Abstract
Cardiovascular magnetic resonance (CMR) imaging is recommended in patients with congenital heart disease (CHD) in clinical practice guidelines as the imaging standard for a large variety of diseases. As CMR is evolving, novel techniques are becoming available. Some of them are already used clinically, whereas others still need further evaluation. In this statement, the authors give an overview of relevant new CMR techniques for the assessment of CHD. Studies with reference values for these new techniques are listed in the Supplementary data online, supplement.
Introduction
Cardiovascular magnetic resonance (CMR) imaging is a gold-standard technique to assess children and adults with congenital heart disease (CHD), and numerous guidelines for CMR use in these patients have been published.1–4 In addition to technical and practical aspects, these documents emphasize the clinical importance of CMR to image these patients.
Several newer CMR techniques and applications have recently been developed such as lymphatic imaging, diffusion tensor imaging (DTI), and virtual and augmented reality; however, they are not yet part of routine clinical practice.5–7 Other methods, including four-dimensional (4D) phase-contrast imaging (4D flow), strain analysis with tissue tracking, and myocardial tissue characterization using parametric mapping techniques, are now widely applied, and a larger number of studies have shown a clinical impact. More recent guidelines include these techniques into their CMR reporting recommendations.2,4,8–10 However, CHD-specific expert statements focusing on the benefit and clinical use of novel CMR technologies are currently not available.
The purpose of this manuscript was therefore to focus on novel CMR methods and their application and potential impact on outcome and clinical decision-making in children and adults with CHD (Figure 1, Table 1). As normative data are essential for the clinical application of these techniques, this manuscript provides an overview of available reference values.

Figure 1
Summary of novel CMR technologies.
Table 1Advantages, disadvantages, availability, and applications of novel technologies in CHD
Technology
. | Advantages
. | Disadvantages
. | Availability
. | Applications in CHD
. |
---|
4D Flow | Planning the acquisition volume is easy Multi-dimensional analysis of blood flow Quantification of multiple haemodynamic markers Commercial analysis software is widely available
| Can be time-consuming | Widely available | Can be applied to every patient with CHD for blood flow assessment (e.g. stroke volumes, regurgitant volumes, Qp/Qs) |
Mapping techniques | | | Widely available | Can be applied to every patient with CHD with suspicion of diffuse myocardial changes |
3D cine | | | Not everywhere available | Can be applied to every patient with CHD |
Diffusion tensor imaging | | | Not everywhere available | Not ready for routine clinical use |
Lymphatic imaging | | | Widely available | Especially used in single-ventricle patients on the Fontan pathway or with an established Fontan circulation |
Foetal MRI | | Might be challenging due to foetal size and movement, high foetal heart rates as well as to difficulties in cardiac gating Largely limited to the third trimester
| | Can be used to assess foetal cardiovascular anatomy and haemodynamics. Assessment of foetal interventions might be possible. |
Stress MRI | | Possible side effects of pharmacological stressors Some pharmacological stressors are not approved for children Physical exercise is not possible in every patient (e.g. small children)
| | In patients with suspected coronary artery pathology in the setting of CHD or acquired paediatric heart disease (e.g. transposition of the great arteries, Kawasaki syndrome) |
Catheter MRI | | Can be time-consuming MRI-approved catheters and other MR conditional materials are still limited MRI conditional haemodynamic measurement systems, screens for visualization, and communication systems are normally needed
| Not everywhere available | Pulmonary vascular resistance measurements are well approved in patients with a Fontan circulation or with pulmonary hypertension |
Computational fluid dynamics | | | Not everywhere available | CFD has been mainly applied in assessing Fontan connections and in patients with aortic diseases. Other applications are possible. |
Deep learning | | | Not everywhere available | Segmentation of 2D and 3D image data sets (e. g. volumetric measurements) |
Strain imaging | Can be applied to routinely acquired cine images Commercial analysis software is widely available Analysis times are short Provides additional functional information Might be able to detect early ventricular dysfunction
| Strain values might not be interchangeable between vendors Lower spatial and temporal resolutions compared with speckle tracking echocardiography Segmental strain values are less reliable
| Widely available | Can be applied to every patient with CHD, especially in the context of ventricular dysfunction. |
3D printing | | High-resolution 3D data set must be acquired Segmentation software is needed Segmentation can be time-consuming
| Not everywhere available | To plan surgical procedures in complex CHD. To plan complex interventions in CHD. To teach students or physicians.
|
Virtual/augmented reality | | High-resolution 3D data set must be acquired Segmentation software is needed Segmentation can be time-consuming Software and head-mounted displays are needed
| Not everywhere available | To plan surgical procedures in complex CHD. To plan complex interventions in CHD. To teach students or physicians.
|
Technology
. | Advantages
. | Disadvantages
. | Availability
. | Applications in CHD
. |
---|
4D Flow | Planning the acquisition volume is easy Multi-dimensional analysis of blood flow Quantification of multiple haemodynamic markers Commercial analysis software is widely available
| Can be time-consuming | Widely available | Can be applied to every patient with CHD for blood flow assessment (e.g. stroke volumes, regurgitant volumes, Qp/Qs) |
Mapping techniques | | | Widely available | Can be applied to every patient with CHD with suspicion of diffuse myocardial changes |
3D cine | | | Not everywhere available | Can be applied to every patient with CHD |
Diffusion tensor imaging | | | Not everywhere available | Not ready for routine clinical use |
Lymphatic imaging | | | Widely available | Especially used in single-ventricle patients on the Fontan pathway or with an established Fontan circulation |
Foetal MRI | | Might be challenging due to foetal size and movement, high foetal heart rates as well as to difficulties in cardiac gating Largely limited to the third trimester
| | Can be used to assess foetal cardiovascular anatomy and haemodynamics. Assessment of foetal interventions might be possible. |
Stress MRI | | Possible side effects of pharmacological stressors Some pharmacological stressors are not approved for children Physical exercise is not possible in every patient (e.g. small children)
| | In patients with suspected coronary artery pathology in the setting of CHD or acquired paediatric heart disease (e.g. transposition of the great arteries, Kawasaki syndrome) |
Catheter MRI | | Can be time-consuming MRI-approved catheters and other MR conditional materials are still limited MRI conditional haemodynamic measurement systems, screens for visualization, and communication systems are normally needed
| Not everywhere available | Pulmonary vascular resistance measurements are well approved in patients with a Fontan circulation or with pulmonary hypertension |
Computational fluid dynamics | | | Not everywhere available | CFD has been mainly applied in assessing Fontan connections and in patients with aortic diseases. Other applications are possible. |
Deep learning | | | Not everywhere available | Segmentation of 2D and 3D image data sets (e. g. volumetric measurements) |
Strain imaging | Can be applied to routinely acquired cine images Commercial analysis software is widely available Analysis times are short Provides additional functional information Might be able to detect early ventricular dysfunction
| Strain values might not be interchangeable between vendors Lower spatial and temporal resolutions compared with speckle tracking echocardiography Segmental strain values are less reliable
| Widely available | Can be applied to every patient with CHD, especially in the context of ventricular dysfunction. |
3D printing | | High-resolution 3D data set must be acquired Segmentation software is needed Segmentation can be time-consuming
| Not everywhere available | To plan surgical procedures in complex CHD. To plan complex interventions in CHD. To teach students or physicians.
|
Virtual/augmented reality | | High-resolution 3D data set must be acquired Segmentation software is needed Segmentation can be time-consuming Software and head-mounted displays are needed
| Not everywhere available | To plan surgical procedures in complex CHD. To plan complex interventions in CHD. To teach students or physicians.
|
Table 1Advantages, disadvantages, availability, and applications of novel technologies in CHD
Technology
. | Advantages
. | Disadvantages
. | Availability
. | Applications in CHD
. |
---|
4D Flow | Planning the acquisition volume is easy Multi-dimensional analysis of blood flow Quantification of multiple haemodynamic markers Commercial analysis software is widely available
| Can be time-consuming | Widely available | Can be applied to every patient with CHD for blood flow assessment (e.g. stroke volumes, regurgitant volumes, Qp/Qs) |
Mapping techniques | | | Widely available | Can be applied to every patient with CHD with suspicion of diffuse myocardial changes |
3D cine | | | Not everywhere available | Can be applied to every patient with CHD |
Diffusion tensor imaging | | | Not everywhere available | Not ready for routine clinical use |
Lymphatic imaging | | | Widely available | Especially used in single-ventricle patients on the Fontan pathway or with an established Fontan circulation |
Foetal MRI | | Might be challenging due to foetal size and movement, high foetal heart rates as well as to difficulties in cardiac gating Largely limited to the third trimester
| | Can be used to assess foetal cardiovascular anatomy and haemodynamics. Assessment of foetal interventions might be possible. |
Stress MRI | | Possible side effects of pharmacological stressors Some pharmacological stressors are not approved for children Physical exercise is not possible in every patient (e.g. small children)
| | In patients with suspected coronary artery pathology in the setting of CHD or acquired paediatric heart disease (e.g. transposition of the great arteries, Kawasaki syndrome) |
Catheter MRI | | Can be time-consuming MRI-approved catheters and other MR conditional materials are still limited MRI conditional haemodynamic measurement systems, screens for visualization, and communication systems are normally needed
| Not everywhere available | Pulmonary vascular resistance measurements are well approved in patients with a Fontan circulation or with pulmonary hypertension |
Computational fluid dynamics | | | Not everywhere available | CFD has been mainly applied in assessing Fontan connections and in patients with aortic diseases. Other applications are possible. |
Deep learning | | | Not everywhere available | Segmentation of 2D and 3D image data sets (e. g. volumetric measurements) |
Strain imaging | Can be applied to routinely acquired cine images Commercial analysis software is widely available Analysis times are short Provides additional functional information Might be able to detect early ventricular dysfunction
| Strain values might not be interchangeable between vendors Lower spatial and temporal resolutions compared with speckle tracking echocardiography Segmental strain values are less reliable
| Widely available | Can be applied to every patient with CHD, especially in the context of ventricular dysfunction. |
3D printing | | High-resolution 3D data set must be acquired Segmentation software is needed Segmentation can be time-consuming
| Not everywhere available | To plan surgical procedures in complex CHD. To plan complex interventions in CHD. To teach students or physicians.
|
Virtual/augmented reality | | High-resolution 3D data set must be acquired Segmentation software is needed Segmentation can be time-consuming Software and head-mounted displays are needed
| Not everywhere available | To plan surgical procedures in complex CHD. To plan complex interventions in CHD. To teach students or physicians.
|
Technology
. | Advantages
. | Disadvantages
. | Availability
. | Applications in CHD
. |
---|
4D Flow | Planning the acquisition volume is easy Multi-dimensional analysis of blood flow Quantification of multiple haemodynamic markers Commercial analysis software is widely available
| Can be time-consuming | Widely available | Can be applied to every patient with CHD for blood flow assessment (e.g. stroke volumes, regurgitant volumes, Qp/Qs) |
Mapping techniques | | | Widely available | Can be applied to every patient with CHD with suspicion of diffuse myocardial changes |
3D cine | | | Not everywhere available | Can be applied to every patient with CHD |
Diffusion tensor imaging | | | Not everywhere available | Not ready for routine clinical use |
Lymphatic imaging | | | Widely available | Especially used in single-ventricle patients on the Fontan pathway or with an established Fontan circulation |
Foetal MRI | | Might be challenging due to foetal size and movement, high foetal heart rates as well as to difficulties in cardiac gating Largely limited to the third trimester
| | Can be used to assess foetal cardiovascular anatomy and haemodynamics. Assessment of foetal interventions might be possible. |
Stress MRI | | Possible side effects of pharmacological stressors Some pharmacological stressors are not approved for children Physical exercise is not possible in every patient (e.g. small children)
| | In patients with suspected coronary artery pathology in the setting of CHD or acquired paediatric heart disease (e.g. transposition of the great arteries, Kawasaki syndrome) |
Catheter MRI | | Can be time-consuming MRI-approved catheters and other MR conditional materials are still limited MRI conditional haemodynamic measurement systems, screens for visualization, and communication systems are normally needed
| Not everywhere available | Pulmonary vascular resistance measurements are well approved in patients with a Fontan circulation or with pulmonary hypertension |
Computational fluid dynamics | | | Not everywhere available | CFD has been mainly applied in assessing Fontan connections and in patients with aortic diseases. Other applications are possible. |
Deep learning | | | Not everywhere available | Segmentation of 2D and 3D image data sets (e. g. volumetric measurements) |
Strain imaging | Can be applied to routinely acquired cine images Commercial analysis software is widely available Analysis times are short Provides additional functional information Might be able to detect early ventricular dysfunction
| Strain values might not be interchangeable between vendors Lower spatial and temporal resolutions compared with speckle tracking echocardiography Segmental strain values are less reliable
| Widely available | Can be applied to every patient with CHD, especially in the context of ventricular dysfunction. |
3D printing | | High-resolution 3D data set must be acquired Segmentation software is needed Segmentation can be time-consuming
| Not everywhere available | To plan surgical procedures in complex CHD. To plan complex interventions in CHD. To teach students or physicians.
|
Virtual/augmented reality | | High-resolution 3D data set must be acquired Segmentation software is needed Segmentation can be time-consuming Software and head-mounted displays are needed
| Not everywhere available | To plan surgical procedures in complex CHD. To plan complex interventions in CHD. To teach students or physicians.
|
Practical and technical considerations
Considerations on field strength for CMR
Magnetic resonance imaging (MRI) systems with a field strength of 1.5 T are currently most commonly used for the clinical assessment of cardiovascular diseases. MRI systems at 3.0 T offer some advantages in patients with CHD due to a higher signal-to-noise ratio (SNR) which can be traded off to improve spatial and temporal resolution11,12 but also suffer from higher sensitivity to B0 inhomogeneity and B1 non-uniformity.
For the best results on sequences with fast gradient switching such as balanced steady-state free precession or 4D flow MRI, a gradient system with high strength (≥45 mT/m) and slew rate (≥200 T/m/s) is beneficial. Moreover, ultra-high-field CMR (≥7T) has potential for certain clinical applications but is not commonly used yet.13–16 It provides submillimetre resolution to image the myocardium but has been difficult to explore11 due to the need of parallel (multi) transmit MRI. Advantages of the theoretically linear relationship between SNR and field strength are limited in practice by increased native T1 which may require longer repetition time and thereby increase the acquisition time, augmented patient-specific absorption rate limiting the maximum flip angle, and artefacts associated with the magnetic susceptibility effects.11,17
In recent years, low-field MRI systems operating at field strengths of 1.0 T or below equipped with high-performance gradients have gained increasing attention because of their potential advantages that include a reduction in artefacts, safety benefits for patients with implanted devices, feasibility of MRI-guided cardiac catheterization using standard guidewires and devices, and hopefully increased global access to good quality CMR imaging due to lower costs.18–20 In addition, low-field systems might be able to decrease greenhouse gas emissions because they are less energy-intensive.21 Research and development on this topic are ongoing. Both ultra-high-field MRI B0 ≥ 7.0 T and low-field MRT B0 < 1.0 T have not yet been widely implemented into routine clinical use so far.
