LV ischemia and thus prevent IMR. In order to determine those changes in annular and papillary muscle tip geometry most closely associated with specific changes in observed leaflet geometry, we used a multivariable statistical model with leaflet geometry change as the dependent variable and changes in annular and papillary muscle tip geometry the independent variables. A stepwise linear regression model (SPSS for Windows, Release 10.0.0, SPSS Inc., Chicago, IL) was used to identify predictors of leaflet geometry change, i.e. change in 3-D leaflet geometry. The aim of the multivariable analysis was to determine which component of change in annular and papillary muscle tip geometry were most important with respect to a specific change in leaflet geometry. Such statistical analysis sheds light on the geometric mechanisms underlying changes in leaflet geometry and consequently IMR, and also the means by which ring annuloplasty may prevent IMR.
3 Results
Animal weight was not significantly different among the 3 groups (control 61±10 kg, Duran 69±8 kg, and Physio 71±5 kg).
3.1 Mitral valve competence
Mitral regurgitation (MR) was not detected at baseline in the control group; after induction of ischemia, two control animals developed 2 plus IMR, two control animals had 3 plus IMR and one animal had 4 plus IMR. One plus IMR was noted in one Duran and one Physio animal before ischemia, but the severity of MR did not change after circumflex occlusion. The remaining ring sheep did not have MR either during baseline or ischemic conditions.
3.2 Hemodynamics
The hemodynamic data for all animals in each of the three groups before and during ischemia are summarized in Table 1 .
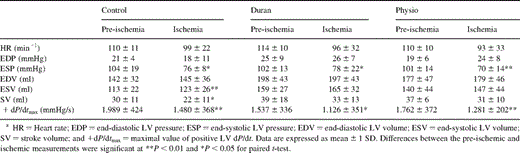
Hemodynamics during data acquisition before (pre-ischemia) and during ischemic conditions (ischemia) due to circumflex coronary artery occlusion
3.3 Interleaflet geometry
Table 2 summarizes displacements of the posterior leaflet during acute LV ischemia at end-systole. Figs. 3A–C graphically depict statistically significant and possibly significant posterior leaflet displacements. In the control group (Fig. 3A) during ischemia, PMIDx-coordinate was displaced towards the lateral annulus and LV apex. In the Duran group (Fig. 3B), PMIDy-coordinate was displaced towards the posterior commissure. In the Physio group (Fig. 3C), PMID was not displaced.
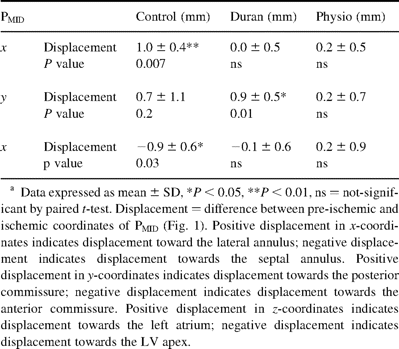
Three-dimensional coordinates at end-systole of the midline posterior mitral leaflet edge marker, PMID, with respect to the midline anterior mitral leaflet edge before and during acute left ventricular ischemia
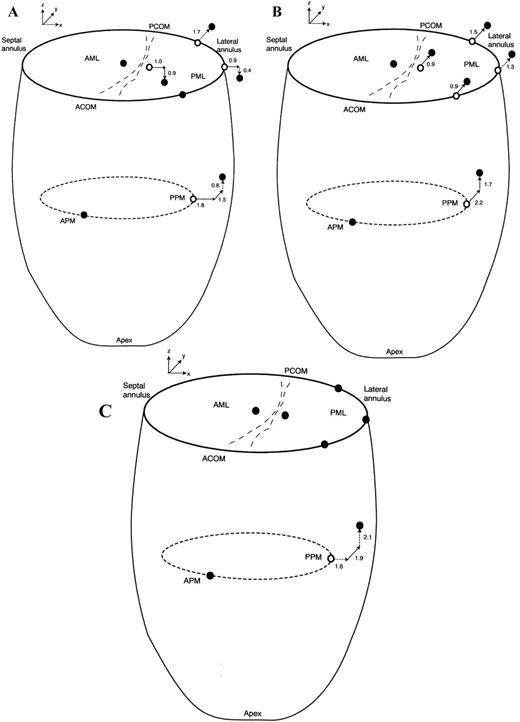
End-systolic 3-D marker positions before (open circles) and during acute left ventricular ischemia (filled circles) of the AML, PML, mitral annulus (oriented by the labels ‘SEPTAL ANNULUS’, ‘ACOM’ for anterior commissure, ‘PCOM’ for posterior commissure, and ‘LATERAL ANNULUS’), APM tip and PPM tip in the control (A); Duran (B); and Physio (C) groups. Statistically significant marker x-, y-, and z-displacements (mm) during LV ischemia relative to pre-ischemic values are depicted by bold arrows and displacements of possible statistical significance by the dashed arrows.
