-
PDF
- Split View
-
Views
-
Cite
Cite
Yankai Mao, Jürgen Duchenne, Yuan Yang, Christophe Garweg, Ying Yang, Xia Sheng, Jiefang Zhang, Yang Ye, Min Wang, Maria F Paton, Alexis Puvrez, Gabor Vöros, Mingming Ma, Guosheng Fu, Jens-Uwe Voigt, Left bundle branch pacing better preserves ventricular mechanical synchrony than right ventricular pacing: a two-centre study, European Heart Journal - Cardiovascular Imaging, Volume 25, Issue 3, March 2024, Pages 328–336, https://doi.org/10.1093/ehjci/jead296
- Share Icon Share
Abstract
Left bundle branch pacing (LBBP) has been shown to better maintain electrical synchrony compared with right ventricular pacing (RVP), but little is known about its impact on mechanical synchrony. This study investigates whether LBBP better preserves left ventricular (LV) mechanical synchronicity and function compared with RVP.
Sixty patients with pacing indication for bradycardia were included: LBBP (n = 31) and RVP (n = 29). Echocardiography was performed before and shortly after pacemaker implantation and at 1-year follow-up. The lateral wall–septal wall (LW–SW) work difference was used as a measure of mechanical dyssynchrony. Septal flash, apical rocking, and septal strain patterns were also assessed. At baseline, LW–SW work difference was small and similar in two groups. SW was markedly decreased, while LW work remained mostly unchanged in RVP, resulting in a larger LW–SW work difference compared with LBBP (1253 ± 687 mmHg·% vs. 439 ± 408 mmHg·%, P < 0.01) at last follow-up. In addition, RVP more often induced septal flash or apical rocking and resulted in more advanced strain patterns compared with LBBP. At 1 year follow-up, LV ejection fraction (EF) and global longitudinal strain (GLS) were more decreased in RVP compared with LBBP (ΔLVEF: −7.4 ± 7.0% vs. 0.3 ± 4.1%; ΔLVGLS: −4.8 ± 4.0% vs. −1.4 ± 2.5%, both P < 0.01). In addition, ΔLW–SW work difference was independently correlated with LV adverse remodelling (r = 0.42, P < 0.01) and LV dysfunction (ΔLVEF: r = −0.61, P < 0.01 and ΔLVGLS: r = −0.38, P = 0.02).
LBBP causes less LV mechanical dyssynchrony than RVP as it preserves a more physiologic electrical conduction. As a consequence, LBBP appears to preserve LV function better than RVP.
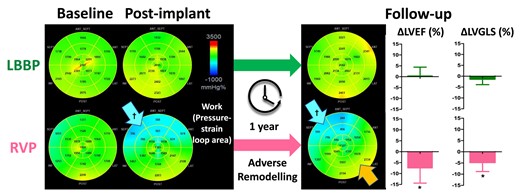
LBBP preserved LV work homogeneity and function better than RVP shortly after device implantation and during follow-up. The panels illustrate how two different pacing modalities affected LV work asymmetry differently. At baseline, the regional work was homogeneously distributed in each group. After device implantation, the myocardial work was immediately markedly reduced in septum (blue arrow) and later also mildly elevated in the lateral region (orange arrow) in RVP patients but remained stable in the LBBP group. Bar graphs: After 1 year follow-up, LBBP has less reduction in LVEF and LVGLS compared with RVP, even after adjusting for baseline LVEF or LVGLS, pacing mode, and valvular disease. ΔLVEF/ΔLVGLS, change in LV ejection fraction/global longitudinal strain from baseline to follow-up; LBBP, left bundle branch pacing; RVP, right ventricular pacing; LV, left ventricular; LVEF, LV ejection fraction; LVGLS, LV global longitudinal strain. *P < 0.01 vs. LBBP; †P < 0.01 vs. baseline.
See the editorial comment for this article ‘Maintaining mechanical synchrony with left bundle branch area pacing', by H. Burri, https://doi.org/10.1093/ehjci/jead310.
Introduction
Right ventricular pacing (RVP) has been used for more than half a century as an effective therapy for bradycardia. However, stimulating the RV introduces electro-mechanical dyssynchrony,1,2 which can lead to left ventricular (LV) systolic dysfunction,3,4 heart failure, and eventually death.5 The dyssynchronous contraction pattern in RVP mimics that of left bundle branch block (LBBB),6,7 which leads to a specific unloading of the early-activated septum and a higher load in the late-activated LV lateral wall (LW).8,9 Earlier studies from our and other research groups have shown that the resulting LW–septal wall (LW–SW) workload asymmetry stimulates adverse LV remodelling in healthy animal hearts with RVP and in patients with LBBB.8,10,11 Similarly, apical rocking (ApRock), septal flash (SF), and speckle tracking-based longitudinal strain patterns reflect RVP-induced or LBBB-induced dyssynchrony and have been shown to be associated with LV remodelling and dysfunction.4,12,13 In reverse, homogenization of workload, inverse transition of strain patterns, or correction of ApRock and/or SF leads to reverse remodelling and functional improvement in many heart failure patients with LBBB or chronic RVP.13–16
To avoid the detrimental effects of RVP, alternative pacing methods have been sought. Recently, left bundle branch pacing (LBBP), defined as pacing of the left bundle branch (LBB) or one of its fascicles along with the LV septal myocardium via a trans-septal approach, has emerged as a new pacing option. Several studies have demonstrated mid-term and long-term feasibility and safety of LBBP in bradycardia patients, with a low capture threshold and high success rates.17–19 Compared with RVP, LBBP better preserves electrical synchrony of the LV, characterized by shorter paced QRS duration and LV activation time.17–20 However, little is known about the impact of LBBP on mechanical dyssynchrony in comparison with RVP.
We hypothesized that LBBP would minimize mechanical dyssynchrony and thus better maintain LV function. We therefore sought to determine whether LBBP better preserves mechanical synchrony, LV remodelling, and function acutely and during follow-up compared with RVP and to further confirm the relationship between the presence of mechanical dyssynchrony and the extent of LV remodelling and dysfunction over time in both LBBP and RVP patients.
Methods
Study population
Consecutive patients who underwent pacemaker implantation with LBBP were enrolled in this observational study at one of the two tertiary hospitals (Sir Run Run Shaw Hospital, Zhejiang University, School of Medicine, China, and University Hospitals Leuven, Belgium) between December 2019 and January 2022. We also identified patients undergoing RVP from the databases in two hospitals. All patients had symptomatic bradycardia. Inclusion criteria included Grade II or III atrioventricular block (AVB), sick sinus syndrome (SSS), or atrial fibrillation (AF) with slow ventricular rate. Exclusion criteria were as follows: heart failure with LV ejection fraction <45%, acute or old myocardial infarction, LBBB morphology, or implanted cardiac devices (more details in the Supplementary data online). The institutional medical ethics committees of the two participating hospitals had approved the protocol, and patients had provided written informed consent.