Common CMR sequences—and their application
4D Flow
Analysing blood flow adds valuable information to the assessment of cardiovascular disease. Traditional two-dimensional (2D) phase-contrast CMR requires careful planning of planes perpendicular to the vessel of interest.22–24 In cases of complex CHD, precise knowledge of patient anatomy is paramount and planning of individual 2D phase-contrast flow imaging acquisitions relies on an exact planning of the imaging plane during the scan. Acquisition times of 4D flow CMR are currently in the range of 10 min or less, the volume of interest can be placed over the entire thorax, and data can be retrospectively interrogated.10,25–27 Novel acceleration techniques such as compressed sensing (CS) are able to reduce scan time further and may improve patient comfort and the need for general anaesthesia or sedation in smaller children.28
Moreover, 4D flow allows to achieve multi-dimensional interactive intra- and extra-cardiac qualitative analysis of flow features using colour-coded stream or path lines as well as quantification of haemodynamic biomarkers such as peak velocity, regurgitant fraction, wall shear stress, helicity, vorticity, and (turbulent) kinetic energy10,29 (Figures 2 and 3).
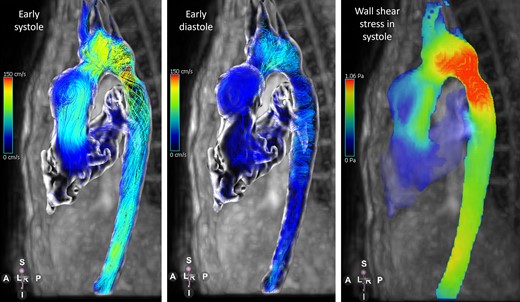
Figure 2
Flow patterns using path lines and wall shear stress derived from 4D flow CMR in a patient with hypoplastic left heart syndrome after Norwood procedure.
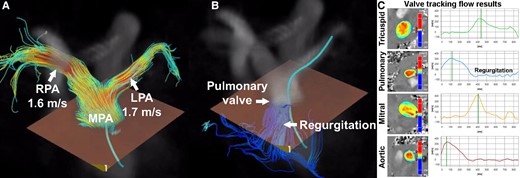
Figure 3
Whole-heart 4D flow CMR of a 14-year-old male after arterial switch operation with the Lecompte manoeuver. Narrowing of the pulmonary arteries is a typical complication of this operation. Assessment of branch pulmonary by echocardiography was inconclusive. Using 4D flow CMR, a peak velocity in the left pulmonary artery of 1.7 m/s and in the right pulmonary artery of 1.6 m/s was measured (A), suggesting mild narrowing of both arteries. Furthermore, assessment of pulmonary valve regurgitation (B, C) and flow assessment of aortic as well as atrioventricular valves (C) was possible. With 2D flow CMR imaging, it would have been more difficult to accurately measure branch pulmonary artery peak velocities because visualization of the area with the highest velocity is more difficult. Courtesy of Dr Joost van Schuppen (Department of Radiology and Nuclear Medicine, Amsterdam University Medical Center).
Commercially available software allows measurement of standard flow parameters and analysis of flow patterns and provides advanced wall shear stress quantification.30 Detailed assessment of CHD including quantification of intracardiac shunts,31–34 caval to pulmonary venous collateral flow in single-ventricle palliation, valvular regurgitation and peak velocity35–41 evaluation in valve or vessel stenosis, pulmonary hypertension, and aortic coarctation42–45 even in neonates46 is now feasible.
Image analysis might be, however, affected by sequence settings, and care must be taken when applying recommendations derived from adult patients to the paediatric population.47 The suggested acquired voxel size of 2.5 mm × 2.5 mm × 2.5 mm should be adjusted according to the paediatric patient size currently down to a limit of 1 mm × 1 mm × 1 mm at the cost of signal loss.10 Scan times can be reduced by a combination of advanced reconstruction methods and more efficient k-space sampling trajectories.48
Tissue characterization
Myocardial tissue characterization is a key determinant of outcomes in patients with paediatric and/or CHD.49 One of the greatest assets of CMR is the ability to detect and quantify tissue changes within the myocardium (such as fibrosis, scarring, oedema, fat, and iron deposition). Fibrosis and scar imaging are based on the intrinsic T1 relaxation properties of fibrosed myocardium (native T1 times) and/or on the retention of gadolinium [late gadolinium enhancement (LGE) and extracellular volume (ECV)]9 (Figure 4). Contrast agent administration in this context creates increased signal that can be exchanged for higher spatial resolution or acceleration. Controversy about the development of nephrogenic systemic fibrosis and accumulation in brain and bone tissue has reduced the enthusiasm for the use of gadolinium-based contrast agent in children. Nevertheless, studies have shown the safety of gadolinium-based contrast application and no adverse health effects have been confirmed to be associated with gadolinium presence in the brain.50 However, contrast-enhanced imaging is not needed in every CMR study in patients with CHD and further developments such as synthetic LGE imaging might be able to completely avoid contrast applications.51,52 Other novel technologies such as free-breathing three-dimensional (3D) LGE imaging and CMR fingerprinting can improve scar detection and diffuse myocardial changes.53,54
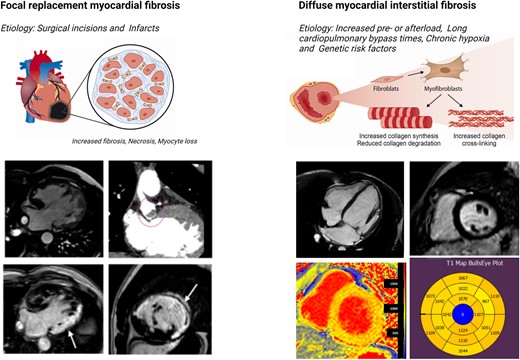
Figure 4
Focal myocardial replacement fibrosis, myocardial necrosis, and myocyte loss are commonly associated with surgical incisions and infarcts. An example of this is seen in a patient with hypoplastic left heart syndrome and a large infarct, indicated by arrows, along with a thrombus in the Damus–Kaye–Stansel anastomosis shown by a red circle. Diffuse myocardial fibrosis can be evaluated by parametric mapping in patients with increased pre- or afterload, long cardiopulmonary bypass times, chronic hypoxia, or genetic risk factors. An example of this is seen in a patient with moderate mitral regurgitation. The short-axis T1 mapping revealed significant cardiac remodelling and diffuse fibrosis prior to the development of symptoms. Late gadolinium enhancement was not detected, as its detection relies on the spatial heterogeneity of fibrosis, which was not present in the non-infarcted myocardium of this patient.
In tetralogy of Fallot (ToF), LGE extent is associated with diminished biventricular ejection fraction (EF), right ventricular dilation, poor functional class and exercise intolerance, and mortality.55–57 ToF is the only CHD to date in which LGE has been shown to independently predict mortality during follow-up.58 In the same study, LGE predicted life-threatening ventricular arrhythmia [not including appropriate implantable cardioverter defibrillator shock, ventricular ectopy, or non-sustained ventricular tachycardia (VT)].58 In patients with a biventricular circulation and a systemic right ventricle, LGE is a harbinger of arrhythmias, impaired exercise performance, heart failure, transplantation, and death.59–61 The presence of LGE in Fontan patients is associated with non-sustained VT.62 In contrast to LGE, which detects patches of replacement fibrosis of a certain size, T1 parametric mapping, with its quantitative metrics of native T1 and ECV fraction, aims at detecting and scaling diffuse fibrotic processes, which might be more prevalent in the current surgical era with better perioperative myocardial protection than replacement fibrosis.56 T1 times and/or ECVs are elevated in patients with CHD with abnormal cardiac pre- or afterload, longer cardiopulmonary bypass times, chronic hypoxia, and genetic factors.57,63–65 In ToF, prolonged T1 or expanded ECVs are associated with abnormal strain and ventricular arrhythmias (mainly comprising frequent ectopics or non-sustained VT), hospital admission, or death.49,57,66 In Fontan patients, they are linked to a composite endpoint of hospital admission, reintervention, Fontan failure, and arrhythmias.67
CMR tissue characterization, encompassing T2-weighted imaging, LGE, and T1 and T2 times, has been used as a virtual biopsy, decreasing the need for invasive tissue sampling, for example, during organ rejection following heart transplantation or in suspected myocarditis.68–71 An increase in T2 values allows increasing accuracy in the diagnosis of myocarditis in the chronic state (beyond 14 days). In addition, the presence of LGE, beyond 6 months, is an important predictor of cardiovascular events at follow-up.72,73
However, more longitudinal outcome-based studies are needed to demonstrate the ability of CMR tissue characterization to aid in the risk stratification for individual patients. Future directions include myocardial viability and fibrosis imaging without the use of gadolinium-based contrast as well as magnetic resonance fingerprinting.74 The latter provides T1 and T2 times in a single sequence and yields metrics on tissue perfusion, diffusion, fat fraction, and T2*.
3D Cine (structural imaging)
Time-resolved cine imaging provides good contrast between the myocardium and the blood pool. 2D cine imaging is commonly used for quantitative ventricular volumetry and function as well as myocardial mass assessment. As such, short-axis cine stacks are the cornerstone of quantitative CMR examinations.4 Disadvantages of a 2D cine approach include the frequent need for consecutive breath-holds, which may be difficult for non-sedated children and thick slices that may lack anatomical detail. Consecutive slices may be misaligned due to patient movement between acquisitions. Isotropic, fast, free-breathing, time-resolved 3D cine sequences are desirable as they allow reformatting any desired plane within a 3D volume75,76 (Figure 5). Contrast agent administration in this context creates increased signal that can be exchanged for higher spatial resolution or acceleration. Controversy about the development of nephrogenic systemic fibrosis and accumulation in brain and bone tissue has reduced the enthusiasm for the use of gadolinium-based contrast agent in children.
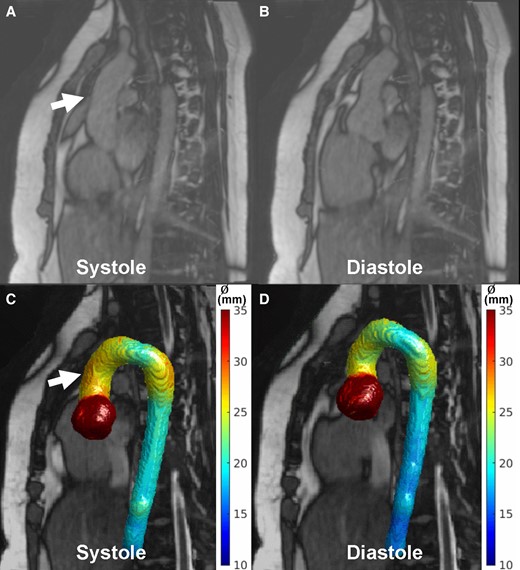
Figure 5
Systolic (A) and diastolic (B) images from an aortic 3D cine acquisition (pseudo-spiral undersampling, scan time ∼4 min, acquired/reconstructed spatial resolution 1.6/1.0(mm)3, temporal resolution ∼67 ms/15 cardiac phases, TR/TE/FA: 2.9 ms/1.44 ms/40°) in a 22-year-old female with the Marfan syndrome. With nnU-Net, 4D segmentations were created followed by 4D diameter calculation showing a larger diameter in the ascending aorta in systole (A, C, arrows) compared with diastole (B, D). Courtesy of Daan Bosshardt and Renske Merton (Department of Radiology and Nuclear Medicine, Amsterdam University Medical Center).
Administration of ferumoxytol, ultra-small superparamagnetic iron oxide nanoparticles with a long intravascular half-life and high relaxivity, provides good diagnostic image quality at both 1.5 and 3 T by shortening T1.77,78 Shorter scan and image reconstruction times can be achieved with parallel imaging or deep learning (DL) technology.79 New isotropic ultrafast 3D cine sequences require only one breath-hold and might be an advantage for smaller children, especially for those with abdominal breathing. Faster image acquisition enabled by novel MR image reconstruction techniques including motion-resolved or motion-corrected reconstruction,79–81 newer acceleration techniques82, and higher resolution imaging by exploiting contrast agents may shift the current clinical use of 2D to 3D cine MR imaging technology.
Diffusion tensor imaging
DTI has been widely applied in brain MRI research and more recently utilized in cardiology to gain insight into the microstructure of healthy and diseased myocardium. Diffusion tensor cardiovascular magnetic resonance (DT-CMR) is a non-invasive imaging method which interrogates the diffusion of water within the myocardial matrix. Diffusion is defined by a mathematical tensor which conveys information in three perpendicular dimensions known as eigenvectors. These measures have been shown to correspond with the arrangement of left ventricular (LV) cardiomyocytes83–85 and validated with histology. Advances in sequence development have enabled the use in vivo to assess beating hearts and begin to understand the relationship between structure and function.
DT-CMR has revealed abnormalities in LV myocardial microarchitecture in ischaemic, hypertrophic, dilated, and infiltrative cardiomyopathies. Certain DT-CMR parameters have been shown to correspond with myocardial disarray and fibrosis.86 There has been limited application of DT-CMR within CHD and the RV in part due to the limits of its spatial resolution, abnormal, and challenging ventricular geometry. On the other side, patients with end-stage complex lesions are less able to tolerate currently lengthy scan times. However, the existing capability has provided insight into myocardial architecture in situs inversus totalis,87 and studies have investigated the cardiac microarchitecture in some CHD lesions in both foetal and adult heart specimens utilizing 3 and 9.4 T scanners.7,88,89 DT-CMR is not yet ready for routine clinical use. Making DT-CMR feasible for investigating myocardial microstructure in the thin-walled RV90 in a spectrum of patients including those with end-stage disease will require ongoing technical developments to improve spatial resolution, withstand arrhythmia, and reduce acquisition time. Future research is ongoing to find out whether DT-CMR assessment of microstructural function could improve prognostic assessment and better determine myocardial remodelling or heart failure and arrhythmia substrates.
Lymphatic imaging
Imaging of the lymphatic system with MRI has gained increasing interest with the growing number of Fontan patients and is currently recommend for its part of the surveillance testing protocol in children and adults with Fontan circulation.91,92 The lymphatic system is a critical component of the circulation, particularly in CHD93 with more treatment options for lymphatic intervention being available recently.6 Lymphatic abnormalities in patients post-single-ventricle surgical palliation were initially described using MRI by employing T2-weighted cardiac-gated and respiratory-navigated sequences.94 Dynamic contrast-enhanced MRI lymphangiography (MRL) permits detailed mapping of the central lymphatics by direct injection of MRI contrast into the inguinal lymph nodes.95 This has helped map sources of active lymphatic leak in plastic bronchitis96 and post-operative chylothorax97 with successful embolization of the leak or thoracic duct in selected cases. Over time, a better understanding of abnormal lymphatic patterns has been shown to improve treatment guidance and prognostication.98
Screening patients at risk of lymphatic abnormalities has been attempted, but T2 imaging alone is challenging. Combining T2-weighted imaging with 3D steady-state free precession (SSFP) or 3D mDixon imaging might improve delineation of lymphatic abnormalities99 (Figure 6). Patients who have a more serious lymphatic abnormality or leak may be selected to undergo percutaneous dynamic contrast-enhanced MRL. In addition to inguinal intranodal injections, intrahepatic MRL was introduced to map the hepatic lymphatics100 and is able to identify pathological abdominal lymphatic flow mediating protein-losing enteropathy101 that cannot be detected by central lymphatic assessment.