3.4 Lateral (or ‘posterior’) annular geometry
Displacements of the lateral (or clinically termed ‘posterior’) mitral annulus at end-systole with acute LV ischemia are tabulated in Table 3 ; Figs. 3A–C depict statistically significant and possibly significant (0.05<P<0.1) changes with ischemia. In control animals (Fig. 3A), the mid-lateral annulus was displaced laterally; posterior displacement of the right lateral annulus was only possibly statistically significant (P=0.10). In the Duran ring animals (Fig. 3B), the right, mid- and left lateral annular loci were all displaced posteriorly. In Physio ring animals (Fig. 3C), there was no significant displacement of any site on the lateral annulus.
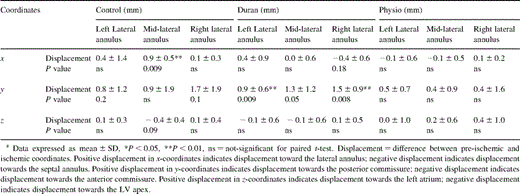
Three-dimensional coordinates at end-systole of the lateral (clinically termed the ‘posterior’) mitral annulus with respect to midline anterior mitral leaflet edge before and during acute left ventricular ischemia
3.5 Papillary muscle tips
Table 4 summarizes the displacements of the papillary muscle tips at end-systole during acute LV ischemia, and Figs. 3A–C depict the significant and possibly statistically significant changes. The position of the APM did not change in all groups. In control hearts (Fig. 3A), the PPM was displaced laterally and posteriorly. In animals with a Duran ring (Fig. 3B), the PPM was displaced posteriorly and towards the left atrium. In the Physio ring animals (Fig. 3C), the PPM was displaced posteriorly.
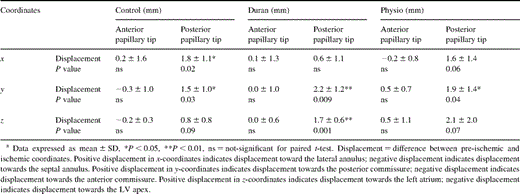
Three-dimensional coordinates at end-systole of the papillary muscle tips with respect to midline anterior mitral leaflet edge before and during acute left ventricular ischemia
3.6 Annular-papillary distance
Table 5 lists the changes in the distance between the papillary muscle tips and the lateral (or ‘posterior’) mitral annulus during acute LV ischemia. In the control group (Fig. 3A), the distance between the APM tip and right lateral annulus was 1.5±0.6 mm greater (P=0.004), and that between the APM tip and mid-lateral annulus was 0.6±0.6 mm greater (P=0.07). The PPM tip was 1.0±0.7 mm closer to the mid-lateral annulus (P=0.03). In the Duran ring group (Fig. 3B), the right lateral annulus was 1.0±0.7 mm further away from the APM tip (P=0.02) and 1.1±0.4 mm closer to the PPM tip (P=0.001). In the Physio ring group (Fig. 3C), acute ischemia produced no significant change in any of these annular-papillary distances.
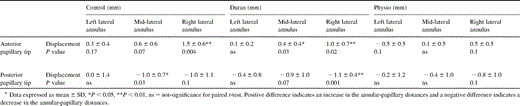
Papillary-annular distances (‘tethering’ geometry) in 3-D space at end-systole before and during acute left ventricular ischemia
3.7 Predictors of leaflet displacement
During acute IMR in control hearts (Fig. 3A), linear regression analysis revealed that the 0.9 mm lateral displacement of the mid-lateral annulus predicted lateral displacement of the PMID leaflet locus by 1.0 mm (R2=0.7, SEE=0.7 mm, P=0.001). In sheep with Duran rings (Fig. 3B), the PMID was displaced 0.9 mm posteriorly as predicted by a posterior displacement of the right lateral annulus (R2=0.6, SEE=0.5 mm, P=0.002); finally, for the Physio rings (Fig. 3C), the absence in change in PMID position corresponded to a lack of displacement of the right lateral annulus (R2=0.7, SEE=0.4 mm, P=0.001) during acute LV ischemia. In control hearts (Fig. 3A), movement of the right lateral annulus away from the non-ischemic APM by 1.5 mm predicted restriction (displacement towards the LV apex) of the PMID leaflet site (R2=0.9, SEE=0.4 mm, P=0.005). In the Duran and Physio groups, no satisfactory linear regression model could be constructed for PMID motion perpendicular to the annular plane as the displacements in the z-axis were tightly distributed about zero.