Pacemaker implantation
All implantation procedures were performed under local anaesthesia by experienced electrophysiologists. Twelve-lead electrocardiogram (ECG) and intracardiac electrograms were simultaneously displayed and recorded on a multi-channel recorder during the procedures.
LBBP was achieved following the methods described elsewhere.19,21 The pacing lead (models 3830, Medtronic Inc, Minneapolis, MN, USA) was screwed deeply into the inter-ventricular septum, so that the paced QRS complex changed from an LBBB to a right bundle branch block (RBBB) morphology in surface lead V1 when the lead tip reached the LV septal subendocardium. LBB capture was confirmed by the abrupt shortening of the stimulus to LV activation time (Stim-LVAT) to <75 ms, which remains constant both at low and high outputs, as well as by observing the LBB potential during intrinsic rhythm.21
RVP was achieved by positioning a ventricular pacing lead or a leadless pacing system (Micra Transcatheter Pacing System VR, Medtronic) in the right ventricle (RV).
To minimize the impact of the pacing site in RVP, an RV mid-septal position was primarily attempted, and an apical position was used if this attempt failed in conventional RVP. In the patients treated by a leadless pacemaker, apical position was avoided, and a higher septal position was then targeted as it has been previously recommended.22
Electrocardiographic measurements
Pacing parameters (capture threshold, impedance, and sensing amplitude) were recorded by unipolar configurations through the programmer during procedures. Baseline QRS duration was measured at the speed of 100 mm/s during intrinsic rhythm (from the onset to the end of QRS complex in all 12 leads). Paced QRS duration was measured during unipolar pacing at 3.5 V/0.4 ms (from the stimulus to the end of QRS complex in all 12 leads). The Stim-LVAT was measured from the pacing stimulus to the peak of R-wave in lead V5 in LBBP patients. High-output and low-output pacing (usually >5 V/0.4 ms and <1 V/0.4 ms) were repeated on the next day of implantation to confirm the capture of LBB. During follow-up, QRS morphology, QRS width, and Stim-LVAT on surface ECG were examined to see if LBBP was maintained.
Echocardiographic examination
Transthoracic echocardiography was performed using commercial Vivid E95 scanners (GE Vingmed Ultrasound AS, Horten, Norway) equipped with an M5Sc probe, at baseline, 1 week after pacemaker implantation, and 1-year follow-up. Apical four-chamber, two-chamber, and three-chamber views were acquired for at least five consecutive cycles at 65 ± 12 frames/s and digitally stored for later offline analysis. Blood pressure by the brachial cuff method was measured at the beginning of each examination.
Conventional echocardiographic data analysis
Echo data were analysed using a dedicated workstation (EchoPAC version 204, GE Vingmed). For all parameters, measurements from three (sinus rhythm) or five (AF) cardiac cycles were averaged. LV end-diastolic volume (EDV), end-systolic volume (ESV), and LV ejection fraction (EF) were determined by the modified Simpson’s method. RV dimensions were determined by measuring end-diastolic area (EDA) from a RV-focused apical four-chamber view. LV EDV, LV ESV, and RV EDA were subsequently indexed to body surface area (iLVEDV, iLVESV, and iRVEDA). Tricuspid regurgitation (TR) was assessed visually, and the degree of TR was categorized as none, mild, moderate, or severe.
The 2D strain function of the software was used to analyse LV global longitudinal strain (GLS) and segmental strain.23,24 The ECG R-trigger served as zero strain reference. Timing of valvular events was measured from spectral Doppler recordings.25 In the following, GLS will be presented in absolute values.
Estimation of regional work
Myocardial work (MW) analysis was performed in Leuven by an observer blinded to other patient data, using the work analysis package of the workstation software (EchoPAC version 204, GE Vingmed).26 MW in the SW and LW was averaged from the respective basal and mid segments in the apical three-chamber and four-chamber views, and the LW–SW work difference was calculated from the two averages. MW in the anterior and inferior wall was calculated as the average of basal and mid segments in the apical two-chamber view.
Assessment of mechanical dyssynchrony
ApRock,12 SF,16 and septal longitudinal strain patterns13 were assessed visually by two experienced readers (Y.M., J.D.). Strain patterns were classified into four stages as described earlier,13 with Stage 1 showing an early notching in the systolic down slope of the curve followed by late peak strain before aortic valve closure, Stages 2 and 3 both presenting double-peaked patterns, and Stage 4 showing early peak strain of the septum followed by stretching during late systole.
Statistical analysis
Continuous data are presented as mean ± standard deviation for normally distributed variables and median (inter-quartile range) for non-normally distributed variables. Categorical variables are reported as number and percentages. Comparisons between two groups at baseline or follow-up were performed using independent t-test, Mann–Whitney U test, χ2 test, or Fisher’s exact test as appropriate. The repeated measures ANOVA with post hoc analyses or non-parametric tests for related samples were used to compare parameters obtained at baseline, 1 week after pacing, and last follow-up. A general linear model was used to investigate the independent association between two pacing strategies and the change of multiple echocardiographic parameters during follow-up. Baseline value of the respective parameters, pacing mode, and presence of valvular disease were included in the general linear model as covariates on the basis of baseline characteristics of the cohort, clinical experience, and previous findings.27 Univariate and multivariate linear regression analyses were used to identify independent associations among ΔiLVESV, ΔLVEF, and ΔLVGLS during follow-up and ΔLW–SW work difference. Inter-observer and intra-observer agreement for LW–SW work difference was studied in a random sample of 20 patients by two independent investigators on two different occasions. Reproducibility was expressed as intra-class correlation coefficient (ICC). IBM SPSS package 25.0 (SPSS, Inc., Chicago, IL, USA) was used to perform the statistical analyses. Statistical significance was defined as P < 0.05.
Results
Participants
A total of 82 bradycardia patients were enrolled [48 from China (LBBP, n = 37; RVP, n = 11), 34 from Belgium (LBBP, n = 2; RVP, n = 32)] during the study period. After exclusion of 22 patients due to poor image quality (n = 9) or ventricle not paced during follow-up echocardiography (n = 6) or lack of follow-up echo (n = 7), 60 remaining patients formed the final study group. The disposition of subjects is presented in Supplementary data online, Figure S1. Among those, 31 patients received LBBP (29 from China and 2 from Belgium, mean 71 ± 10 years; 58% male) and 29 had RVP (4 from China and 25 from Belgium, mean age 75 ± 9 years; 79% male). Of the 29 RVP patients, 8 were treated with leadless pacemakers. All patients in the LBBP group and 13 patients with conventional RVP received dual-chamber pacemakers (Medtronic Inc) with the atrial pacing leads in the right auricular appendix. Those receiving LBBP had a lower prevalence of valvular disease. Table 1 summarizes the clinical characteristics of all patients. All patients underwent an early echocardiography within 1 week after implantation except five in the LBBP group. A follow-up examination was available at 15 ± 9 months in all patients. Work analysis could be performed in all 60 patients at baseline and in 54 shortly after device implantation. At last follow-up, work analysis was not feasible in three patients due to the incorrect raw DICOM format.