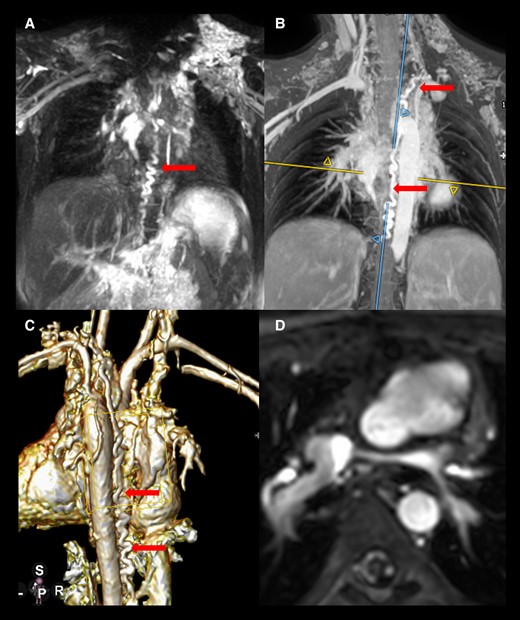
Figure 6
Static lymphatic imaging using a 3D gradient echo and spin echo and 3D modified Dixon technique in a 6-year-old girl with hypoplastic left heart syndrome and plastic bronchitis (A–C). She has a bilateral and bidirectional upper cavopulmonary connection. Both the left pulmonary artery and the connection between the right-sided hemi-Fontan and the left Glenn anastomosis are hypoplastic (D) possibly creating a drainage problem for the left-sided thoracic duct that is dilated and tortuous.
While the importance of lymphatic abnormalities in cardiac disease and CHD is increasingly recognized, reports on the current knowledge are now available for more holistic assessment combining imaging with other blood biomarkers and genetic testing.102 A collaborative effort involving cardiologists and interventional radiologists is advised to maximize the impact of this approach.
Foetal cardiac MRI
Prenatal detection of CHD has remained limited to ultrasound for many years. While foetal MRI has been widely available, application of CMR has been challenged by foetal size, motion, and the lack of cardiac gating. Developments in image acquisition and post-processing techniques including motion correction have enabled a rapid uptake in the application of foetal CMR at 1.5 and 3T, largely in the third trimester. Foetal CMR has a potential role in the evaluation of foetal cardiac anatomy and physiology in the context of the wider foetal circulation and extra-cardiac abnormalities. Non-gated approaches to 3D whole-heart imaging by means of image reconstruction of 2D images have been successful and permit refinement of clinical diagnosis,103,104 more so when combined with foetal flows utilizing metric optimized gating.105,106 Novel Doppler ultrasound devices107 have extended the use of gated MRI acquisitions using standard CMR acquisition techniques suitable for clinical interpretation.108 The addition of dynamic gated cine data and flow more recently109 permits the quantification of cardiac functional parameters akin to post-natal life. Developments including 4D flow110,111 and T2 mapping allow for more complete assessment of the foetal circulation112,113 which can be applied to assess foetal interventions such as maternal hyperoxygenation.114 Extension to scanners with a field strength of 0.55 T as seen in non-cardiac foetal applications115 with a wider bore size and improved maternal experience of the scan offer further promise to wider adoption.
Stress MRI
In CHD, stress CMR is an established, although not widely used, tool. Physical stress and pharmacological stress have been applied, mainly in CHD affecting the right ventricle and in the single-ventricle circulation, to determine global systolic function, contractile reserve, wall motion, and diastolic and vascular function and have proved their clinical value.116–139 In patients with suspected coronary artery pathology in the setting of CHD or acquired paediatric heart disease, stress CMR has important clinical applications. Typical indications are anomalous origins of the coronary arteries in patients who underwent coronary artery reimplantation (especially in transposition of the great arteries).3,140 Quantitative assessment of stress CMR studies has been shown to be feasible in children.141,142
Physical exercise is considered the optimal stressor of the cardiovascular system.143,144 With specific MR-compatible ergometers, supine exercise can be performed,144,145 although the effect of posture on central haemodynamics must be considered.146 Supine exercise protocols currently lack standardization.122,123,131–133,147–153 Oxygen uptake-driven protocols are the preferred method but have not found widespread application.148 Considering the limitations of physical exercise in the MR environment, image acquisition is mainly performed directly after cessation of exercise, which can be done either in or outside the scanner.120,122,123,133,154
Pharmacological agents can be used to overcome the limitations of scanning combined with physical exercise. Most stress imaging studies in patients with CHD have used pharmacological stress, mostly dobutamine. Various protocols with high-dose dobutamine (20 microgram/kg/min or more)136,155–161 and lower-dose dobutamine (5.0–20 μg/kg/min) stress have been used, with adequate patient safety and a significant cardiovascular stress response.116,117,129,130,136,144,155–162 For perfusion imaging, adenosine and (more recently) regadenoson have successfully been applied in infants and children.163 However, vasodilator stress testing has not proved its clinical value in the setting of anomalous coronary artery origin. Further clinical trials are needed to compare the diagnostic efficiency of adenosine vasodilator stress testing with dobutamine and CMR exercise stress testing in patients with abnormal coronary artery origin. Furthermore, differences in stroke volume and/or ventricular volumes should be weighed against practical differences.164–166 Dobutamine stress for instance has been associated with a larger increase in EF and decrease in end-systolic volume compared with physical stress.166
Cardiopulmonary exercise is often performed in patients with CHD and can provide highly important information on cardiovascular physiology and prognosis. The combination with stress CMR has been shown to be feasible, and if practical issues can be solved, this would be a highly powerful tool in the clinical practice.167 With improved fast real-time imaging and motion compensation, this might be feasible in near future.119 Alternatively, in-scanner exercise combined with real-time imaging can be used, combining ungated real-time CMR using highly accelerated sequences and retrospective synchronization of ECG and respiratory motion.119,131,132,168
Future work should pay attention to standardization of both dobutamine and physical stress levels during stress CMR, allowing better comparison of results of scientific and clinical applications of these methods.153
Catheter MRI
Hybrid CMR catheterization combining X-ray fluoroscopically guided catheter placements for invasive pressure measurements with CMR-derived haemodynamic parameters, such as phase-contrast flows, for the pulmonary vascular resistance measurement has previously been described.169,170 This has become largely superseded by pure MRI-guided catheterization dispensing with the need for X-ray fluoroscopy. This approach is now regarded as the gold standard for haemodynamic measurements, especially in CHD.171 Non-braided balloon-tipped angiographic catheters are typically used, with visualization of the catheter tip using either the susceptibility artefact from a CO2-filled balloon, or positive contrast from diluted gadolinium to aid navigation.172
This approach has been widely adopted in the management of patients with CHD and/or pulmonary hypertension using hybrid X-ray and MRI suites173 or standalone MRI scanners174 that have been augmented with MRI conditional haemodynamic measurement systems, screens for visualization, and communication systems. MRI safety is paramount in the set-up and should be embedded into the facility and clinical workflows.175 Commercially available guidewires have widened the use to include more technically challenging cases and haemodynamic assessments such as dynamic Fontan fenestration occlusion176 or assessment of stress haemodynamics.159,177 Advances in acceleration and real-time imaging further reduce procedure times for more clinically acceptable workflows.174 These developments can open up new possibilities for interventional MRI in CHD such as electrophysiology procedures178–181 and endovascular interventions.182,183 Applications combining invasive pressure measurements and stress CMR are not implemented in the clinical routine yet, mainly due to the lack of MRI compatible catheter equipment. These studies can enhance our understanding of ventricular physiology under stress conditions in patients with CHD.159,177
While diagnostic catheterization is more widely established, interventional procedures in the CMR environment remain limited.175,184,185 The challenges of MRI conditional hardware and adequate imaging capabilities of the entire length of the catheter or guidewire are being actively addressed by the scientific community and industry. Exploration of CMR catheterization at low field strength defined as systems in the range 0.25–1.0 T,18,186 also offers potential for expanding the use of standard guidewires and catheters in an MRI environment with potential for clinical adoption.
Intravascular contrast agents
Contrast agents exhibit fast longitudinal (and transverse) magnetization relaxation after an excitation pulse, thus increasing signal on T1 (and T2)-weighted acquisitions. Different scanning sequences take advantage of this property to acquire contrast-enhanced magnetic resonance angiography images, or for tissue characterization such as ECV or LGE. Some contrast agents, sometimes referred to as ‘intravascular’ or ‘blood pool’ agents, have a long intravascular half-life, thus avoiding bolus timing, and provide sufficient imaging time for 3D SSFP angiography,187,188 3D cine SSFP,189 4D multiphase, steady-state imaging with contrast enhancement (MUSIC)—an angiographic technique that acquires 3D volumetric data over multiple phases of the cardiac cycle,190 2D flow,191 or 4D flow.
Ferumoxytol has an intravascular half-life of ∼15 h and can be used in patients with reduced kidney function in all age groups as it is excreted through the liver. It can be used for contrast-enhanced magnetic resonance angiography192 and may provide greater accuracy for 4D flow-derived volumes in small children than gadolinium.193 Some utility for T2* mapping of the myocardium has been suggested,194 and tissue properties may be examined in the future based on iron retention in the reticuloendothelial system. Ferumoxytol is used off-label as an MRI contrast agent and has been associated with 2% adverse events.195 There are currently no available gadolinium-based intravascular agents.
Hyperpolarized isotopes for quantification of metabolites in the myocardium are subject of research in adults but are not part of clinical evaluations in children so far.
Novel post-processing methods
Computational fluid dynamics
Computational fluid dynamics (CFD) is a technique to simulate blood flow in finite-element meshes that represent vascular regions of interest (Figure 7). Accuracy of these simulations depends on geometry and flow, or pressure conditions prescribed on the boundaries of the mesh. Patient-specific geometries can be obtained from CT or high-resolution anatomical MRI. Flow profiles for boundary conditions are usually taken from the literature or from phase-contrast MRI. CFD allows the derivation of many haemodynamic and biomechanical parameters such as velocity, shear stress, energetics, pressure, wall displacement, and strain. Mesh optimization and calculations of the haemodynamic profiles can be extremely time-consuming in the order of 60 h.196 This makes clinical use of CFD challenging. Nonetheless, CFD in CHD can be used for evaluation of functionality of the connections in a Fontan circuit, for example, for predictions of surgical planning in terms of pulmonary and hepatic flow distribution196 or for the virtual testing of conduit haemodynamic performance at rest and during exercise.197 Additionally, CFD is suitable for the simulation of haemodynamics in patients with CHD pre- and post-repair of aortic coarctation at rest and during exercise.198,199 For certain applications, however, care should be taken to include fluid–structure interaction methodology, for example, for complex haemodynamics in patients with the Marfan syndrome.199 By using steady-state simulations and optimizing meshes, the long CFD calculation times have been reduced for the Fontan to a few minutes, bringing CFD a step closer to application in clinical workflows.200
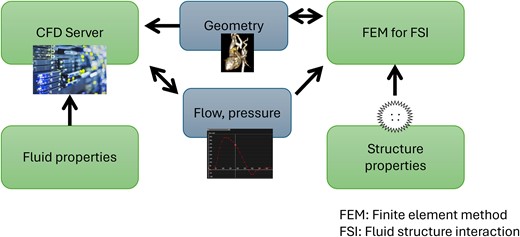
Figure 7
A sophisticated CFD workflow with consideration of fluid–structure interaction (FSI): CFD is based on fixed fluid properties and variable boundary conditions. The finite-element method (FEM) is applied for simulation of FSI and is based on fixed structure properties and variable boundary conditions. Boundary conditions can be anatomic geometry on the one hand and flow or pressure on the other hand. They form a link between CFD and FSI. In the course of the simulation, CFD calculations can have an impact on flow or pressure boundary conditions, while FSI calculations can have an impact on geometry boundary conditions.
Deep learning
DL approaches can improve image acquisition, reconstruction, and analysis. They can reconstruct images with similar or superior image quality to CS in a fraction of the time. Studies in CHD have mainly focused on rapid gated or real-time acquisitions for ventricular volume or flow assessment. One of the first studies focused on DL reconstruction of radial real-time images for assessment of ventricular volumes in CHD.201 The authors demonstrated a reduction in total scan time from ∼280 s for a conventional acquisition to ∼18 s for real-time imaging. More importantly, they found that overall reconstruction time with the DL network was five times faster than CS. Other studies have demonstrated similar findings with rapid gated Cartesian acquisitions for assessment of ventricular volumes.202 DL methods have also been used for flow quantification, including spiral real-time and Cartesian gated approaches.203,204
DL networks have been used in a few 3D imaging studies either to accelerate image acquisition with highly undersampled k-space sampling or by acquisition of lower-resolution 3D whole-heart images that can be acquired in 3× less time.79,205 In the latter case, the low-resolution images underwent DL super-resolution reconstruction, which provided recovery of high-resolution features that was particularly evident in small vessels.206
The other area in which DL is increasingly used is segmentation of CHD data, including 2D cine and 3D data. Segmentation of ventricular volumes is probably the most pressing task. Convolutional networks have been demonstrated to perform reasonably well for this task207 using generative adversarial network to artificially synthesize CMR images and their corresponding segmentation.207 More recently, larger training data sets have become available, and U-Nets have been used to perform highly robust segmentation.208 Segmentation of 3D data is also important for applications such as 3D printing and computational simulation. Several models based on 2D and 3D segmentation have been shown to be robust and reach human levels of accuracy and reproducibility.209
CMR strain imaging
Strain analysis inferred from 2D CMR-feature tracking (FT; syn. tissue tracking) provides a practicable and additive method for a sensitive assessment of myocardial deformation in longitudinal, circumferential, and radial planes (Figure 8). It has also been shown to help in early disease prediction.210–212
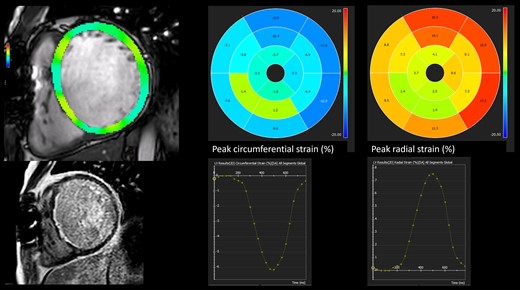
Figure 8
2D feature tracking in a 29-year-old male status post-repair of subaortic stenosis, cor triatriatum, and ventricular septal defect who also underwent resection of subaortic re-stenosis. Subendocardial late gadolinium enhancement is present (red arrows). Longitudinal and circumferential peak strain values are significantly reduced.