4 Discussion
4.1 Leaflet geometry
Echocardiographic [9–18] and prior marker studies [4] of IMR have provided uni-dimensional measurements of perpendicular leaflet distance from the mitral annular plane, cross-sectional area between the leaflets and mitral annular plane, or scalar distances between mitral leaflet edges [3], but have not characterized the overall 3-D geometry of mitral leaflet malcoaptation during ischemia in terms of the magnitude and direction of leaflet displacement. To characterize such, we used an internal coordinate system centered at the anterior mitral leaflet edge and orientated by the mitral annular plane to measure displacements of the posterior leaflet, lateral annulus and papillary muscles relative to the anterior leaflet at end-systole. In the control group during acute LV ischemia, this 3-D analysis demonstrated: (1) septal-lateral separation of the mitral leaflet edges with a 1.0 mm lateral displacement of PMID away from AMID; and (2) PMID apical restriction by 0.9 mm, which corresponds to Carpentier type IIIb restricted systolic leaflet motion [19]. These leaflet perturbations were associated with IMR. Either type of annuloplasty ring prevented septal-lateral leaflet separation, PMID restriction, and acute IMR. A flexible (Duran) ring allowed interleaflet ‘shearing’ characterized by displacement of PMID relative to AMID by 0.9 mm towards the posterior commissure, which was not observed in the Physio ring animals; however, this interleaflet shearing did not have a detrimental effect on valvular competence.
4.2 Predictors of leaflet displacement
Stepwise linear regression showed that lateral displacement of the mid-lateral annulus pulled the posterior leaflet laterally, and hindered coaptation by drawing the anterior and posterior mitral leaflets apart in directions parallel to the annular plane in control animals (Fig. 3A). Posterior papillary muscle tip displacement in directions parallel to the x-y plane did not contribute to mitral leaflet septal-lateral separation in the x-y plane. This stems from the fact that the posterior papillary tip is attached to both anterior and posterior leaflets and any papillary tip displacement in the x-y plane would bring about a similar x-y displacement of both leaflets. Thus in this model, annular dilatation is required to produce septal-lateral leaflet separation. By fixing the septal-lateral dimension of the mitral annulus, annuloplasty rings prevented septal-lateral separation of the edges of the mitral leaflets. The flexible ring, however, allowed posterior displacement of the lateral annulus, which drew the PMID coordinate posteriorly relative to the AMID (Fig. 3B).
Like a ‘tug-of-war’, we hypothesized that displacement of the mitral annulus away from a papillary tip (i.e. an increase in annular-papillary distance) would result in restriction of the leaflet anatomically attached to both structures, a phenomenon postulated nearly 20 years ago by Boltwood [17]. Indeed, linear regression showed that displacement of the right lateral annulus away from the non-ischemic APM predicted restriction of PMID attached to the both the lateral annulus and APM. In the Physio group, the right lateral annulus was not displaced relative to the non-ischemic APM, which accounts for the absence of PMID restriction. In contrast to the control group, displacement of the right lateral annulus by 1.0±0.7 mm away from the APM in the Duran group unexpectedly did not lead to PMID restriction, as the posterior leaflet was already ‘frozen’ and perhaps maximally restricted already by annular changes induced by the annuloplasty ring per se [20,21]. In echocardiographic studies [22], annular-papillary distances have been measured only from the septal annulus because the septal annulus is easy to identify. The annular-papillary distances measured from the septal annulus, however, should only be used to describe anterior leaflet restriction as the posterior mitral leaflet is not attached to the septal annulus and cannot be involved in a tug-of-war between the papillary muscles and the septal annulus. Extrapolating changes in the annular-papillary distances measured from the septal annulus to restriction of the posterior leaflet could lead to spurious conclusions.
Previous investigators have largely favored papillary displacement [9,10,14–16,23] over annular dilatation as the predominant cause of acute IMR. In the present experiment, however, septal-lateral leaflet separation and Carpentier type IIIb leaflet restriction in control animals were predicted by lateral annular displacement away from the AMID and APM, respectively, indicating that mitral annular dilatation was the predominant responsible mechanism for leaflet malcoaptation during acute circumflex occlusion. Furthermore, annuloplasty rings did not prevent misalignment of the PPM yet preserved valvular competence by preventing lateral annular displacement, affirming the importance of the annulus, and particularly of the septal-lateral annular dimension (clinically referred to as between the middle of the anterior and the middle of the posterior annulus) in the genesis of acute IMR. The relative dominance of either mechanism, however, would depend on the region of LV ischemia and the magnitude of the ischemic injury [24] as mitral annular dilatation is usually seen in cases of inferior LV ischemia [24].