Variables . | RVP (N = 29) . | LBBP (N = 31) . | P-value . |
---|---|---|---|
Demographics | |||
Male | 23 (79) | 18 (58) | 0.10 |
Age, years | 75 ± 9 | 71 ± 10 | 0.11 |
BMI, kg/m2 | 25 ± 4 | 25 ± 4 | 0.74 |
Comorbidities | |||
Atrial fibrillation | 10 (35) | 5 (16) | 0.14 |
Coronary artery disease | 7 (24) | 6 (19) | 0.65 |
Hypertension | 16 (55) | 17 (55) | 0.98 |
Diabetes | 9 (31) | 6 (19) | 0.30 |
Hyperlipidaemia | 13 (45) | 14 (45) | 0.98 |
Valvular disease | 13 (45) | 3 (10) | <0.01 |
Aortic valve replacement | 9 (31) | 0 (0) | <0.01 |
Mitral valve repair or replacement | 5 (17) | 0 (0) | <0.01 |
Moderate to severe TR | 5 (17) | 3 (10) | 0.45 |
Pacing indication | 0.82 | ||
SSS | 9 (31) | 12 (39) | |
AVB | 19 (66) | 18 (58) | |
Slow AF | 1 (3) | 1 (3) | |
Blood pressure | |||
Systolic, mmHg | 134 ± 20 | 135 ± 14 | 0.94 |
Diastolic, mmHg | 71 ± 10 | 75 ± 10 | 0.15 |
QRS pattern | |||
RBBB | 15 (52) | 13 (42) | 0.45 |
QRS duration | 114 ± 22 | 107 ± 24 | 0.24 |
Variables . | RVP (N = 29) . | LBBP (N = 31) . | P-value . |
---|---|---|---|
Demographics | |||
Male | 23 (79) | 18 (58) | 0.10 |
Age, years | 75 ± 9 | 71 ± 10 | 0.11 |
BMI, kg/m2 | 25 ± 4 | 25 ± 4 | 0.74 |
Comorbidities | |||
Atrial fibrillation | 10 (35) | 5 (16) | 0.14 |
Coronary artery disease | 7 (24) | 6 (19) | 0.65 |
Hypertension | 16 (55) | 17 (55) | 0.98 |
Diabetes | 9 (31) | 6 (19) | 0.30 |
Hyperlipidaemia | 13 (45) | 14 (45) | 0.98 |
Valvular disease | 13 (45) | 3 (10) | <0.01 |
Aortic valve replacement | 9 (31) | 0 (0) | <0.01 |
Mitral valve repair or replacement | 5 (17) | 0 (0) | <0.01 |
Moderate to severe TR | 5 (17) | 3 (10) | 0.45 |
Pacing indication | 0.82 | ||
SSS | 9 (31) | 12 (39) | |
AVB | 19 (66) | 18 (58) | |
Slow AF | 1 (3) | 1 (3) | |
Blood pressure | |||
Systolic, mmHg | 134 ± 20 | 135 ± 14 | 0.94 |
Diastolic, mmHg | 71 ± 10 | 75 ± 10 | 0.15 |
QRS pattern | |||
RBBB | 15 (52) | 13 (42) | 0.45 |
QRS duration | 114 ± 22 | 107 ± 24 | 0.24 |
Values are mean ± SD, n (%), or median (inter-quartile range).
AF, atrial fibrillation; AVB, atrioventricular block; BMI, body mass index; RVP, right ventricular pacing; LBBP, left bundle brunch pacing; RBBB, right bundle branch block; SSS, sick sinus syndrome; TR, tricuspid regurgitation.
Variables . | RVP (N = 29) . | LBBP (N = 31) . | P-value . |
---|---|---|---|
Demographics | |||
Male | 23 (79) | 18 (58) | 0.10 |
Age, years | 75 ± 9 | 71 ± 10 | 0.11 |
BMI, kg/m2 | 25 ± 4 | 25 ± 4 | 0.74 |
Comorbidities | |||
Atrial fibrillation | 10 (35) | 5 (16) | 0.14 |
Coronary artery disease | 7 (24) | 6 (19) | 0.65 |
Hypertension | 16 (55) | 17 (55) | 0.98 |
Diabetes | 9 (31) | 6 (19) | 0.30 |
Hyperlipidaemia | 13 (45) | 14 (45) | 0.98 |
Valvular disease | 13 (45) | 3 (10) | <0.01 |
Aortic valve replacement | 9 (31) | 0 (0) | <0.01 |
Mitral valve repair or replacement | 5 (17) | 0 (0) | <0.01 |
Moderate to severe TR | 5 (17) | 3 (10) | 0.45 |
Pacing indication | 0.82 | ||
SSS | 9 (31) | 12 (39) | |
AVB | 19 (66) | 18 (58) | |
Slow AF | 1 (3) | 1 (3) | |
Blood pressure | |||
Systolic, mmHg | 134 ± 20 | 135 ± 14 | 0.94 |
Diastolic, mmHg | 71 ± 10 | 75 ± 10 | 0.15 |
QRS pattern | |||
RBBB | 15 (52) | 13 (42) | 0.45 |
QRS duration | 114 ± 22 | 107 ± 24 | 0.24 |
Variables . | RVP (N = 29) . | LBBP (N = 31) . | P-value . |
---|---|---|---|
Demographics | |||
Male | 23 (79) | 18 (58) | 0.10 |
Age, years | 75 ± 9 | 71 ± 10 | 0.11 |
BMI, kg/m2 | 25 ± 4 | 25 ± 4 | 0.74 |
Comorbidities | |||
Atrial fibrillation | 10 (35) | 5 (16) | 0.14 |
Coronary artery disease | 7 (24) | 6 (19) | 0.65 |
Hypertension | 16 (55) | 17 (55) | 0.98 |
Diabetes | 9 (31) | 6 (19) | 0.30 |
Hyperlipidaemia | 13 (45) | 14 (45) | 0.98 |
Valvular disease | 13 (45) | 3 (10) | <0.01 |
Aortic valve replacement | 9 (31) | 0 (0) | <0.01 |
Mitral valve repair or replacement | 5 (17) | 0 (0) | <0.01 |
Moderate to severe TR | 5 (17) | 3 (10) | 0.45 |
Pacing indication | 0.82 | ||
SSS | 9 (31) | 12 (39) | |
AVB | 19 (66) | 18 (58) | |
Slow AF | 1 (3) | 1 (3) | |
Blood pressure | |||
Systolic, mmHg | 134 ± 20 | 135 ± 14 | 0.94 |
Diastolic, mmHg | 71 ± 10 | 75 ± 10 | 0.15 |
QRS pattern | |||
RBBB | 15 (52) | 13 (42) | 0.45 |
QRS duration | 114 ± 22 | 107 ± 24 | 0.24 |
Values are mean ± SD, n (%), or median (inter-quartile range).
AF, atrial fibrillation; AVB, atrioventricular block; BMI, body mass index; RVP, right ventricular pacing; LBBP, left bundle brunch pacing; RBBB, right bundle branch block; SSS, sick sinus syndrome; TR, tricuspid regurgitation.