During post-processing, the imaging software identifies small geometric structures (features) that are followed through all frames during the cardiac cycle in SSFP cine images. Displacement of these myocardial features is used to calculate myocardial strain (%) and strain rate (1/s):
Strain (%) = (end diastolic length – end systolic length) \sol end systolic length.213
In contrast to conventional CMR-derived ventricular volumes and EF, strain analysis provides data for global, segmental, and local myocardial function.214
Strain parameters are important indicators, especially in early ventricular (global or segmental) dysfunction. Both paediatric and adult CMR studies demonstrated the superiority of LV global longitudinal strain over LVEF in the sensitive determination of LV myocardial (dys)function.215
Reference values for CMR-FT strain parameters have been published for adults over the last 15 years.216 More recently, such normal CMR-FT strain reference values have been published for different paediatric age and weight groups.217–219 High feasibility was demonstrated for CMR-FT strain.219 Lower inter- and intraobserver variability compared with speckle tracking echocardiography appears to be an advantage.220 Limitations of CMR-FT include a relatively high inter-vendor variability for global and segmental strain values221–223 and lower spatial and temporal resolutions compared with speckle tracking echocardiography (the echocardiographic equivalent to CMR-FT) that can impair the detection of local displacements and negatively affect segmental strain values especially in the context of higher heart rates in children.213,224 Cine displacement encoding using stimulated echoes as a another method to measure strain values can be used to improve spatial and temporal resolution, but is not widely used.225
However, the increasing diagnostic and prognostic value of strain analysis derived from CMR-FT in paediatric cardiology has been demonstrated in several studies (Table 2), in particular for coarctation of the aorta,215 pulmonary arterial hypertension,226 hypertrophic cardiomyopathy,227 dilated cardiomyopathy,228,229 and corrected CHD such as repaired ToF.210,230–233
Table 2Paediatric CMR-FT studies
Study
. | Patients’ number
. | Heart disease
. | Examined CMR strain parameters
. | Main results
. |
---|
Kutty et al. (2013)215 | 81 | Coarctation of the aorta (COA) | LV-GLS; LV-GRS; LV-GCS | LVEF, LV-GLS, and LV-GRS are reduced, while LV-GCS is preserved in post-repair COA. LV-GLS reduction is more noticeable in the presence of LVH. LV-GLS as an indicator of early LV dysfunction. |
Siegel et al. (2018)229 | 10 | Dilated cardiomyopathy associated with Duchenne muscular dystrophy (DMD) | LV-GLS, LV-GCS | LV-GCS/LV-GLS demonstrated differences between subjects with DMD and controls not detected by speckle tracking echocardiography. CMR-FT also demonstrated differences in late gadolinium enhancement (LGE)-positive and LGE-negative segments in patients with DMD. |
Vigneault et al. (2019)227 | 99 | Hypertrophic cardiomyopathy (HCM) | LV-GCS, circumferential transmural strain difference (cTSD) | LV-GCS and cTSD identify myocardial dysfunction in patients with overt HCM. Contractile abnormalities are present even when LV wall thickness is normal. |
Hagdorn et al. (2019)210 | 172 | Repaired ToF | LV-GCSR (strain rate) | LV-GCSR was a significant and independent predictor of a combined endpoint of sustained and non-sustained VT. |
Hasan et al. (2022)226 | 20 | Pulmonary arterial hypertension (PAH) | RV-GLS, RV-GCS, RV-GRS | Strong correlations between RV-GLS, RV-GCS, RV-GRS and the EPPVDN paediatric PH risk score. RV strain parameters indicate disease severity in paediatric PAH. |
Krupickova et al. (2022)234 | 60 | Paediatric inflammatory multisystem syndrome (PIMS) | LV-GLS, LV-GRS, LV-GCS | All strain parameters are within normal range within 2.5 months after the onset of PIMS, although lower than normal controls. |
Study
. | Patients’ number
. | Heart disease
. | Examined CMR strain parameters
. | Main results
. |
---|
Kutty et al. (2013)215 | 81 | Coarctation of the aorta (COA) | LV-GLS; LV-GRS; LV-GCS | LVEF, LV-GLS, and LV-GRS are reduced, while LV-GCS is preserved in post-repair COA. LV-GLS reduction is more noticeable in the presence of LVH. LV-GLS as an indicator of early LV dysfunction. |
Siegel et al. (2018)229 | 10 | Dilated cardiomyopathy associated with Duchenne muscular dystrophy (DMD) | LV-GLS, LV-GCS | LV-GCS/LV-GLS demonstrated differences between subjects with DMD and controls not detected by speckle tracking echocardiography. CMR-FT also demonstrated differences in late gadolinium enhancement (LGE)-positive and LGE-negative segments in patients with DMD. |
Vigneault et al. (2019)227 | 99 | Hypertrophic cardiomyopathy (HCM) | LV-GCS, circumferential transmural strain difference (cTSD) | LV-GCS and cTSD identify myocardial dysfunction in patients with overt HCM. Contractile abnormalities are present even when LV wall thickness is normal. |
Hagdorn et al. (2019)210 | 172 | Repaired ToF | LV-GCSR (strain rate) | LV-GCSR was a significant and independent predictor of a combined endpoint of sustained and non-sustained VT. |
Hasan et al. (2022)226 | 20 | Pulmonary arterial hypertension (PAH) | RV-GLS, RV-GCS, RV-GRS | Strong correlations between RV-GLS, RV-GCS, RV-GRS and the EPPVDN paediatric PH risk score. RV strain parameters indicate disease severity in paediatric PAH. |
Krupickova et al. (2022)234 | 60 | Paediatric inflammatory multisystem syndrome (PIMS) | LV-GLS, LV-GRS, LV-GCS | All strain parameters are within normal range within 2.5 months after the onset of PIMS, although lower than normal controls. |
Table 2Paediatric CMR-FT studies
Study
. | Patients’ number
. | Heart disease
. | Examined CMR strain parameters
. | Main results
. |
---|
Kutty et al. (2013)215 | 81 | Coarctation of the aorta (COA) | LV-GLS; LV-GRS; LV-GCS | LVEF, LV-GLS, and LV-GRS are reduced, while LV-GCS is preserved in post-repair COA. LV-GLS reduction is more noticeable in the presence of LVH. LV-GLS as an indicator of early LV dysfunction. |
Siegel et al. (2018)229 | 10 | Dilated cardiomyopathy associated with Duchenne muscular dystrophy (DMD) | LV-GLS, LV-GCS | LV-GCS/LV-GLS demonstrated differences between subjects with DMD and controls not detected by speckle tracking echocardiography. CMR-FT also demonstrated differences in late gadolinium enhancement (LGE)-positive and LGE-negative segments in patients with DMD. |
Vigneault et al. (2019)227 | 99 | Hypertrophic cardiomyopathy (HCM) | LV-GCS, circumferential transmural strain difference (cTSD) | LV-GCS and cTSD identify myocardial dysfunction in patients with overt HCM. Contractile abnormalities are present even when LV wall thickness is normal. |
Hagdorn et al. (2019)210 | 172 | Repaired ToF | LV-GCSR (strain rate) | LV-GCSR was a significant and independent predictor of a combined endpoint of sustained and non-sustained VT. |
Hasan et al. (2022)226 | 20 | Pulmonary arterial hypertension (PAH) | RV-GLS, RV-GCS, RV-GRS | Strong correlations between RV-GLS, RV-GCS, RV-GRS and the EPPVDN paediatric PH risk score. RV strain parameters indicate disease severity in paediatric PAH. |
Krupickova et al. (2022)234 | 60 | Paediatric inflammatory multisystem syndrome (PIMS) | LV-GLS, LV-GRS, LV-GCS | All strain parameters are within normal range within 2.5 months after the onset of PIMS, although lower than normal controls. |
Study
. | Patients’ number
. | Heart disease
. | Examined CMR strain parameters
. | Main results
. |
---|
Kutty et al. (2013)215 | 81 | Coarctation of the aorta (COA) | LV-GLS; LV-GRS; LV-GCS | LVEF, LV-GLS, and LV-GRS are reduced, while LV-GCS is preserved in post-repair COA. LV-GLS reduction is more noticeable in the presence of LVH. LV-GLS as an indicator of early LV dysfunction. |
Siegel et al. (2018)229 | 10 | Dilated cardiomyopathy associated with Duchenne muscular dystrophy (DMD) | LV-GLS, LV-GCS | LV-GCS/LV-GLS demonstrated differences between subjects with DMD and controls not detected by speckle tracking echocardiography. CMR-FT also demonstrated differences in late gadolinium enhancement (LGE)-positive and LGE-negative segments in patients with DMD. |
Vigneault et al. (2019)227 | 99 | Hypertrophic cardiomyopathy (HCM) | LV-GCS, circumferential transmural strain difference (cTSD) | LV-GCS and cTSD identify myocardial dysfunction in patients with overt HCM. Contractile abnormalities are present even when LV wall thickness is normal. |
Hagdorn et al. (2019)210 | 172 | Repaired ToF | LV-GCSR (strain rate) | LV-GCSR was a significant and independent predictor of a combined endpoint of sustained and non-sustained VT. |
Hasan et al. (2022)226 | 20 | Pulmonary arterial hypertension (PAH) | RV-GLS, RV-GCS, RV-GRS | Strong correlations between RV-GLS, RV-GCS, RV-GRS and the EPPVDN paediatric PH risk score. RV strain parameters indicate disease severity in paediatric PAH. |
Krupickova et al. (2022)234 | 60 | Paediatric inflammatory multisystem syndrome (PIMS) | LV-GLS, LV-GRS, LV-GCS | All strain parameters are within normal range within 2.5 months after the onset of PIMS, although lower than normal controls. |
Ventriculo-arterial coupling
Ventriculo-arterial coupling (VAC) is a concept to assess the interaction between the ventricular and arterial function.235 It assumes that there is a balanced ratio of elastance of the ventricle and arterial system and that this system tries to reach optimal efficiency.236 Invasive measurement of pressure–volume loops under different loading conditions is the gold standard for assessment of VAC. This method is not widely applicable in clinical practice but is predictive of outcomes in patients with aortic valve replacement and pulmonary hypertension.237,238 Attempts have been made to assess VAC non-invasively.239,240 These attempts have been relatively successful for coupling between the left ventricle and aorta. Non-invasive VAC has been linked to the worsening of chronic heart failure using the method described by Chen et al.239,240 Interaction between the right ventricle and pulmonary artery is more difficult to assess because of the lack of reliable non-invasive pressure measurements. While some have attempted to use tricuspid regurgitation in conjunction with the Bernoulli equation, this method has been shown to only estimate arterial elastance.241 A relatively popular measurement is the so-called volumetric approach, where ESV and SV measured by CMR are used to assess arterial coupling.242–246 The quotient SV/ESV has been shown to be a strong predictor for adverse events in pulmonary arterial hypertension.247 However, this method is not a representation of VAC but a numerical substitute to and therefore should not be interpreted as VAC.247,248 Novel approaches such as global longitudinal strain/pulse wave velocity and wave reflection analysis were proposed as possible methods to non-invasively assess VAC using echocardiography and/or CMR.249,250 However, more research is needed before these methods can become clinically established.251–254 Further research should also explore the role of CMR for VAC measurements and their predictive value for clinical outcomes.
3D Printing
3D modelling allows 3D visualization of cardiac anatomy from primary 3D data sets acquired mainly by cross-sectional imaging.5 Since the beginning of this century, 3D modelling technology has markedly improved, and its application is gaining interest for surgical or interventional planning, procedural simulation, or education in CHD255–258 (Figure 9).
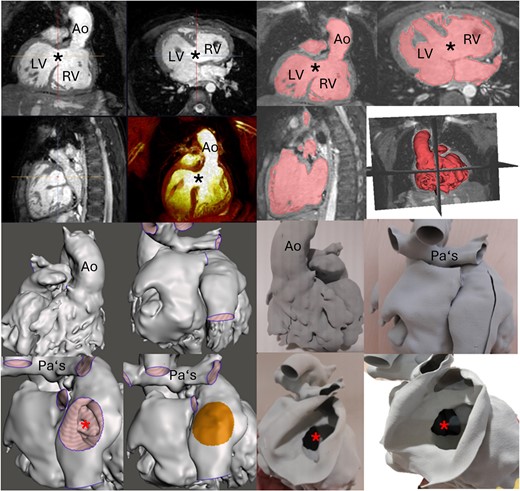
Figure 9
CMR evaluation of a 10-year-old patient with atrioventricular and ventriculo-arterial discordance, pulmonary atresia, and ventricular septal defect (black star) post-Glenn operation. The upper eight images show images from a 3D steady-state free precession sequence and their segmentation. The lower eight images show the virtual and printed cardiac model. Ao, aorta; atrial septal defect, red star; LV, left ventricle; PAs, pulmonary arteries; RV, right ventricle.
Creating a 3D (printed) model involves image acquisition, segmentation of the 3D data set, and transforming it into a stereolithography or Standard Tessellation Language or another format, and finally printing the model. A high-resolution free-breathing motion-corrected 3D volume CMR acquisition with high vascular contrast is mandatory to perform an accurate segmentation process, and significant advances in motion correction and image reconstruction now enable obtaining a 3D whole-heart scan in 3–7 min depending on the spatial resolution.259,260 The volume data acquired should be isotropic. ECG gating and respiration navigation should be performed to avoid cardiac and respiratory motion.261 ECG-gated 3D SSFP imaging is currently the most frequently used image acquisition technique. However, other approaches may be more suited,261 such as imaging data sets from ferumoxytol contrast-enhanced angiography. The type of segmentation, the printing resolution, and the printing material used depend on the intended use of the model, availability, and cost. The segmentation process is usually achieved in a semi-automatic mode, with subsequent manual correction. The operator’s knowledge of the cardiac anatomy is crucial for high-quality 3D-printed models. For surgical planning of complex CHD, the segmentation of the endocardial surface anatomy is generally sufficient,261 and a soft material for printing is preferred. Visualization of the virtual 3D model should be performed prior to printing to check the model quality. Close communication between the production team and the interventionalist is suggested to achieve the best outcome for the patient.262 Model limitations include distortions of fine moving structures such as valve leaflets and chordae tendineae.261
Virtual reality/augmented reality
Virtual reality (VR) applications use models made from anatomical and/or functional data for visualization and simulation, prior to cardiac intervention or surgery or during catheterization. Augmented reality is a combination of physical reality and VR. VR is computing intensive and interactive. Data are imported into display software and used on a screen, with a head-mounted display, or on the patient surface.
As for 3D printing, a high-resolution 3D data set must be acquired, then converted into a transferable file (which may be vendor specific), and segmented by an operator trained in CHD. No material or time for printing is necessary, and virtual models lend themselves to modifications by the user with immediate feedback, e.g. virtual construction of Fontan conduits200 or baffles.263 Patient-specific procedure planning is facilitated.264–266 For catheter interventions (electrophysiology or structural), fusion techniques are promising to reduce radiation and contrast exposure.267 Lastly, VR may be preferable to printed models for education.268 There are, however, challenges to evaluation and approval of such applications regarding image quality and calibration, usability, user training, reproducibility, indications, and trial outcome assessment.269
Conclusion
This scientific statement aims to provide a comprehensive summary of clinical applications of advanced CMR techniques in children and adults with CHD. New CMR imaging and post-processing methods are steadily expanding the field. While some of them are already used clinically, others are promising novel technologies that will likely further strengthen the role of CMR for CHD assessment.
Acknowledgements
The authors thank Dr Miriam Conway (Royal Brompton Hospital, Part of Guy’s and St Thomas’ NHS Foundation Trust, Sydney Street, London, SW3 6NP, UK) for her help in writing the diffusion tensor imaging section of this document.
Supplementary data
Supplementary data are available at European Heart Journal - Cardiovascular Imaging online.
Funding
None.
Data availability
No new data were generated or analysed in support of this research.