4.3 Implications
Analysis of the data obtained in this acute experiment indicates that either type of annuloplasty ring can prevent the leaflet and annular, but not subvalvular, misalignments associated with acute IMR in previously normal ovine hearts. Given the observed mechanistic importance of mitral septal-lateral annular dilatation in the genesis of acute IMR, annuloplasty rings for patients with IMR should be designed primarily to reduce the septal-lateral annular dimension, which should minimize leaflet edge separation. Ring flexibility allowing additional annular deformation in the antero-posterior (or commissure-commissure) axis did not compromise valvular competence in this acute model.
The perturbations in mitral leaflet 3-D geometry that accompany acute IMR were small, but consistent with the concept advocated previously by Gorman and colleagues [23] and ourselves [3–5] that multiple submillimeter perturbations in the 3-D spatial subunits of the mitral ‘valvular-ventricular complex’ are instrumental in the genesis of leaflet malcoaptation and acute IMR. In contrast, in the setting of chronic IMR, larger derangements of LV geometry [11,25] with 9–13 mm posterolateral and apical displacement of the papillary tips were associated with larger amounts of systolic leaflet restriction measuring upwards of 4 mm [11,25]. Thus, failure of mitral repair using annuloplasty rings in patients with chronic IMR [2] may reflect basic differences in the mechanistic basis of IMR between acute and chronic LV ischemia. Acute IMR in normal hearts appears to be primarily due to annular perturbations, but chronic IMR is most likely due to subvalvular perturbations given the increased magnitude of PPM displacement driven by post-infarction LV remodeling [11,25]. Mitral annuloplasty rings do not remedy subvalvular derangements in acute LV ischemia, and thus would be unlikely to correct the larger magnitude derangements in mitral subvalvular 3-D geometry in patients with chronic LV ischemia; hence, other measures, such as ventricular plication [11], an Alfieri stitch, or a circular inter-papillary plication suture may be necessary to restore subvalvular geometry and valvular competence in patients with chronic IMR.
Interpretation of 3-D mitral valve geometry is totally dependent on the frame of reference. The advantages of using the mitral annular plane as a frame of reference have been previously discussed [4]. Of the many other reference systems we have explored to date, this analysis indicates that a floating internal coordinate system based on the mitral annular plane and centered at the anterior mitral leaflet edge can be useful in evaluating and interpreting the 3-D geometrical relationships of the motion of one leaflet with respect to the other and also amongst the various spatial components of the mitral valve apparatus.
4.4 Limitations
Transthoracic echocardiography could not localize the site or timing of the MR jet; thus, although we could confirm the presence or absence of IMR, we have no data to confirm whether the MR occurred at end-systole. Nevertheless, geometric data analyzed at mid-systole (50% ejection of LV volume) demonstrated septal-lateral separation of the mitral leaflet edges, suggesting that IMR was a pansystolic phenomenon. The leaflet markers were placed only in the midline, but subsequently we have performed experiments using a larger and denser leaflet marker array to study leaflet perturbations at other coaptation sites. The myocardial marker method provides reproducible determination of 3-D marker position with submillimeter spatial resolution every 16.7 ms, but requires suturing small metal markers to the leaflets. It is unlikely that the markers interfered with mitral leaflet motion, as they are very small (aggregate mass=20±6 mg) [4]. We also have no data on the differences in the perturbations of mitral valve complex 3-D geometry that might arise with varying the extent of acute LV ischemia. Finally, a major limitation of this work is the acute animal preparation; a chronic model is required to address the question of chronic infarction with its attendant IMR, LV remodeling, and chronic LV volume overload.
Supported by grants HL-29589 and HL-67025 from the National Heart, Lung, and Blood Institute and accomplished during the tenure of a fellowship from the American Heart Association, Western States Affiliate, awarded to Doctor Lai. Doctors Green, Timek and Tibayan were supported by NHLBI Individual Research Service Awards HL-09569, HL-10452, and HL-67563; Doctors Green, Timek, and Lai were Carl and Leah McConnell Cardiovascular Surgical Research Fellows. Doctor Timek was a recipient of Thoracic Surgery Education and Research Foundation Fellowship Awards. We appreciate the technical assistance provided by Mary K. Zasio, B.A., Carol W. Mead, B.A., and Erin M. Schultz, B.S. We acknowledge and thank Julie R. Glasson, M.D. for her earlier work on these animals in our laboratory.