Pacing parameters and electrophysiological characteristics
There were no significant differences in intrinsic QRS duration at baseline between the LBBP and RVP group (see Supplementary data online, Table S1). Those receiving RVP had a higher pacing impedance and lower pacing threshold than LBBP. The ventricular pacing percentages were similar between two groups (RVP: median 94% vs. LBBP: median 95% P = 0.85). Nearly half of the patients with RVP (45%) had the RV lead in mid septum, while 28% had a higher septal position and 28% used an apical position. RVP demonstrated a significantly larger increase in paced QRS duration during follow-up compared with LBBP (ΔQRS duration: 51 ± 26 ms vs. 18 ± 20 ms, P < 0.01). The Stim-LVAT was 69 ± 9 ms in the LBBP group. In 74% cases (23/31), the LBB potential during intrinsic rhythm was observed at the final lead location as another indication of LBB capture. In two patients, there was loss of LBB capture at 6 months after implantation, but local LV septal capture was maintained, with a QS pattern in Lead V1 and a mean QRS duration >130 ms.
Myocardial work
At baseline, LV MW was homogeneously distributed in both groups, as reflected by the small LW–SW work difference, which was not significantly different between two groups. During follow-up, global MW was preserved in LBBP but was markedly decreased in the RVP group (2039 ± 369 vs. 1457 ± 537 mmHg·%, P < 0.01). We further noticed a significant work re-distribution in the RVP group at post-implantation and during follow-up (Graphical Abstract), with septal work decreasing strongly from 1825 ± 425 to 845 ± 571 mmHg·% (P < 0.01), while lateral work remained largely unchanged, resulting in a marked increase of LW–SW work difference (Table 2). In contrast to that, LBBP showed only a slightly increased LW–SW work difference at post-implantation and during follow-up, which was also significantly smaller than that of the RVP group (Figure 1). Sub-analysis with each centre revealed similar results for LW–SW work difference (see Supplementary data online, Figure S2).

Left ventricular work asymmetry indicated by septal and lateral work (upper panels) and by the lateral wall to septal work difference (lower panels). Left ventricular work is evenly distributed at baseline in all patients. Pacing induced a significant lateral-to-septal work difference in RVP patients. LBBP, left bundle branch pacing; LW-SW, lateral-to-septal; RVP, right ventricular pacing. *P < 0.01 vs. LBBP; †P < 0.05 vs. baseline.
Global and segmental myocardial work in the LBBP and RVP groups at baseline and during follow-up
Parameters . | RVP . | LBBP . | P-value . |
---|---|---|---|
Baseline | n = 29 | n = 31 | |
Global MW, mmHg·% | 2055 ± 439 | 2106 ± 391 | 0.64 |
Inferior MW, mmHg·% | 1807 ± 536 | 1923 ± 517 | 0.40 |
Anterior MW, mmHg·% | 1819 ± 382 | 1878 ± 567 | 0.64 |
Lateral MW, mmHg·% | 1939 ± 420 | 1962 ± 371 | 0.82 |
Septal MW, mmHg·% | 1825 ± 425 | 1833 ± 373 | 0.94 |
LW–SW work difference, mmHg·% | 114 ± 276 | 129 ± 297 | 0.84 |
Post-implantation | n = 28 | n = 26 | |
Global MW, mmHg·% | 1694 ± 450* | 2004 ± 359 | <0.01 |
Inferior MW, mmHg·% | 1524 ± 457 | 1670 ± 479 | 0.26 |
Anterior MW, mmHg·% | 1625 ± 604 | 1738 ± 717 | 0.54 |
Lateral MW, mmHg·% | 2166 ± 451 | 2035 ± 342 | 0.24 |
Septal MW, mmHg·% | 962 ± 429* | 1592 ± 313* | <0.01 |
LW–SW work difference, mmHg·% | 1204 ± 543* | 443 ± 309* | <0.01 |
Last follow-up | (n = 27) | (n = 30) | |
Global MW, mmHg·% | 1457 ± 537* | 2039 ± 369 | <0.01 |
Inferior MW, mmHg·% | 1536 ± 648 | 1747 ± 563 | 0.19 |
Anterior MW, mmHg·% | 1362 ± 615* | 1821 ± 504 | <0.01 |
Lateral MW, mmHg·% | 2098 ± 625 | 2180 ± 369 | 0.55 |
Septal MW, mmHg·% | 845 ± 571* | 1741 ± 428 | <0.01 |
LW–SW work difference, mmHg·% | 1253 ± 687* | 439 ± 408* | <0.01 |
Parameters . | RVP . | LBBP . | P-value . |
---|---|---|---|
Baseline | n = 29 | n = 31 | |
Global MW, mmHg·% | 2055 ± 439 | 2106 ± 391 | 0.64 |
Inferior MW, mmHg·% | 1807 ± 536 | 1923 ± 517 | 0.40 |
Anterior MW, mmHg·% | 1819 ± 382 | 1878 ± 567 | 0.64 |
Lateral MW, mmHg·% | 1939 ± 420 | 1962 ± 371 | 0.82 |
Septal MW, mmHg·% | 1825 ± 425 | 1833 ± 373 | 0.94 |
LW–SW work difference, mmHg·% | 114 ± 276 | 129 ± 297 | 0.84 |
Post-implantation | n = 28 | n = 26 | |
Global MW, mmHg·% | 1694 ± 450* | 2004 ± 359 | <0.01 |
Inferior MW, mmHg·% | 1524 ± 457 | 1670 ± 479 | 0.26 |
Anterior MW, mmHg·% | 1625 ± 604 | 1738 ± 717 | 0.54 |
Lateral MW, mmHg·% | 2166 ± 451 | 2035 ± 342 | 0.24 |
Septal MW, mmHg·% | 962 ± 429* | 1592 ± 313* | <0.01 |
LW–SW work difference, mmHg·% | 1204 ± 543* | 443 ± 309* | <0.01 |
Last follow-up | (n = 27) | (n = 30) | |
Global MW, mmHg·% | 1457 ± 537* | 2039 ± 369 | <0.01 |
Inferior MW, mmHg·% | 1536 ± 648 | 1747 ± 563 | 0.19 |
Anterior MW, mmHg·% | 1362 ± 615* | 1821 ± 504 | <0.01 |
Lateral MW, mmHg·% | 2098 ± 625 | 2180 ± 369 | 0.55 |
Septal MW, mmHg·% | 845 ± 571* | 1741 ± 428 | <0.01 |
LW–SW work difference, mmHg·% | 1253 ± 687* | 439 ± 408* | <0.01 |
*P < 0.05 vs. baseline.
LBBP, left bundle branch pacing; LW–SW, lateral wall to septum; MW, myocardial work; RVP, right ventricular pacing.