References
1Fratz
S
, Chung
T
, Greil
GF
, Samyn
MM
, Taylor
AM
, Valsangiacomo Buechel
ER
et al.
Guidelines and protocols for cardiovascular magnetic resonance in children and adults with congenital heart disease: SCMR expert consensus group on congenital heart disease
.
J Cardiovasc Magn Reson
2013
;
15
:
51
.
2Valsangiacomo Buechel
ER
, Grosse-Wortmann
L
, Fratz
S
, Eichhorn
J
, Sarikouch
S
, Greil
GF
et al.
Indications for cardiovascular magnetic resonance in children with congenital and acquired heart disease: an expert consensus paper of the Imaging Working Group of the AEPC and the Cardiovascular Magnetic Resonance Section of the EACVI
.
Cardiol Young
2015
;
25
:
819
–
38
.
3Fogel
MA
, Anwar
S
, Broberg
C
, Browne
L
, Chung
T
, Johnson
T
et al.
Society for Cardiovascular Magnetic Resonance/European Society of Cardiovascular Imaging/American Society of Echocardiography/Society for Pediatric Radiology/North American Society for Cardiovascular Imaging Guidelines for the Use of Cardiac Magnetic Resonance in Pediatric Congenital and Acquired Heart Disease: Endorsed by The American Heart Association
.
Circ Cardiovasc Imaging
2022
;
15
:
e014415
.
4Dorfman
AL
, Geva
T
, Samyn
MM
, Greil
G
, Krishnamurthy
R
, Messroghli
D
et al.
SCMR expert consensus statement for cardiovascular magnetic resonance of acquired and non-structural pediatric heart disease
.
J Cardiovasc Magn Reson
2022
;
24
:
44
.
5Chessa
M
, Van De Bruaene
A
, Farooqi
K
, Valverde
I
, Jung
C
, Votta
E
et al.
Three-dimensional printing, holograms, computational modelling, and artificial intelligence for adult congenital heart disease care: an exciting future
.
Eur Heart J
2022
;
43
:
2672
–
84
.
6Dori
Y
, Smith
CL
.
Lymphatic disorders in patients with single ventricle heart disease
.
Front Pediatr
2022
;
10
:
828107
.
7Garcia-Canadilla
P
, Dejea
H
, Bonnin
A
, Balicevic
V
, Loncaric
S
, Zhang
C
et al.
Complex congenital heart disease associated with disordered myocardial architecture in a midtrimester human fetus
.
Circ Cardiovasc Imaging
2018
;
11
:
e007753
.
8Hundley
WG
, Bluemke
DA
, Bogaert
J
, Flamm
SD
, Fontana
M
, Friedrich
MG
et al.
Society for Cardiovascular Magnetic Resonance (SCMR) guidelines for reporting cardiovascular magnetic resonance examinations
.
J Cardiovasc Magn Reson
2022
;
24
:
29
.
9Messroghli
DR
, Moon
JC
, Ferreira
VM
, Grosse-Wortmann
L
, He
T
, Kellman
P
et al.
Clinical recommendations for cardiovascular magnetic resonance mapping of T1, T2, T2* and extracellular volume: a consensus statement by the Society for Cardiovascular Magnetic Resonance (SCMR) endorsed by the European Association for Cardiovascular Imaging (EACVI)
.
J Cardiovasc Magn Reson
2017
;
19
:
75
.
10Dyverfeldt
P
, Bissell
M
, Barker
AJ
, Bolger
AF
, Carlhäll
CJ
, Ebbers
T
et al.
4D flow cardiovascular magnetic resonance consensus statement
.
J Cardiovasc Magn Reson
2015
;
17
:
72
.
11Niendorf
T
, Schulz-Menger
J
, Paul
K
, Huelnhagen
T
, Ferrari
VA
, Hodge
R
.
High field cardiac magnetic resonance imaging: a case for ultrahigh field cardiac magnetic resonance
.
Circ Cardiovasc Imaging
2017
;
10
:
e005460
.
12Rajiah
P
, Bolen
MA
.
Cardiovascular MR imaging at 3 T: opportunities, challenges, and solutions
.
Radiographics
2014
;
34
:
1612
–
35
.
13Prothmann
M
, von Knobelsdorff-Brenkenhoff
F
, Töpper
A
, Dieringer
MA
, Shahid
E
, Graessl
A
et al.
High spatial resolution cardiovascular magnetic resonance at 7.0 Tesla in patients with hypertrophic cardiomyopathy—first experiences: lesson learned from 7.0 Tesla
.
PLoS One
2016
;
11
:
e0148066
.
14Reiter
T
, Lohr
D
, Hock
M
, Ankenbrand
MJ
, Stefanescu
MR
, Kosmala
A
et al.
On the way to routine cardiac MRI at 7 Tesla—a pilot study on consecutive 84 examinations
.
PLoS One
2021
;
16
:
e0252797
.
15Stäb
D
, Al Najjar
A
, O'Brien
K
, Strugnell
W
, Richer
J
, Rieger
J
et al.
Cardiac magnetic resonance imaging at 7 Tesla
.
J Vis Exp
2019
.
16Ibrahim
EH
, Arpinar
VE
, Muftuler
LT
, Stojanovska
J
, Nencka
AS
, Koch
KM
.
Cardiac functional magnetic resonance imaging at 7T: image quality optimization and ultra-high field capabilities
.
World J Radiol
2020
;
12
:
231
–
46
.
17Vachha
B
, Huang
SY
.
MRI with ultrahigh field strength and high-performance gradients: challenges and opportunities for clinical neuroimaging at 7 T and beyond
.
Eur Radiol Exp
2021
;
5
:
35
.
18Campbell-Washburn
AE
, Ramasawmy
R
, Restivo
MC
, Bhattacharya
I
, Basar
B
, Herzka
DA
et al.
Opportunities in interventional and diagnostic imaging by using high-performance low-field-strength MRI
.
Radiology
2019
;
293
:
384
–
93
.
19Simonetti
OP
, Ahmad
R
.
Low-Field cardiac magnetic resonance imaging: a compelling case for cardiac magnetic resonance’s future
.
Circ Cardiovasc Imaging
2017
;
10
:
e005446
.
20Qin
C
, Murali
S
, Lee
E
, Supramaniam
V
, Hausenloy
DJ
, Obungoloch
J
et al.
Sustainable low-field cardiovascular magnetic resonance in changing healthcare systems
.
Eur Heart J Cardiovasc Imaging
2022
;
23
:
e246
–
60
.
21Doo
FX
, Vosshenrich
J
, Cook
TS
, Moy
L
, Almeida
EPRP
, Woolen
SA
et al.
Environmental sustainability and AI in radiology: a double-edged sword
.
Radiology
2024
;
310
:
e232030
.
22Lotz
J
, Meier
C
, Leppert
A
, Galanski
M
.
Cardiovascular flow measurement with phase-contrast MR imaging: basic facts and implementation
.
Radiographics
2002
;
22
:
651
–
71
.
23Chai
P
, Mohiaddin
R
.
How we perform cardiovascular magnetic resonance flow assessment using phase-contrast velocity mapping
.
J Cardiovasc Magn Reson
2005
;
7
:
705
–
16
.
24Demirkiran
A
, van Ooij
P
, Westenberg
JJM
, Hofman
MBM
, van Assen
HC
, Schoonmade
LJ
et al.
Clinical intra-cardiac 4D flow CMR: acquisition, analysis, and clinical applications
.
Eur Heart J Cardiovasc Imaging
2022
;
23
:
154
–
65
.
25Isorni
MA
, Martins
D
, Ben Moussa
N
, Monnot
S
, Boddaert
N
, Bonnet
D
et al.
4D flow MRI versus conventional 2D for measuring pulmonary flow after tetralogy of Fallot repair
.
Int J Cardiol
2020
;
300
:
132
–
6
.
26Isorni
MA
, Moisson
L
, Moussa
NB
, Monnot
S
, Raimondi
F
, Roussin
R
et al.
4D flow cardiac magnetic resonance in children and adults with congenital heart disease: clinical experience in a high volume center
.
Int J Cardiol
2020
;
320
:
168
–
77
.
27Markl
M
, Frydrychowicz
A
, Kozerke
S
, Hope
M
, Wieben
O
.
4D flow MRI
.
J Magn Reson Imaging
2012
;
36
:
1015
–
36
.
28Sodhi
A
, Markl
M
, Popescu
AR
, Griffin
LM
, Robinson
JD
, Rigsby
CK
.
Highly accelerated compressed sensing 4D flow MRI in congenital and acquired heart disease: comparison of aorta and main pulmonary artery flow parameters with conventional 4D flow MRI in children and young adults
.
Pediatr Radiol
2023
;
53
:
2597
–
607
.
29Rijnberg
FM
, Westenberg
JJM
, van Assen
HC
, Juffermans
JF
, Kroft
LJM
, van den Boogaard
PJ
et al.
4D flow cardiovascular magnetic resonance derived energetics in the Fontan circulation correlate with exercise capacity and CMR-derived liver fibrosis/congestion
.
J Cardiovasc Magn Reson
2022
;
24
:
21
.
30Oyama-Manabe
N
, Aikawa
T
, Tsuneta
S
, Manabe
O
.
Clinical applications of 4D flow MR imaging in aortic valvular and congenital heart disease
.
Magn Reson Med Sci
2022
;
21
:
319
–
26
.
31Horowitz
MJ
, Kupsky
DF
, El-Said
HG
, Alshawabkeh
L
, Kligerman
SJ
, Hsiao
A
.
4D flow MRI quantification of congenital shunts: comparison to invasive catheterization
.
Radiol Cardiothorac Imaging
2021
;
3
:
e200446
.
32Raimondi
F
, Martins
D
, Coenen
R
, Panaioli
E
, Khraiche
D
, Boddaert
N
et al.
Prevalence of venovenous shunting and high-output state quantified with 4D flow MRI in patients with Fontan circulation
.
Radiol Cardiothorac Imaging
2021
;
3
:
e210161
.
33Urmeneta Ulloa
J
, Álvarez Vázquez
A
, Martínez de Vega
V
, Cabrera
JÁ
.
Evaluation of cardiac shunts with 4D flow cardiac magnetic resonance: intra- and interobserver variability
.
J Magn Reson Imaging
2020
;
52
:
1055
–
63
.
34Valverde
I
, Nordmeyer
S
, Uribe
S
, Greil
G
, Berger
F
, Kuehne
T
et al.
Systemic-to-pulmonary collateral flow in patients with palliated univentricular heart physiology: measurement using cardiovascular magnetic resonance 4D velocity acquisition
.
J Cardiovasc Magn Reson
2012
;
14
:
25
.
35Jarvis
K
, Vonder
M
, Barker
AJ
, Schnell
S
, Rose
M
, Carr
J
et al.
Hemodynamic evaluation in patients with transposition of the great arteries after the arterial switch operation: 4D flow and 2D phase contrast cardiovascular magnetic resonance compared with Doppler echocardiography
.
J Cardiovasc Magn Reson
2016
;
18
:
59
.
36Chelu
RG
, van den Bosch
AE
, van Kranenburg
M
, Hsiao
A
, van den Hoven
AT
, Ouhlous
M
et al.
Qualitative grading of aortic regurgitation: a pilot study comparing CMR 4D flow and echocardiography
.
Int J Cardiovasc Imaging
2016
;
32
:
301
–
7
.
37van der Hulst
AE
, Westenberg
JJ
, Kroft
LJ
, Bax
JJ
, Blom
NA
, de Roos
A
et al.
Tetralogy of fallot: 3D velocity-encoded MR imaging for evaluation of right ventricular valve flow and diastolic function in patients after correction
.
Radiology
2010
;
256
:
724
–
34
.
38Adriaans
BP
, Westenberg
JJM
, van Cauteren
YJM
, Gerretsen
S
, Elbaz
MSM
, Bekkers
SCAM
et al.
Clinical assessment of aortic valve stenosis: comparison between 4D flow MRI and transthoracic echocardiography
.
J Magn Reson Imaging
2020
;
51
:
472
–
80
.
39Juffermans
JF
, Minderhoud
SCS
, Wittgren
J
, Kilburg
A
, Ese
A
, Fidock
B
et al.
Multicenter consistency assessment of valvular flow quantification with automated valve tracking in 4D flow CMR
.
Cardiovascular Imaging
2021
;
14
:
1354
–
66
.
40Calkoen
EE
, Westenberg
JJM
, Kroft
LJ
, Blom
NA
, Hazekamp
MG
, Rijlaarsdam
ME
et al.
Characterization and quantification of dynamic eccentric regurgitation of the left atrioventricular valve after atrioventricular septal defect correction with 4D flow cardiovascular magnetic resonance and retrospective valve tracking
.
J Cardiovasc Magn Reson
2015
;
17
:
18
.
41Blanken
CPS
, Westenberg
JJM
, Aben
J-P
, Bijvoet
GP
, Chamuleau
SAJ
, Boekholdt
SM
et al.
Quantification of mitral valve regurgitation from 4D flow MRI using semiautomated flow tracking
.
Radiol Cardiothorac Imaging
2020
;
2
:
e200004
.
42Rengier
F
, Delles
M
, Eichhorn
J
, Azad
Y-J
, von Tengg-Kobligk
H
, Ley-Zaporozhan
J
et al.
Noninvasive 4D pressure difference mapping derived from 4D flow MRI in patients with repaired aortic coarctation: comparison with young healthy volunteers
.
Int J Cardiovasc Imaging
2015
;
31
:
823
–
30
.
43Riesenkampff
E
, Fernandes
JF
, Meier
S
, Goubergrits
L
, Kropf
S
, Schubert
S
et al.
Pressure fields by flow-sensitive, 4D, velocity-encoded CMR in patients with aortic coarctation
.
JACC Cardiovasc Imaging
2014
;
7
:
920
–
6
.
44Kamphuis
VP
, Roest
AAW
, Ajmone Marsan
N
, van den Boogaard
PJ
, Kroft
LJM
, Aben
J-P
et al.
Automated cardiac valve tracking for flow quantification with four-dimensional flow MRI
.
Radiology
2019
;
290
:
70
–
8
.
45Ramos
JG
, Wieslander
B
, Fyrdahl
A
, Reiter
G
, Reiter
U
, Jin
N
et al.
Pulmonary hypertension by catheterization is more accurately detected by cardiovascular magnetic resonance 4D-flow than echocardiography
.
JACC Cardiovasc Imaging
2023
;
16
:
558
–
9
.
46Geiger
J
, Callaghan
FM
, Burkhardt
BEU
, Valsangiacomo Buechel
ER
, Kellenberger
CJ
.
Additional value and new insights by four-dimensional flow magnetic resonance imaging in congenital heart disease: application in neonates and young children
.
Pediatr Radiol
2021
;
51
:
1503
–
17
.
47Bissell
MM
, Raimondi
F
, Ait Ali
L
, Allen
BD
, Barker
AJ
, Bolger
A
et al.
4D flow cardiovascular magnetic resonance consensus statement: 2023 update
.
J Cardiovasc Magn Reson
2023
;
25
:
40
.
48Valvano
G
, Martini
N
, Huber
A
, Santelli
C
, Binter
C
, Chiappino
D
et al.