Global and segmental myocardial work in the LBBP and RVP groups at baseline and during follow-up
Parameters . | RVP . | LBBP . | P-value . |
---|---|---|---|
Baseline | n = 29 | n = 31 | |
Global MW, mmHg·% | 2055 ± 439 | 2106 ± 391 | 0.64 |
Inferior MW, mmHg·% | 1807 ± 536 | 1923 ± 517 | 0.40 |
Anterior MW, mmHg·% | 1819 ± 382 | 1878 ± 567 | 0.64 |
Lateral MW, mmHg·% | 1939 ± 420 | 1962 ± 371 | 0.82 |
Septal MW, mmHg·% | 1825 ± 425 | 1833 ± 373 | 0.94 |
LW–SW work difference, mmHg·% | 114 ± 276 | 129 ± 297 | 0.84 |
Post-implantation | n = 28 | n = 26 | |
Global MW, mmHg·% | 1694 ± 450* | 2004 ± 359 | <0.01 |
Inferior MW, mmHg·% | 1524 ± 457 | 1670 ± 479 | 0.26 |
Anterior MW, mmHg·% | 1625 ± 604 | 1738 ± 717 | 0.54 |
Lateral MW, mmHg·% | 2166 ± 451 | 2035 ± 342 | 0.24 |
Septal MW, mmHg·% | 962 ± 429* | 1592 ± 313* | <0.01 |
LW–SW work difference, mmHg·% | 1204 ± 543* | 443 ± 309* | <0.01 |
Last follow-up | (n = 27) | (n = 30) | |
Global MW, mmHg·% | 1457 ± 537* | 2039 ± 369 | <0.01 |
Inferior MW, mmHg·% | 1536 ± 648 | 1747 ± 563 | 0.19 |
Anterior MW, mmHg·% | 1362 ± 615* | 1821 ± 504 | <0.01 |
Lateral MW, mmHg·% | 2098 ± 625 | 2180 ± 369 | 0.55 |
Septal MW, mmHg·% | 845 ± 571* | 1741 ± 428 | <0.01 |
LW–SW work difference, mmHg·% | 1253 ± 687* | 439 ± 408* | <0.01 |
Parameters . | RVP . | LBBP . | P-value . |
---|---|---|---|
Baseline | n = 29 | n = 31 | |
Global MW, mmHg·% | 2055 ± 439 | 2106 ± 391 | 0.64 |
Inferior MW, mmHg·% | 1807 ± 536 | 1923 ± 517 | 0.40 |
Anterior MW, mmHg·% | 1819 ± 382 | 1878 ± 567 | 0.64 |
Lateral MW, mmHg·% | 1939 ± 420 | 1962 ± 371 | 0.82 |
Septal MW, mmHg·% | 1825 ± 425 | 1833 ± 373 | 0.94 |
LW–SW work difference, mmHg·% | 114 ± 276 | 129 ± 297 | 0.84 |
Post-implantation | n = 28 | n = 26 | |
Global MW, mmHg·% | 1694 ± 450* | 2004 ± 359 | <0.01 |
Inferior MW, mmHg·% | 1524 ± 457 | 1670 ± 479 | 0.26 |
Anterior MW, mmHg·% | 1625 ± 604 | 1738 ± 717 | 0.54 |
Lateral MW, mmHg·% | 2166 ± 451 | 2035 ± 342 | 0.24 |
Septal MW, mmHg·% | 962 ± 429* | 1592 ± 313* | <0.01 |
LW–SW work difference, mmHg·% | 1204 ± 543* | 443 ± 309* | <0.01 |
Last follow-up | (n = 27) | (n = 30) | |
Global MW, mmHg·% | 1457 ± 537* | 2039 ± 369 | <0.01 |
Inferior MW, mmHg·% | 1536 ± 648 | 1747 ± 563 | 0.19 |
Anterior MW, mmHg·% | 1362 ± 615* | 1821 ± 504 | <0.01 |
Lateral MW, mmHg·% | 2098 ± 625 | 2180 ± 369 | 0.55 |
Septal MW, mmHg·% | 845 ± 571* | 1741 ± 428 | <0.01 |
LW–SW work difference, mmHg·% | 1253 ± 687* | 439 ± 408* | <0.01 |
*P < 0.05 vs. baseline.
LBBP, left bundle branch pacing; LW–SW, lateral wall to septum; MW, myocardial work; RVP, right ventricular pacing.
A general linear model was used to further compare changes of MW during follow-up between the two pacing modalities, after controlling for covariates including baseline value of the respective parameters, pacing mode, and presence of valvular disease (see Supplementary data online, Table S2). Compared with the RVP group, LBBP was independently associated with smaller decrease in global work (Δglobal work: −57 ± 482 vs. −583 ± 465 mmHg·%, P < 0.01). LBBP further showed significantly less reduction in septal work (Δseptal work: −88 ± 538 vs. −975 ± 553 mmHg·%, P < 0.01) and consequently much smaller increase in LW–SW work difference (ΔLW–SW work difference: 296 ± 370 vs. 1157 ± 662 mmHg·%, P < 0.01) than RVP (Figure 2). Additionally, we observed a similar decline of MW in the inferior and anterior wall in both groups. Intra-observer and inter-observer ICC for LW–SW work difference was 0.95 [95% confidence interval (CI): 0.88–0.98] and 0.90 (95% CI: 0.79–0.97), respectively.
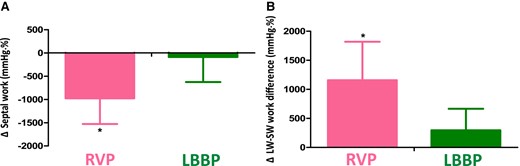
Comparison of changes in septal work and lateral wall to septum work difference from baseline to last follow-up between LBBP and RVP. LBBP has much smaller decrease in septal work (A) and thus less increase in LW–SW work differences (B) compared with RVP, even after adjusting for baseline value of the respective parameters, pacing mode, and valvular disease. *P < 0.01 vs. LBBP.
Assessment of other mechanical dyssynchrony parameters
Most of the patients in the RVP group showed SF (93%) and ApRock (90%) once being paced. In contrast, in the LBBP group, SF and ApRock were less common (8% and 42%, both P < 0.01 vs. RVP). Nearly all the RVP patients (96%) presented with mild changes in septal strain patterns (Stage 1 or 2) after device implantation, and 11 patients progressed towards more advanced stages during follow-up. In contrast, shortly after implantation, one-third of LBBP patients preserved a normal strain pattern, while 69% were in Stage 1. During follow-up, only two of them progressed to Stage 2 (see Supplementary data online, Table S3). Representative strain curves are shown in Supplementary data online, Figure S3.
By using univariate linear regression analyses, we could show a significant association between LW–SW work difference and dyssynchrony parameters including septal strain patterns, SF, ApRock, and paced QRS duration following device implantation (see Supplementary data online, Table S4).
LV function
During follow-up, the overall change of iLVEDV was not significant in the RVP group, while LBBP showed a small decrease (Table 3; see Supplementary data online, Figure S4). In the RVP group, a significant increase in iLVESV over time was noticed, but LBBP patients exhibited a significant decline. LVEF and LVGLS were therefore substantially decreased in the RVP group, while no significant changes were noted in LBBP patients. Multivariate linear regression showed that LBBP was independently associated with less decrease in LVEF (0.3 ± 4.1 vs. −7.4 ± 7.0%, P < 0.01) and less reduction in LVGLS than the RVP group (−1.4 ± 2.5 vs. −4.8 ± 4.0%, P < 0.01) after adjusting for baseline LVEF or LVGLS, pacing mode, and valvular disease (Graphical Abstract; see Supplementary data online, Table S2).
. | RVP . | LBBP . | P-value . |
---|---|---|---|
Baseline | N = 29 | N = 31 | |
Septal thickness, mm | 12 ± 3 | 11 ± 2 | 0.10 |
LV posterior wall thickness, mm | 11 ± 2 | 10 ± 2 | 0.24 |
LV mass index, g/m2 | 113 ± 32 | 105 ± 28 | 0.29 |
iLVEDV, mL/m2 | 57 ± 14 | 54 ± 11 | 0.40 |
iLVESV, mL/m2 | 20 ± 7 | 19 ± 6 | 0.85 |
LVEF, % | 65 ± 7 | 65 ± 6 | 0.71 |
LVGLS, % | 19 ± 4 | 20 ± 3 | 0.61 |
iRVEDA, cm2/m2 | 11 ± 3 | 10 ± 2 | 0.15 |
Tricuspid regurgitation | 0.50 | ||
Mild | 24 (83) | 28 (90) | |
Moderate | 4 (14) | 3 (10) | |
Severe | 1 (3) | 0 (0) | |
Post-implantation | N = 29 | N = 26 | |
iLVEDV, mL/m2 | 54 ± 12 | 49 ± 12* | 0.12 |
iLVESV, mL/m2 | 21 ± 6 | 18 ± 7 | 0.17 |
LVEF, % | 62 ± 7 | 64 ± 7 | 0.35 |
iRVEDA, cm2/m2 | 9.6 (8.8–11.8) | 9 ± 2 | 0.11 |
Tricuspid regurgitation | 0.45 | ||
Mild | 21 (72) | 24 (92) | |
Moderate | 7 (24) | 2 (8) | |
Severe | 1 (3) | 0 (0) | |
N = 28 | N = 26 | ||
LVGLS, % | 17 ± 4* | 19 ± 4 | 0.03 |
At last follow-up | N = 29 | N = 31 | |
iLVEDV, mL/m2 | 58 ± 15 | 48 ± 11* | <0.01 |
iLVESV, mL/m2 | 25 ± 9*,** | 17 ± 7* | <0.01 |
LVEF, % | 58 ± 8*,** | 65 ± 7 | <0.01 |
iRVEDA, cm2/m2 | 10.2 (9.0–11.7) | 9 ± 2 | 0.10 |
Tricuspid regurgitation | |||
Mild | 12 (41) | 23 (74) | 0.02 |
Moderate | 15 (52) | 8 (26) | 0.03 |
Severe | 2 (7) | 0 (0) | NS |
RVP (N = 28) | LBBP (N = 30) | ||
LVGLS, % | 14 ± 4*,** | 18 ± 2 | <0.01 |
. | RVP . | LBBP . | P-value . |
---|---|---|---|
Baseline | N = 29 | N = 31 | |
Septal thickness, mm | 12 ± 3 | 11 ± 2 | 0.10 |
LV posterior wall thickness, mm | 11 ± 2 | 10 ± 2 | 0.24 |
LV mass index, g/m2 | 113 ± 32 | 105 ± 28 | 0.29 |
iLVEDV, mL/m2 | 57 ± 14 | 54 ± 11 | 0.40 |
iLVESV, mL/m2 | 20 ± 7 | 19 ± 6 | 0.85 |
LVEF, % | 65 ± 7 | 65 ± 6 | 0.71 |
LVGLS, % | 19 ± 4 | 20 ± 3 | 0.61 |
iRVEDA, cm2/m2 | 11 ± 3 | 10 ± 2 | 0.15 |
Tricuspid regurgitation | 0.50 | ||
Mild | 24 (83) | 28 (90) | |
Moderate | 4 (14) | 3 (10) | |
Severe | 1 (3) | 0 (0) | |
Post-implantation | N = 29 | N = 26 | |
iLVEDV, mL/m2 | 54 ± 12 | 49 ± 12* | 0.12 |
iLVESV, mL/m2 | 21 ± 6 | 18 ± 7 | 0.17 |
LVEF, % | 62 ± 7 | 64 ± 7 | 0.35 |
iRVEDA, cm2/m2 | 9.6 (8.8–11.8) | 9 ± 2 | 0.11 |
Tricuspid regurgitation | 0.45 | ||
Mild | 21 (72) | 24 (92) | |
Moderate | 7 (24) | 2 (8) | |
Severe | 1 (3) | 0 (0) | |
N = 28 | N = 26 | ||
LVGLS, % | 17 ± 4* | 19 ± 4 | 0.03 |
At last follow-up | N = 29 | N = 31 | |
iLVEDV, mL/m2 | 58 ± 15 | 48 ± 11* | <0.01 |
iLVESV, mL/m2 | 25 ± 9*,** | 17 ± 7* | <0.01 |
LVEF, % | 58 ± 8*,** | 65 ± 7 | <0.01 |
iRVEDA, cm2/m2 | 10.2 (9.0–11.7) | 9 ± 2 | 0.10 |
Tricuspid regurgitation | |||
Mild | 12 (41) | 23 (74) | 0.02 |
Moderate | 15 (52) | 8 (26) | 0.03 |
Severe | 2 (7) | 0 (0) | NS |
RVP (N = 28) | LBBP (N = 30) | ||
LVGLS, % | 14 ± 4*,** | 18 ± 2 | <0.01 |
Values are mean ± SD, n (%), or median (inter-quartile range).
*P < 0.05 vs. baseline; **P < 0.05 vs. post-implantation.
EDA, end-diastolic area; EDV, end-diastolic volume; EF, ejection fraction; ESV, end-systolic volume; GLS, longitudinal global strain; i, indexed to body surface area; LBBP, left bundle branch pacing; LV, left ventricular; NS, not significant; RV, right ventricular; RVP, right ventricular pacing.