Accelerating 4 D flow MRI by exploiting low-rank matrix structure and hadamard sparsity
.
Magn Reson Med
2017
;
78
:
1330
–
41
.
49Broberg
CS
, Burchill
LJ
.
Myocardial factor revisited: the importance of myocardial fibrosis in adults with congenital heart disease
.
Int J Cardiol
2015
;
189
:
204
–
10
.
50Endrikat
J
, Gutberlet
M
, Hoffmann
KT
, Schöckel
L
, Bhatti
A
, Harz
C
et al.
Clinical safety of gadobutrol: review of over 25 years of use exceeding 100 million administrations
.
Invest Radiol
, Online ahead of print. 2024. doi:
51Lee
S
, Kim
P
, Im
DJ
, Suh
YJ
, Hong
YJ
, Choi
BW
et al.
The image quality and diagnostic accuracy of T1-mapping-based synthetic late gadolinium enhancement imaging: comparison with conventional late gadolinium enhancement imaging in real-life clinical situation
.
J Cardiovasc Magn Reson
2022
;
24
:
28
.
52Zhang
Q
, Burrage
MK
, Lukaschuk
E
, Shanmuganathan
M
, Popescu
IA
, Nikolaidou
C
et al.
Toward replacing late gadolinium enhancement with artificial intelligence virtual native enhancement for gadolinium-free cardiovascular magnetic resonance tissue characterization in hypertrophic cardiomyopathy
.
Circulation
2021
;
144
:
589
–
99
.
53Peters
AA
, Wagner
B
, Spano
G
, Haupt
F
, Ebner
L
, Kunze
KP
et al.
Myocardial scar detection in free-breathing Dixon-based fat- and water-separated 3D inversion recovery late-gadolinium enhancement whole heart MRI
.
Int J Cardiovasc Imaging
2023
;
39
:
135
–
44
.
54Rashid
I
, Cruz
G
, Seiberlich
N
, Hamilton
JI
.
Cardiac MR fingerprinting: overview, technical developments, and applications
.
J Magn Reson Imaging
, Online ahead of print. 2023. doi:
55de Alba
CG
, Khan
A
, Woods
P
, Broberg
CS
.
Left ventricular strain and fibrosis in adults with repaired tetralogy of Fallot: a case-control study
.
Int J Cardiol
2021
;
323
:
34
–
9
.
56Riesenkampff
E
, Luining
W
, Seed
M
, Chungsomprasong
P
, Manlhiot
C
, Elders
B
et al.
Increased left ventricular myocardial extracellular volume is associated with longer cardiopulmonary bypass times, biventricular enlargement and reduced exercise tolerance in children after repair of tetralogy of Fallot
.
J Cardiovasc Magn Reson
2016
;
18
:
75
.
57Yim
D
, Riesenkampff
E
, Caro-Dominguez
P
, Yoo
SJ
, Seed
M
, Grosse-Wortmann
L
.
Assessment of diffuse ventricular myocardial fibrosis using native T1 in children with repaired tetralogy of fallot
.
Circ Cardiovasc Imaging
2017
;
10
:
e005695
.
58Ghonim
S
, Gatzoulis
MA
, Ernst
S
, Li
W
, Moon
JC
, Smith
GC
et al.
Predicting survival in repaired tetralogy of fallot: a lesion-specific and personalized approach
.
JACC Cardiovasc Imaging
2022
;
15
:
257
–
68
.
59Ladouceur
M
, Bruneval
P
, Mousseaux
E
.
Cardiovascular flashlight. Magnetic resonance assessment of fibrosis in systemic right ventricle after atrial switch procedure
.
Eur Heart J
2009
;
30
:
2613
.
60Babu-Narayan
SV
, Goktekin
O
, Moon
JC
, Broberg
CS
, Pantely
GA
, Pennell
DJ
et al.
Late gadolinium enhancement cardiovascular magnetic resonance of the systemic right ventricle in adults with previous atrial redirection surgery for transposition of the great arteries
.
Circulation
2005
;
111
:
2091
–
8
.
61Babu-Narayan
SV
, Prati
D
, Rydman
R
, Dimopoulos
K
, Diller
GP
, Uebing
A
et al.
Dyssynchrony and electromechanical delay are associated with focal fibrosis in the systemic right ventricle—insights from echocardiography
.
Int J Cardiol
2016
;
220
:
382
–
8
.
62Rathod
RH
, Prakash
A
, Powell
AJ
, Geva
T
.
Myocardial fibrosis identified by cardiac magnetic resonance late gadolinium enhancement is associated with adverse ventricular mechanics and ventricular tachycardia late after Fontan operation
.
J Am Coll Cardiol
2010
;
55
:
1721
–
8
.
63Aly
S
, Seed
M
, Yoo
SJ
, Lam
C
, Grosse-Wortmann
L
.
Myocardial fibrosis in pediatric patients with Ebstein’s anomaly
.
Circ Cardiovasc Imaging
2021
;
14
:
e011136
.
64Dusenbery
SM
, Jerosch-Herold
M
, Rickers
C
, Colan
SD
, Geva
T
, Newburger
JW
et al.
Myocardial extracellular remodeling is associated with ventricular diastolic dysfunction in children and young adults with congenital aortic stenosis
.
J Am Coll Cardiol
2014
;
63
:
1778
–
85
.
65Vaikom House
AK
, Chetan
D
, Mital
S
, Grosse-Wortmann
L
.
Patients with repaired tetralogy of Fallot and the HIF1A1744C/T variant have increased imaging markers of diffuse myocardial fibrosis
.
Int J Cardiol
2022
;
350
:
33
–
5
.
66Chen
CA
, Dusenbery
SM
, Valente
AM
, Powell
AJ
, Geva
T
.
Myocardial ECV fraction assessed by CMR is associated with type of hemodynamic load and arrhythmia in repaired tetralogy of Fallot
.
JACC Cardiovasc Imaging
2016
;
9
:
1
–
10
.
67Pisesky
A
, Reichert
MJE
, de Lange
C
, Seed
M
, Yoo
SJ
, Lam
CZ
et al.
Adverse fibrosis remodeling and aortopulmonary collateral flow are associated with poor Fontan outcomes
.
J Cardiovasc Magn Reson
2021
;
23
:
134
.
68Cornicelli
MD
, Rigsby
CK
, Rychlik
K
, Pahl
E
, Robinson
JD
.
Diagnostic performance of cardiovascular magnetic resonance native T1 and T2 mapping in pediatric patients with acute myocarditis
.
J Cardiovasc Magn Reson
2019
;
21
:
40
.
69Ferreira
VM
, Schulz-Menger
J
, Holmvang
G
, Kramer
CM
, Carbone
I
, Sechtem
U
et al.
Cardiovascular magnetic resonance in nonischemic myocardial inflammation: expert recommendations
.
J Am Coll Cardiol
2018
;
72
:
3158
–
76
.
70Law
YM
, Lal
AK
, Chen
S
, Čiháková
D
, Cooper
LT
Jr, Deshpande
S
et al.
Diagnosis and management of myocarditis in children: a scientific statement from the American Heart Association
.
Circulation
2021
;
144
:
e123
–
35
.
71Soslow
JH
, Samyn
MM
.
Multi-modal imaging of the pediatric heart transplant recipient
.
Transl Pediatr
2019
;
8
:
322
–
38
.
72Aquaro
GD
, Ghebru Habtemicael
Y
, Camastra
G
, Monti
L
, Dellegrottaglie
S
, Moro
C
et al.
Prognostic value of repeating cardiac magnetic resonance in patients with acute myocarditis
.
J Am Coll Cardiol
2019
;
74
:
2439
–
48
.
73Bohbot
Y
, Sanguineti
F
, Renard
C
, Hovasse
T
, Limouzineau
I
, Unterseeh
T
et al.
Associated factors and clinical implications of dynamic changes in late gadolinium enhancement after acute myocarditis
.
JACC Cardiovasc Imaging
2023
;
16
:
859
–
61
.
74Hamilton
JI
, Jiang
Y
, Chen
Y
, Ma
D
, Lo
WC
, Griswold
M
et al.
MR fingerprinting for rapid quantification of myocardial T(1), T(2), and proton spin density
.
Magn Reson Med
2017
;
77
:
1446
–
58
.
75Moghari
MH
, Barthur
A
, Amaral
ME
, Geva
T
, Powell
AJ
.
Free-breathing whole-heart 3D cine magnetic resonance imaging with prospective respiratory motion compensation
.
Magn Reson Med
2018
;
80
:
181
–
9
.
76Merton
R
, Bosshardt
D
, Strijkers
GJ
, Nederveen
AJ
, Schrauben
EM
, van Ooij
P
.
Reproducibility of 3D thoracic aortic displacement from 3D cine balanced SSFP at 3T without contrast enhancement
.
Magn Reson Med
2024
;
91
:
466
–
80
.
77Nguyen
KL
, Ghosh
RM
, Griffin
LM
, Yoshida
T
, Bedayat
A
, Rigsby
CK
et al.
Four-dimensional multiphase steady-state MRI with ferumoxytol enhancement: early multicenter feasibility in pediatric congenital heart disease
.
Radiology
2021
;
300
:
162
–
73
.
78Roy
CW
, Di Sopra
L
, Whitehead
KK
, Piccini
D
, Yerly
J
, Heerfordt
J
et al.
Free-running cardiac and respiratory motion-resolved 5D whole-heart coronary cardiovascular magnetic resonance angiography in pediatric cardiac patients using ferumoxytol
.
J Cardiovasc Magn Reson
2022
;
24
:
39
.
79Küstner
T
, Fuin
N
, Hammernik
K
, Bustin
A
, Qi
H
, Hajhosseiny
R
et al.
CINENet: deep learning-based 3D cardiac CINE MRI reconstruction with multi-coil complex-valued 4D spatio-temporal convolutions
.
Sci Rep
2020
;
10
:
13710
.
80Küstner
T
, Bustin
A
, Jaubert
O
, Hajhosseiny
R
, Masci
PG
, Neji
R
et al.
Fully self-gated free-running 3D Cartesian cardiac CINE with isotropic whole-heart coverage in less than 2 min
.
NMR Biomed
2021
;
34
:
e4409
.
81Cruz
G
, Hammernik
K
, Kuestner
T
, Velasco
C
, Hua
A
, Ismail
TF
et al.
Single-heartbeat cardiac cine imaging via jointly regularized nonrigid motion-corrected reconstruction
.
NMR Biomed
2023
;
36
:
e4942
.
82Curione
D
, Ciliberti
P
, Monti
CB
, Capra
D
, Bordonaro
V
, Ciancarella
P
et al.
Compressed sensing cardiac cine imaging compared with standard balanced steady-state free precession cine imaging in a pediatric population
.
Radiol Cardiothorac Imaging
2022
;
4
:
e210109
.
83Hales
PW
, Schneider
JE
, Burton
RA
, Wright
BJ
, Bollensdorff
C
, Kohl
P
.
Histo-anatomical structure of the living isolated rat heart in two contraction states assessed by diffusion tensor MRI
.
Prog Biophys Mol Biol
2012
;
110
(
2–3
):
319
–
30
.
84Kung
GL
, Nguyen
TC
, Itoh
A
, Skare
S
, Ingels
NB
Jr, Miller
DC
et al.
The presence of two local myocardial sheet populations confirmed by diffusion tensor MRI and histological validation
.
J Magn Reson Imaging
2011
;
34
:
1080
–
91
.
85Nielles-Vallespin
S
, Khalique
Z
, Ferreira
PF
, de Silva
R
, Scott
AD
, Kilner
P
et al.
Assessment of myocardial microstructural dynamics by in vivo diffusion tensor cardiac magnetic resonance
.
J Am Coll Cardiol
2017
;
69
:
661
–
76
.
86Nguyen
C
, Lu
M
, Fan
Z
, Bi
X
, Kellman
P
, Zhao
S
et al.
Contrast-free detection of myocardial fibrosis in hypertrophic cardiomyopathy patients with diffusion-weighted cardiovascular magnetic resonance
.
J Cardiovasc Magn Reson
2015
;
17
:
107
.
87Khalique
Z
, Ferreira
PF
, Scott
AD
, Nielles-Vallespin
S
, Kilner
PJ
, Kutys
R
et al.
Deranged myocyte microstructure in situs Inversus totalis demonstrated by diffusion tensor cardiac magnetic resonance
.
JACC Cardiovasc Imaging
2018
;
11
:
1360
–
2
.
88Campanale
CM
, Scherrer
B
, Afacan
O
, Majeed
A
, Warfield
SK
, Sanders
SP
.
Myofiber organization in the failing systemic right ventricle
.
J Cardiovasc Magn Reson
2020
;
22
:
49
.
89Tous
C
, Gentles
TL
, Young
AA
, Pontré
BP
.
Ex vivo cardiovascular magnetic resonance diffusion weighted imaging in congenital heart disease, an insight into the microstructures of tetralogy of Fallot, biventricular and univentricular systemic right ventricle
.
J Cardiovasc Magn Reson
2020
;
22
:
69
.
90Harmer
J
, Pushparajah
K
, Toussaint
N
, Stoeck
CT
, Chan
R
, Atkinson
D
et al.
In vivo myofibre architecture in the systemic right ventricle
.
Eur Heart J
2013
;
34
:
3640
.
91Alsaied
T
, Rathod
RH
, Aboulhosn
JA
, Budts
W
, Anderson
JB
, Baumgartner
H
et al.
Reaching consensus for unified medical language in Fontan care
.
ESC Heart Fail
2021
;
8
:
3894
–
905
.
92Rychik
J
, Atz
AM
, Celermajer
DS
, Deal
BJ
, Gatzoulis
MA
, Gewillig
MH
et al.
Evaluation and management of the child and adult with Fontan circulation: a scientific statement from the American Heart Association
.
Circulation
2019
;
140
:
e234
–
84
.
93Itkin
M
, Rockson
SG
, Burkhoff
D
.
Pathophysiology of the lymphatic system in patients with heart failure: JACC state-of-the-art review
.
J Am Coll Cardiol
2021
;
78
:
278
–
90
.
94Dori
Y
, Keller
MS
, Fogel
MA
, Rome
JJ
, Whitehead
KK
, Harris
MA
et al.
MRI of lymphatic abnormalities after functional single-ventricle palliation surgery
.
Am J Roentgenol
2014
;
203
:
426
–
31
.
95Krishnamurthy
R
, Hernandez
A
, Kavuk
S
, Annam
A
, Pimpalwar
S
.
Imaging the central conducting lymphatics: initial experience with dynamic MR lymphangiography
.
Radiology
2015
;
274
:
871
–
8
.
96Dori
Y
, Keller
MS
, Rychik
J
, Itkin
M
.
Successful treatment of plastic bronchitis by selective lymphatic embolization in a Fontan patient
.
Pediatrics
2014
;
134
:
e590
–
5
.
97Savla
JJ
, Itkin
M
, Rossano
JW
, Dori
Y
.
Post-Operative chylothorax in patients with congenital heart disease
.
J Am Coll Cardiol
2017
;
69
:
2410
–
22
.
98Biko
DM
, DeWitt
AG
, Pinto
EM
, Morrison
RE
, Johnstone
JA
, Griffis
H
et al.