. | RVP . | LBBP . | P-value . |
---|---|---|---|
Baseline | N = 29 | N = 31 | |
Septal thickness, mm | 12 ± 3 | 11 ± 2 | 0.10 |
LV posterior wall thickness, mm | 11 ± 2 | 10 ± 2 | 0.24 |
LV mass index, g/m2 | 113 ± 32 | 105 ± 28 | 0.29 |
iLVEDV, mL/m2 | 57 ± 14 | 54 ± 11 | 0.40 |
iLVESV, mL/m2 | 20 ± 7 | 19 ± 6 | 0.85 |
LVEF, % | 65 ± 7 | 65 ± 6 | 0.71 |
LVGLS, % | 19 ± 4 | 20 ± 3 | 0.61 |
iRVEDA, cm2/m2 | 11 ± 3 | 10 ± 2 | 0.15 |
Tricuspid regurgitation | 0.50 | ||
Mild | 24 (83) | 28 (90) | |
Moderate | 4 (14) | 3 (10) | |
Severe | 1 (3) | 0 (0) | |
Post-implantation | N = 29 | N = 26 | |
iLVEDV, mL/m2 | 54 ± 12 | 49 ± 12* | 0.12 |
iLVESV, mL/m2 | 21 ± 6 | 18 ± 7 | 0.17 |
LVEF, % | 62 ± 7 | 64 ± 7 | 0.35 |
iRVEDA, cm2/m2 | 9.6 (8.8–11.8) | 9 ± 2 | 0.11 |
Tricuspid regurgitation | 0.45 | ||
Mild | 21 (72) | 24 (92) | |
Moderate | 7 (24) | 2 (8) | |
Severe | 1 (3) | 0 (0) | |
N = 28 | N = 26 | ||
LVGLS, % | 17 ± 4* | 19 ± 4 | 0.03 |
At last follow-up | N = 29 | N = 31 | |
iLVEDV, mL/m2 | 58 ± 15 | 48 ± 11* | <0.01 |
iLVESV, mL/m2 | 25 ± 9*,** | 17 ± 7* | <0.01 |
LVEF, % | 58 ± 8*,** | 65 ± 7 | <0.01 |
iRVEDA, cm2/m2 | 10.2 (9.0–11.7) | 9 ± 2 | 0.10 |
Tricuspid regurgitation | |||
Mild | 12 (41) | 23 (74) | 0.02 |
Moderate | 15 (52) | 8 (26) | 0.03 |
Severe | 2 (7) | 0 (0) | NS |
RVP (N = 28) | LBBP (N = 30) | ||
LVGLS, % | 14 ± 4*,** | 18 ± 2 | <0.01 |
. | RVP . | LBBP . | P-value . |
---|---|---|---|
Baseline | N = 29 | N = 31 | |
Septal thickness, mm | 12 ± 3 | 11 ± 2 | 0.10 |
LV posterior wall thickness, mm | 11 ± 2 | 10 ± 2 | 0.24 |
LV mass index, g/m2 | 113 ± 32 | 105 ± 28 | 0.29 |
iLVEDV, mL/m2 | 57 ± 14 | 54 ± 11 | 0.40 |
iLVESV, mL/m2 | 20 ± 7 | 19 ± 6 | 0.85 |
LVEF, % | 65 ± 7 | 65 ± 6 | 0.71 |
LVGLS, % | 19 ± 4 | 20 ± 3 | 0.61 |
iRVEDA, cm2/m2 | 11 ± 3 | 10 ± 2 | 0.15 |
Tricuspid regurgitation | 0.50 | ||
Mild | 24 (83) | 28 (90) | |
Moderate | 4 (14) | 3 (10) | |
Severe | 1 (3) | 0 (0) | |
Post-implantation | N = 29 | N = 26 | |
iLVEDV, mL/m2 | 54 ± 12 | 49 ± 12* | 0.12 |
iLVESV, mL/m2 | 21 ± 6 | 18 ± 7 | 0.17 |
LVEF, % | 62 ± 7 | 64 ± 7 | 0.35 |
iRVEDA, cm2/m2 | 9.6 (8.8–11.8) | 9 ± 2 | 0.11 |
Tricuspid regurgitation | 0.45 | ||
Mild | 21 (72) | 24 (92) | |
Moderate | 7 (24) | 2 (8) | |
Severe | 1 (3) | 0 (0) | |
N = 28 | N = 26 | ||
LVGLS, % | 17 ± 4* | 19 ± 4 | 0.03 |
At last follow-up | N = 29 | N = 31 | |
iLVEDV, mL/m2 | 58 ± 15 | 48 ± 11* | <0.01 |
iLVESV, mL/m2 | 25 ± 9*,** | 17 ± 7* | <0.01 |
LVEF, % | 58 ± 8*,** | 65 ± 7 | <0.01 |
iRVEDA, cm2/m2 | 10.2 (9.0–11.7) | 9 ± 2 | 0.10 |
Tricuspid regurgitation | |||
Mild | 12 (41) | 23 (74) | 0.02 |
Moderate | 15 (52) | 8 (26) | 0.03 |
Severe | 2 (7) | 0 (0) | NS |
RVP (N = 28) | LBBP (N = 30) | ||
LVGLS, % | 14 ± 4*,** | 18 ± 2 | <0.01 |
Values are mean ± SD, n (%), or median (inter-quartile range).
*P < 0.05 vs. baseline; **P < 0.05 vs. post-implantation.
EDA, end-diastolic area; EDV, end-diastolic volume; EF, ejection fraction; ESV, end-systolic volume; GLS, longitudinal global strain; i, indexed to body surface area; LBBP, left bundle branch pacing; LV, left ventricular; NS, not significant; RV, right ventricular; RVP, right ventricular pacing.
LV function and MW
In univariate analysis, ΔLW–SW work difference inversely correlated with ΔLVEF and ΔLVGLS (r = −0.63, −0.42, respectively, both P < 0.01) and positively related to ΔiLVESV (r = 0.56, P < 0.01) during follow-up (see Supplementary data online, Figure S5). Furthermore, multivariate analysis revealed that ΔLW–SW work difference was an independent contributor to LV adverse remodelling (ΔiLVESV: r = 0.42 P < 0.01) and LV dysfunction (ΔLVEF: r = −0.61, P < 0.01 and ΔLVGLS: r = −0.38, P = 0.02) after adjusting for valvular disease and pacing mode, indicating more adverse LV remodelling and greater LVEF or LVGLS decrease with larger increase in LW–SW work difference.
RV size and TR
Changes in RV size and TR are shown in Table 1 and Supplementary data online, Figure S6. No significant change in iRVEDA was observed in either group over time. During follow-up, TR worsened in 13 (44.8%) RVP patients and 5 (16.1%) LBBP patients (P = 0.015). TR developed from mild to moderate in 17 of 18 patients, and moderate to severe in 1 patient who received RVP.
Discussion
In this two-centre study, we investigated LV mechanical dyssynchrony and LV global function in bradycardia patients undergoing LBBP compared with RVP. The main findings were as follows: (i) LBBP causes less mechanical dyssynchrony than RVP shortly after implantation and during 1-year follow-up and (ii) LBBP retains stable LVEF and LVGLS, while RVP leads to a decrease of LV function during follow-up.