MRI evaluation of lymphatic abnormalities in the neck and thorax after Fontan surgery: relationship with outcome
.
Radiology
2019
;
291
:
774
–
80
.
99Gooty
VD
, Veeram Reddy
SR
, Greer
JS
, Blair
Z
, Zahr
RA
, Arar
Y
et al.
Lymphatic pathway evaluation in congenital heart disease using 3D whole-heart balanced steady state free precession and T2-weighted cardiovascular magnetic resonance
.
J Cardiovasc Magn Reson
2021
;
23
:
16
.
100Biko
DM
, Smith
CL
, Otero
HJ
, Saul
D
, White
AM
, DeWitt
A
et al.
Intrahepatic dynamic contrast MR lymphangiography: initial experience with a new technique for the assessment of liver lymphatics
.
Eur Radiol
2019
;
29
:
5190
–
6
.
101Lemley
BA
, Biko
DM
, Dewitt
AG
, Glatz
AC
, Goldberg
DJ
, Saravanan
M
et al.
Intrahepatic dynamic contrast-enhanced magnetic resonance lymphangiography: potential imaging signature for protein-losing enteropathy in congenital heart disease
.
J Am Heart Assoc
2021
;
10
:
e021542
.
102Singhal
D
, Börner
K
, Chaikof
EL
, Detmar
M
, Hollmén
M
, Iliff
JJ
et al.
Mapping the lymphatic system across body scales and expertise domains: a report from the 2021 National Heart, Lung, and Blood Institute workshop at the Boston Lymphatic Symposium
.
Front Physiol
2023
;
14
:
1099403
.
103Lloyd
DFA
, Pushparajah
K
, Simpson
JM
, van Amerom
JFP
, van Poppel
MPM
, Schulz
A
et al.
Three-dimensional visualisation of the fetal heart using prenatal MRI with motion-corrected slice-volume registration: a prospective, single-centre cohort study
.
Lancet
2019
;
393
:
1619
–
27
.
104Van Poppel
MPM
, Pushparajah
K
, Lloyd
DFA
, Razavi
R
, Speggiorin
S
, Nyman
A
et al.
Insights from fetal cardiac magnetic resonance imaging in double aortic arch
.
Ultrasound Obstet Gynecol
2020
;
56
:
636
–
9
.
105Jansz
MS
, Seed
M
, van Amerom
JFP
, Wong
D
, Grosse-Wortmann
L
, Yoo
S-J
et al.
Metric optimized gating for fetal cardiac MRI
.
Magn Reson Med
2010
;
64
:
1304
–
14
.
106Lloyd
DFA
, van Poppel
MPM
, Pushparajah
K
, Vigneswaran
TV
, Zidere
V
, Steinweg
J
et al.
Analysis of 3-dimensional arch anatomy, vascular flow, and postnatal outcome in cases of suspected coarctation of the aorta using fetal cardiac magnetic resonance imaging
.
Circ Cardiovasc Imaging
2021
;
14
:
e012411
.
107Kording
F
, Yamamura
J
, de Sousa
MT
, Ruprecht
C
, Hedström
E
, Aletras
AH
et al.
Dynamic fetal cardiovascular magnetic resonance imaging using Doppler ultrasound gating
.
J Cardiovasc Magn Reson
2018
;
20
:
17
.
108Salehi
D
, Fricke
K
, Bhat
M
, Arheden
H
, Liuba
P
, Hedström
E
.
Utility of fetal cardiovascular magnetic resonance for prenatal diagnosis of complex congenital heart defects
.
JAMA Netw Open
2021
;
4
:
e213538
.
109Minocha
PK
, Englund
EK
, Friesen
RM
, Fujiwara
T
, Smith
SA
, Meyers
ML
et al.
Reference values for fetal cardiac dimensions, volumes, ventricular function and left ventricular longitudinal strain using Doppler ultrasound gated cardiac magnetic resonance imaging in healthy third trimester fetuses
.
J Magn Reson Imaging
2023
;
60
:
365
–
74
.
110Roberts
TA
, van Amerom
JFP
, Uus
A
, Lloyd
DFA
, van Poppel
MPM
, Price
AN
et al.
Fetal whole heart blood flow imaging using 4D cine MRI
.
Nat Commun
2020
;
11
:
4992
.
111Knapp
J
, Tavares de Sousa
M
, Lenz
A
, Herrmann
J
, Zhang
S
, Kording
F
et al.
Fetal 4D flow MRI of the great thoracic vessels at 3 Tesla using Doppler-ultrasound gating: a feasibility study
.
Eur Radiol
2023
;
33
:
1698
–
706
.
112Saini
BS
, Darby
JRT
, Portnoy
S
, Sun
L
, van Amerom
J
, Lock
MC
et al.
Normal human and sheep fetal vessel oxygen saturations by T2 magnetic resonance imaging
.
J Physiol
2020
;
598
:
3259
–
81
.
113Sun
L
, van Amerom
JFP
, Marini
D
, Portnoy
S
, Lee
FT
, Saini
BS
et al.
MRI characterization of hemodynamic patterns of human fetuses with cyanotic congenital heart disease
.
Ultrasound Obstet Gynecol
2021
;
58
:
824
–
36
.
114Lee
FT
, Marini
D
, Seed
M
, Sun
L
.
Maternal hyperoxygenation in congenital heart disease
.
Transl Pediatr
2021
;
10
:
2197
–
209
.
115Aviles Verdera
J
, Story
L
, Hall
M
, Finck
T
, Egloff
A
, Seed
PT
et al.
Reliability and feasibility of low-field-strength fetal MRI at 0.55 T during pregnancy
.
Radiology
2023
;
309
:
e223050
.
116van den Berg
J
, Wielopolski
PA
, Meijboom
FJ
, Witsenburg
M
, Bogers
AJ
, Pattynama
PM
et al.
Diastolic function in repaired tetralogy of Fallot at rest and during stress: assessment with MR imaging
.
Radiology
2007
;
243
:
212
–
9
.
117Robbers-Visser
D
, Helderman
F
, Strengers
JL
, van Osch-Gevers
L
, Kapusta
L
, Pattynama
PM
et al.
Pulmonary artery size and function after Fontan operation at a young age
.
J Magn Reson Imaging
2008
;
28
:
1101
–
7
.
118Schubert
C
, Brüning
J
, Goubergrits
L
, Hennemuth
A
, Berger
F
, Kühne
T
et al.
Assessment of hemodynamic responses to exercise in aortic coarctation using MRI-ergometry in combination with computational fluid dynamics
.
Sci Rep
2020
;
10
:
18894
.
119Craven
TP
, Tsao
CW
, La Gerche
A
, Simonetti
OP
, Greenwood
JP
.
Exercise cardiovascular magnetic resonance: development, current utility and future applications
.
J Cardiovasc Magn Reson
2020
;
22
:
65
.
120Roest
AA
, Kunz
P
, Lamb
HJ
, Helbing
WA
, van der Wall
EE
, de Roos
A
.
Biventricular response to supine physical exercise in young adults assessed with ultrafast magnetic resonance imaging
.
Am J Cardiol
2001
;
87
:
601
–
5
.
121Oosterhof
T
, Tulevski
II
, Roest
AA
, Steendijk
P
, Vliegen
HW
, van der Wall
EE
et al.
Disparity between dobutamine stress and physical exercise magnetic resonance imaging in patients with an intra-atrial correction for transposition of the great arteries
.
J Cardiovasc Magn Reson
2005
;
7
:
383
–
9
.
122Roest
AA
, Helbing
WA
, Kunz
P
, van den Aardweg
JG
, Lamb
HJ
, Vliegen
HW
et al.
Exercise MR imaging in the assessment of pulmonary regurgitation and biventricular function in patients after tetralogy of fallot repair
.
Radiology
2002
;
223
:
204
–
11
.
123Roest
AA
, Kunz
P
, Helbing
WA
, Lamb
HJ
, Vliegen
HW
, van den Aardweg
JG
et al.
Prolonged cardiac recovery from exercise in asymptomatic adults late after atrial correction of transposition of the great arteries: evaluation with magnetic resonance flow mapping
.
Am J Cardiol
2001
;
88
:
1011
–
7
.
124Dodge-Khatami
A
, Tulevski
II
, Bennink
GB
, Hitchcock
JF
, de Mol
BA
, van der Wall
EE
et al.
Comparable systemic ventricular function in healthy adults and patients with unoperated congenitally corrected transposition using MRI dobutamine stress testing
.
Ann Thorac Surg
2002
;
73
:
1759
–
64
.
125van der Zedde
J
, Oosterhof
T
, Tulevski
II
, Vliegen
HW
, Mulder
BJ
.
Comparison of segmental and global systemic ventricular function at rest and during dobutamine stress between patients with transposition and congenitally corrected transposition
.
Cardiol Young
2005
;
15
:
148
–
53
.
126Tulevski
II
, Lee
PL
, Groenink
M
, van der Wall
EE
, Stoker
J
, Pieper
PG
et al.
Dobutamine-induced increase of right ventricular contractility without increased stroke volume in adolescent patients with transposition of the great arteries: evaluation with magnetic resonance imaging
.
Int J Card Imaging
2000
;
16
:
471
–
8
.
127Tulevski
II
, Hirsch
A
, Dodge-Khatami
A
, Stoker
J
, van der Wall
EE
, Mulder
BJ
.
Effect of pulmonary valve regurgitation on right ventricular function in patients with chronic right ventricular pressure overload
.
Am J Cardiol
2003
;
92
:
113
–
6
.
128Tulevski
I
, van der Wall
EE
, Groenink
M
, Dodge-Khatami
A
, Hirsch
A
, Stoker
J
et al.
Usefulness of magnetic resonance imaging dobutamine stress in asymptomatic and minimally symptomatic patients with decreased cardiac reserve from congenital heart disease (complete and corrected transposition of the great arteries and subpulmonic obstruction)
.
Am J Cardiol
2002
;
89
:
1077
–
81
.
129van den Berg
J
, Strengers
JL
, Wielopolski
PA
, Hop
WC
, Meijboom
FJ
, de Rijke
YB
et al.
Assessment of biventricular functional reserve and NT-proBNP levels in patients with RV volume overload after repair of tetralogy of Fallot at young age
.
Int J Cardiol
2008
;
133
:
364
–
70
.
130Robbers-Visser
D
, Jan ten Harkel
D
, Kapusta
L
, Strengers
JL
, Dalinghaus
M
, Meijboom
FJ
et al.
Usefulness of cardiac magnetic resonance imaging combined with low-dose dobutamine stress to detect an abnormal ventricular stress response in children and young adults after fontan operation at young age
.
Am J Cardiol
2008
;
101
:
1657
–
62
.
131Hjortdal
VE
, Christensen
TD
, Larsen
SH
, Emmertsen
K
, Pedersen
EM
.
Caval blood flow during supine exercise in normal and Fontan patients
.
Ann Thorac Surg
2008
;
85
:
599
–
603
.
132Hjortdal
VE
, Emmertsen
K
, Stenbog
E
, Frund
T
, Schmidt
MR
, Kromann
O
et al.
Effects of exercise and respiration on blood flow in total cavopulmonary connection: a real-time magnetic resonance flow study
.
Circulation
2003
;
108
:
1227
–
31
.
133Pedersen
EM
, Stenbøg
EV
, Fründ
T
, Houlind
K
, Kromann
O
, Sørensen
KE
et al.
Flow during exercise in the total cavopulmonary connection measured by magnetic resonance velocity mapping
.
Heart
2002
;
87
:
554
–
8
.
134Taylor
AM
, Dymarkowski
S
, De Meerleer
K
, Hamaekers
P
, Gewillig
M
, Mertens
L
et al.
Validation and application of single breath-hold cine cardiac MR for ventricular function assessment in children with congenital heart disease at rest and during adenosine stress
.
J Cardiovasc Magn Reson
2005
;
7
:
743
–
51
.
135Fratz
S
, Hager
A
, Busch
R
, Kaemmerer
H
, Schwaiger
M
, Lange
R
et al.
Patients after atrial switch operation for transposition of the great arteries can not increase stroke volume under dobutamine stress as opposed to patients with congenitally corrected transposition
.
Circ J
2008
;
72
:
1130
–
5
.
136Strigl
S
, Beroukhim
R
, Valente
AM
, Annese
D
, Harrington
JS
, Geva
T
et al.
Feasibility of dobutamine stress cardiovascular magnetic resonance imaging in children
.
J Magn Reson Imaging
2009
;
29
:
313
–
9
.
137Winter
MM
, Scherptong
RW
, Kumar
S
, Bouma
BJ
, Tulevski
II
, Tops
LF
et al.
Ventricular response to stress predicts outcome in adult patients with a systemic right ventricle
.
Am Heart J
2010
;
160
:
870
–
6
.
138van den Bosch
E
, Bossers
SSM
, Robbers-Visser
D
, Boersma
E
, Roos-Hesselink
JW
, Breur
H
et al.
Ventricular response to dobutamine stress CMR is a predictor for outcome in Fontan patients
.
JACC Cardiovasc Imaging
2019
;
12
:
368
–
70
.
139van den Bosch
E
, Cuypers
JAAE
, Luijnenburg
SE
, Duppen
N
, Boersma
E
, Budde
RPJ
et al.
Ventricular response to dobutamine stress cardiac magnetic resonance imaging is associated with adverse outcome during 8-year follow-up in patients with repaired tetralogy of Fallot
.
Eur Heart J Cardiovasc Imaging
2020
;
21
:
1039
–
46
.
140Moscatelli
S
, Bianco
F
, Cimini
A
, Panebianco
M
, Leo
I
, Bucciarelli-Ducci
C
et al.
The use of stress cardiovascular imaging in pediatric population
.
Children (Basel)
2023
;
10
:
218
.
141Rickers
C
, Wegner
P
, Silberbach
M
, Madriago
E
, Gabbert
DD
, Kheradvar
A
et al.
Myocardial perfusion in hypoplastic left heart syndrome
.
Circ Cardiovasc Imaging
2021
;
14
:
e012468
.
142Scannell
CM
, Hasaneen
H
, Greil
G
, Hussain
T
, Razavi
R
, Lee
J
et al.
Automated quantitative stress perfusion cardiac magnetic resonance in pediatric patients
.
Front Pediatr
2021
;
9
:
699497
.
143Lafountain
RA
, da Silveira
JS
, Varghese
J
, Mihai
G
, Scandling
D
, Craft
J
et al.
Cardiopulmonary exercise testing in the MRI environment
.
Physiol Meas
2016
;
37
:
N11
–
25
.
144Robbers-Visser
D
, Luijnenburg
SE
, van den Berg
J
, Moelker
A
, Helbing
WA
.
Stress imaging in congenital cardiac disease
.
Cardiol Young
2009
;
19
:
552
–
62
.
145von Knobelsdorff-Brenkenhoff
F
, Dieringer
MA
, Fuchs
K
, Hezel
F
, Niendorf
T
, Schulz-Menger
J
.
Isometric handgrip exercise during cardiovascular magnetic resonance imaging: set-up and cardiovascular effects
.
J Magn Reson Imaging
2013
;
37
:
1342
–
50
.
146Cheng
CP
, Herfkens
RJ
, Lightner
AL
, Taylor
CA
, Feinstein
JA
.