LBBP and electrical dyssynchrony
The LBB is a continuation of the main branch of the His bundle, which divides into left anterior and posterior fascicles.28 LBBP is therefore likely to be a physiological pacing modality, since the paced ventricular activation is mediated by the intrinsic conduction system, resulting in a simultaneous depolarization of the LV with a narrow paced QRS.17–19,21 In contrast, traditional RVP does not utilize the natural conduction system. The abnormal electrical activation starts from the septum or apex and spreads through non-specific myocardium, causing a significantly delayed involvement of the LV free wall and a prolonged QRS duration.2,5 Accordingly, we measured a much shorter paced QRS duration in LBBP compared with RVP, indicating a better electrical synchrony with LBBP, which is consistent with previous findings.17–20
LBBP and mechanical dyssynchrony
Previous studies demonstrated that abnormal electrical activation due to RVP leads to a dyssynchronous ventricular contraction, which is comparable with typical activation patterns in LBBB.7,29 This RVP-induced mechanical dyssynchrony is characterized by a rapid contraction of the early-activated septum against very low load, which wastes part of the regional work through pre-stretching of the late-activated LW. The delayed segments, on the other hand, have to bear the entire stroke work.5,29 Our data suggest that MW was able to characterize and quantify the asymmetry in workload between LW and septum during pacing. Septal work was significantly impaired relative to LW work in the RVP group, resulting in large LW–SW work differences. On the contrary, the LBBP group exhibited a stable septal work and thus a much lower LW–SW work difference. To our knowledge, the present study is the first to demonstrate that, compared with RVP, LBBP better preserves a homogeneous MW distribution in patients with anti-bradycardia pacing. This may be explained by the fact that in LBBP, capturing the LBB creates a ventricular activation that is very similar to normal conduction and, thus, avoids significant mechanical dyssynchrony.29 Furthermore, the LW–SW work difference in LBBP was not significantly increased during the study period, demonstrating the capability of LBBP in preserving mechanical synchrony during mid-long-term follow-up.
SF and ApRock are well-known parameters that provide visual assessment of mechanical dyssynchrony.12,16 Also, speckle tracking-based strain patterns are an alternative method to characterize LV dyssynchrony.30 The significant positive correlations between these parameters and LW–SW work difference supported the hypothesis that all of them reflect the same phenomenon.
Although LBBP patients exhibited much less mechanical dyssynchrony than patients with RVP, some of them still showed slight SF and/or ApRock during follow-up. We noticed that such mild LV mechanical dyssynchrony occurred acutely after pacing. Possible explanations may be that non-selective LBBP (nsLBBP) is almost always present at working output (3.5 V/0.4 ms),31–33 i.e. both the LBB and adjacent local septal myocardium were captured. With nsLBBP, the directly activated local septal myocardium might contribute to the mild mechanical dyssynchrony. Furthermore, the capture of the pre-divisional LBB with simultaneous activation of all of its fascicles was not always possible due to the substantial variability in LBB anatomy.34 Therefore, many patients only achieved the capture of one of the LBB fascicles, which might lead to a slight delay in LV activation and consequently mechanical dyssynchrony. It is noteworthy that, although LV mechanical dyssynchrony remained unchanged in most patients during the study period, two patients exhibited progression of LV mechanical dysynchrony in terms of more advanced strain patterns and higher LW–SW work difference at 6-month follow-up. The possibility of a progressive conduction delay in these patients was excluded by a regular check of the ECG with pacing off. The QRS morphology on surface ECG with pacing showed LV septal pacing with prolongation in QRS duration and Stim-LVAT, indicating that the LBB might no longer be captured. This was likely to have occurred due to the fact that the LBB capture threshold had increased during follow-up as gradual development of scarring around the tip of the pacing lead,32 which is a known phenomenon35,36 even if pacing tips are coated with steroids, or possible micro dislodgement of the lead, which was not readily detectable by visual assessment. However, in the current study, histological data were not available to test this hypothesis.
LBBP and LV function
We observed a significant acute decrease of LVEDV in LBBP, which is in agreement with the results from Cai et al.17 One-year follow-up data demonstrated stable LVEF in LBBP compared with reduced LVEF in RVP, consistent with findings from previous studies.37,38 Similar changes were also noted for LVGLS. Furthermore, we found an independent association between ΔLW–SW work difference and ΔiLVESV, ΔLVEF, and ΔLVGLS, indicating that preserved synchronicity by LBBP can lead to preserved LV volumes and function over time and confirming that progressive mechanical dyssynchrony induced by RVP triggers LV adverse remodelling and dysfunction.8,39
Clinical implications
The present study demonstrates that the impact of LBBP on mechanical dyssynchrony is smaller compared with RVP by causing less asymmetry in LW–SW work distribution, providing further evidence of the potential superiority of LBBP to RVP. Despite the advantages of LBBP over RVP at implant, there is still possibility that LBB might not be permanently captured during follow-up. Assessing the magnitude of work asymmetry could be useful in the long-term surveillance of patients with LBBP to optimize therapy delivery.
MW difference is measured by acquisition of the apical views, which is feasible in nearly all patients. It offers a quantitative approach for the assessment of pacing-induced mechanical dyssynchrony, which was shown to be closely correlated with LV adverse remodelling and dysfunction. The results in the present study provide early mechanistic data to further understand the mechanical effect of protective pacing strategies associated with LBBP.
Study limitations
The present study should be interpreted in light of several methodologic limitations. First, this was a non-randomized, observational study involving a limited number of participants from two centres. Patients underwent LBBP or RVP on the basis of individual choice and clinical practice of the operators. Some baseline characteristics are not perfectly matched between two groups due to the lack of randomization, especially ethnicities (one group being mostly Asian-Chinese and one European-Belgian). However, after indexation of parameters and controlling for important baseline covariates (i.e. valvular heart disease, pacing mode, etc.), the difference between two groups remained significant in terms of LV mechanical dyssynchrony, remodelling, and function.
Furthermore, the present study provided data on mechanical dyssynchrony with the longest follow-up so far and reported changes over time, which have not been thoroughly investigated before. Nevertheless, it can be assumed that a longer follow-up period could have led to a more pronounced remodelling and clearer changes in cardiac function.
Additionally, the use of brachial cuff pressure for work analysis in patients with AF might be problematic as the LV pressure varies substantially from beat to beat. However, as blood pressure is a scaling factor in the work calculation that affects all walls similarly, the characteristic differences between walls remain preserved. Long-term, multi-centred, and randomized trials are warranted to confirm the mechanical benefits of LBBP in bradycardia patients indicated for a pacemaker implantation.
Conclusion
LBBP is associated with less LV mechanical dyssynchrony compared with RVP. During 1-year follow-up, LBBP appears to maintain LV function better than RVP.
Supplementary data
Supplementary data are available at European Heart Journal - Cardiovascular Imaging online.
Acknowledgements
The authors thank Dr Qin Fei and Dr Dan Xu for their valuable contributions to the study.
Funding
J.D. is supported by a Research Foundation Flanders (FWO) project grant (T002919N) and a FWO post-doc grant (12ZZN22N). J.-U.V. is supported as a senior clinical investigator by the FWO (1832917N). Y.Y. is supported by Medical Health Science and Technology Program of Zhejiang Province (2020KY165) and by Natural Science Foundation of Zhejiang Province (LY21H020006). M.F.P. is supported by a National Institute for Health Research Clinical Lectureship (NIHR31225).
Data availability
The datasets used and/or analysed during the current study are available from the corresponding author upon reasonable request.
References
Author notes
Yankai Mao, Jürgen Duchenne and Yuan Yang shared the first authorship.
Guosheng Fu and Jens-Uwe Voigt shared the senior authorship.
Conflict of interests: None declared.