Blood flow conditions in the proximal pulmonary arteries and vena cavae: healthy children during upright cycling exercise
.
Am J Physiol Heart Circ Physiol
2004
;
287
:
H921
–
6
.
147Alpert
BS
, Bloom
KR
, Olley
PM
.
Assessment of left ventricular contractility during supine exercise in children with left-sided cardiac disease
.
Br Heart J
1980
;
44
:
703
–
10
.
148Barber
NJ
, Ako
EO
, Kowalik
GT
, Cheang
MH
, Pandya
B
, Steeden
JA
et al.
Magnetic resonance-augmented cardiopulmonary exercise testing: comprehensively assessing exercise intolerance in children with cardiovascular disease
.
Circ Cardiovasc Imaging
2016
;
9
:
e005282
.
149Hauser
M
, Bengel
FM
, Kühn
A
, Sauer
U
, Zylla
S
, Braun
SL
et al.
Myocardial blood flow and flow reserve after coronary reimplantation in patients after arterial switch and ross operation
.
Circulation
2001
;
103
:
1875
–
80
.
150Kaplan
JD
, Foster
E
, Redberg
RF
, Schiller
NB
.
Exercise Doppler echocardiography identifies abnormal hemodynamics in adults with congenital heart disease
.
Am Heart J
1994
;
127
:
1572
–
80
.
151Cyran
SE
, Grzeszczak
M
, Kaufman
K
, Weber
HS
, Myers
JL
, Gleason
MM
et al.
Aortic “recoarctation” at rest versus at exercise in children as evaluated by stress Doppler echocardiography after a “good” operative result
.
Am J Cardiol
1993
;
71
:
963
–
70
.
152Oyen
EM
, Ingerfeld
G
, Ignatzy
K
, Brode
PE
.
Dynamic exercise echocardiography in children with congenital heart disease affecting the left heart
.
Int J Cardiol
1987
;
17
:
315
–
25
.
153Steenhorst
JJ
, Hirsch
A
, van den Berg
LEM
, Kamphuis
LS
, Merkus
D
, Boersma
E
et al.
Standardizing submaximal exercise intensities for use of supine push-pull exercise during cardiovascular magnetic resonance
.
Clin Physiol Funct Imaging
2023
;
43
:
10
–
9
.
154Pedersen
EM
, Kozerke
S
, Ringgaard
S
, Scheidegger
MB
, Boesiger
P
.
Quantitative abdominal aortic flow measurements at controlled levels of ergometer exercise
.
Magn Reson Imaging
1999
;
17
:
489
–
94
.
155Li
W
, Hornung
TS
, Francis
DP
, O'Sullivan
C
, Duncan
A
, Gatzoulis
M
et al.
Relation of biventricular function quantified by stress echocardiography to cardiopulmonary exercise capacity in adults with Mustard (atrial switch) procedure for transposition of the great arteries
.
Circulation
2004
;
110
:
1380
–
6
.
156Apostolopoulou
SC
, Laskari
CV
, Tsoutsinos
A
, Rammos
S
.
Doppler tissue imaging evaluation of right ventricular function at rest and during dobutamine infusion in patients after repair of tetralogy of Fallot
.
Int J Cardiovasc Imaging
2007
;
23
:
25
–
31
.
157Brili
SV
, Alexopoulos
NA
, Barberis
VI
, Gatzoulis
MA
, Barbetseas
J
, Chrysohoou
C
et al.
Dobutamine stress echocardiography for the evaluation of cardiac reserve late after Fontan operation
.
Hellenic J Cardiol
2007
;
48
:
252
–
7
.
158Stephensen
SS
, Steding-Ehrenborg
K
, Thilén
U
, Holm
J
, Hochbergs
P
, Arheden
H
et al.
Changes in blood volume shunting in patients with atrial septal defects: assessment of heart function with cardiovascular magnetic resonance during dobutamine stress
.
Eur Heart J Cardiovasc Imaging
2017
;
18
:
1145
–
52
.
159Wong
J
, Pushparajah
K
, de Vecchi
A
, Ruijsink
B
, Greil
GF
, Hussain
T
et al.
Pressure-volume loop-derived cardiac indices during dobutamine stress: a step towards understanding limitations in cardiac output in children with hypoplastic left heart syndrome
.
Int J Cardiol
2017
;
230
:
439
–
46
.
160Parish
V
, Valverde
I
, Kutty
S
, Head
C
, Greil
GF
, Schaeffter
T
et al.
Higher dose dobutamine stress MR imaging in repaired tetralogy of Fallot: observer variance of volumetric assessment compared with normal volunteers
.
J Magn Reson Imaging
2013
;
38
:
1356
–
61
.
161Doan
TT
, Molossi
S
, Sachdeva
S
, Wilkinson
JC
, Loar
RW
, Weigand
JD
et al.
Dobutamine stress cardiac MRI is safe and feasible in pediatric patients with anomalous aortic origin of a coronary artery (AAOCA)
.
Int J Cardiol
2021
;
334
:
42
–
8
.
162Robbers-Visser
D
, Luijnenburg
SE
, van den Berg
J
, Roos-Hesselink
JW
, Strengers
JL
, Kapusta
L
et al.
Safety and observer variability of cardiac magnetic resonance imaging combined with low-dose dobutamine stress-testing in patients with complex congenital heart disease
.
Int J Cardiol
2011
;
147
:
214
–
8
.
163Wilkinson
JC
, Doan
TT
, Loar
RW
, Pednekar
AS
, Trivedi
PM
, Masand
PM
et al.
Myocardial stress perfusion MRI using regadenoson: a weight-based approach in infants and young children
.
Radiol Cardiothorac Imaging
2019
;
1
:
e190061
.
164Berthe
C
, Pierard
LA
, Hiernaux
M
, Trotteur
G
, Lempereur
P
, Carlier
J
et al.
Predicting the extent and location of coronary artery disease in acute myocardial infarction by echocardiography during dobutamine infusion
.
Am J Cardiol
1986
;
58
:
1167
–
72
.
165Picano
E
, Lattanzi
F
, Masini
M
, Distante
A
, L'Abbate
A
.
High dose dipyridamole echocardiography test in effort angina pectoris
.
J Am Coll Cardiol
1986
;
8
:
848
–
54
.
166Lanser
CNG
, van Poecke
WHA
, Scheffers
LE
, van den Berg
LE
, Helbing
WA
.
Stress imaging in patients with a Fontan circulation: a systematic review
.
Int J Cardiol
2023
;
391
:
131192
.
167Barber
NJ
, Ako
EO
, Kowalik
GT
, Steeden
JA
, Pandya
B
, Muthurangu
V
.
MR augmented cardiopulmonary exercise testing-a novel approach to assessing cardiovascular function
.
Physiol Meas
2015
;
36
:
N85
–
94
.
168La Gerche
A
, Claessen
G
, Van de Bruaene
A
, Pattyn
N
, Van Cleemput
J
, Gewillig
M
et al.
Cardiac MRI: a new gold standard for ventricular volume quantification during high-intensity exercise
.
Circ Cardiovasc Imaging
2013
;
6
:
329
–
38
.
169Razavi
R
, Hill
DL
, Keevil
SF
, Miquel
ME
, Muthurangu
V
, Hegde
S
et al.
Cardiac catheterisation guided by MRI in children and adults with congenital heart disease
.
Lancet
2003
;
362
:
1877
–
82
.
170Muthurangu
V
, Taylor
A
, Andriantsimiavona
R
, Hegde
S
, Miquel
ME
, Tulloh
R
et al.
Novel method of quantifying pulmonary vascular resistance by use of simultaneous invasive pressure monitoring and phase-contrast magnetic resonance flow
.
Circulation
2004
;
110
:
826
–
34
.
171Di Salvo
G
, Miller
O
, Babu Narayan
S
, Li
W
, Budts
W
, Valsangiacomo Buechel
ER
et al.
Imaging the adult with congenital heart disease: a multimodality imaging approach-position paper from the EACVI
.
Eur Heart J Cardiovasc Imaging
2018
;
19
:
1077
–
98
.
172Velasco Forte
MN
, Pushparajah
K
, Schaeffter
T
, Valverde Perez
I
, Rhode
K
, Ruijsink
B
et al.
Improved passive catheter tracking with positive contrast for CMR-guided cardiac catheterization using partial saturation (pSAT)
.
J Cardiovasc Magn Reson
2017
;
19
:
60
.
173Velasco Forte
MN
, Roujol
S
, Ruijsink
B
, Valverde
I
, Duong
P
, Byrne
N
et al.
MRI for guided right and left heart cardiac catheterization: a prospective study in congenital heart disease
.
J Magn Reson Imaging
2021
;
53
:
1446
–
57
.
174Knight
DS
, Kotecha
T
, Martinez-Naharro
A
, Brown
JT
, Bertelli
M
, Fontana
M
et al.
Cardiovascular magnetic resonance-guided right heart catheterization in a conventional CMR environment—predictors of procedure success and duration in pulmonary artery hypertension
.
J Cardiovasc Magn Reson
2019
;
21
:
57
.
175Nageotte
SJ
, Lederman
RJ
, Ratnayaka
K
.
MRI catheterization: ready for broad adoption
.
Pediatr Cardiol
2020
;
41
:
503
–
13
.
176Veeram Reddy
SR
, Arar
Y
, Zahr
RA
, Gooty
V
, Hernandez
J
, Potersnak
A
et al.
Invasive cardiovascular magnetic resonance (iCMR) for diagnostic right and left heart catheterization using an MR-conditional guidewire and passive visualization in congenital heart disease
.
J Cardiovasc Magn Reson
2020
;
22
:
20
.
177Pushparajah
K
, Wong
JK
, Bellsham-Revell
HR
, Hussain
T
, Valverde
I
, Bell
A
et al.
Magnetic resonance imaging catheter stress haemodynamics post-Fontan in hypoplastic left heart syndrome
.
Eur Heart J Cardiovasc Imaging
2016
;
17
:
644
–
51
.
178Chubb
H
, Harrison
JL
, Weiss
S
, Krueger
S
, Koken
P
, Bloch
L
et al.
Development, preclinical validation, and clinical translation of a cardiac magnetic resonance—electrophysiology system with active catheter tracking for ablation of cardiac arrhythmia
.
JACC Clin Electrophysiol
2017
;
3
:
89
–
103
.
179Chubb
H
, Williams
SE
, Whitaker
J
, Harrison
JL
, Razavi
R
, O'Neill
M
.
Cardiac electrophysiology under MRI guidance: an emerging technology
.
Arrhythm Electrophysiol Rev
2017
;
6
:
85
–
93
.
180Hilbert
S
, Sommer
P
, Gutberlet
M
, Gaspar
T
, Foldyna
B
, Piorkowski
C
et al.
Real-time magnetic resonance-guided ablation of typical right atrial flutter using a combination of active catheter tracking and passive catheter visualization in man: initial results from a consecutive patient series
.
Europace
2016
;
18
:
572
–
7
.
181Bijvoet
GP
, Nies
H
, Holtackers
RJ
, Martens
BM
, Smink
J
, Linz
D
et al.
Tissue characterization of acute lesions during cardiac magnetic resonance-guided ablation of cavo-tricuspid isthmus-dependent atrial flutter: a feasibility study
.
Eur Heart J Cardiovasc Imaging
2023
;
24
:
jead119.415
.
182Massmann
A
, Buecker
A
, Schneider
GK
.
Glass-Fiber-based MR-safe guidewire for MR imaging-guided endovascular interventions: in vitro and preclinical in vivo feasibility study
.
Radiology
2017
;
284
:
541
–
51
.
183Tzifa
A
, Krombach
GA
, Krämer
N
, Krüger
S
, Schütte
A
, von Walter
M
et al.
Magnetic resonance-guided cardiac interventions using magnetic resonance-compatible devices: a preclinical study and first-in-man congenital interventions
.
Circ Cardiovasc Interv
2010
;
3
:
585
–
92
.
184Amin
EK
, Campbell-Washburn
A
, Ratnayaka
K
.
MRI-guided cardiac catheterization in congenital heart disease: how to get started
.
Curr Cardiol Rep
2022
;
24
:
419
–
29
.
185Pushparajah
K
, Chubb
H
, Razavi
R
.
MR-guided cardiac interventions
.
Top Magn Reson Imaging
2018
;
27
:
115
–
28
.
186Hori
M
, Hagiwara
A
, Goto
M
, Wada
A
, Aoki
S
.
Low-Field magnetic resonance imaging: its history and renaissance
.
Invest Radiol
2021
;
56
:
669
–
79
.
187Makowski
MR
, Wiethoff
AJ
, Uribe
S
, Parish
V
, Botnar
RM
, Bell
A
et al.
Congenital heart disease: cardiovascular MR imaging by using an intravascular blood pool contrast agent
.
Radiology
2011
;
260
:
680
–
8
.
188Dedieu
N
, Lossnitzer
D
, Makowski
MR
, Vieira
MSN
, Hussain
T
, Wong
J
et al.
Coronary artery imaging in patients with congenital heart disease: improved image quality using an intravascular contrast agent and specific magnetic resonance sequence design
.
Clin Med Rev Case Rep
2016
;
3
:
92
–
7
.
189Makowski
MR
, Wiethoff
AJ
, Jansen
CH
, Uribe
S
, Parish
V
, Schuster
A
et al.
Single breath-hold assessment of cardiac function using an accelerated 3D single breath-hold acquisition technique–comparison of an intravascular and extravascular contrast agent
.
J Cardiovasc Magn Reson
2012
;
14
:
53
.
190Yoshida
T
, Chen
JJ
, Zhou
B
, Finn
JP
, Hu
P
, Nguyen
KL
.
Ferumoxytol-enhanced 4D multiphase, steady-state imaging with magnetic resonance in congenital heart disease: ventricular volume and function across 2D and 3D software platforms
.
Quant Imaging Med Surg
2022
;
12
:
4377
–
89
.
191Makowski
MR
, Wiethoff
AJ
, Ebersberger
HU
, Botnar
RM
, Razavi
R
, Schaeffter
T
et al.
2D phase contrast blood flow velocity measurements of the thoracic vasculature: comparison of the effect of gadofosveset trisodium and gadopentetate dimeglumine
.
Int J Cardiovasc Imaging
2015
;
31
:
409
–
16
.
192Renella
P
, Li
J
, Prosper
AE
, Finn
JP
, Nguyen
KL
.
Ferumoxytol-Enhanced cardiac magnetic resonance angiography and 4D flow: safety and utility in pediatric and adult congenital heart disease
.
Children (Basel)
2022
;
9
:
1810
.
193Kollar
SE
, Udine
ML
, Mandell
JG
, Cross
RR
, Loke
YH
, Olivieri
LJ
.
Impact of ferumoxytol vs gadolinium on 4D flow cardiovascular magnetic resonance measurements in small children with congenital heart disease
.
J Cardiovasc Magn Reson
2022
;
24
:
58
.
194Stirrat
CG
, Alam
SR
, MacGillivray
TJ
, Gray
CD
, Dweck
MR
, Raftis
J
et al.
Ferumoxytol-enhanced magnetic resonance imaging assessing inflammation after myocardial infarction
.
Heart
2017
;
103
:
1528
–
35
.
195Ahmad
F
, Treanor
L
, McGrath
TA
, Walker
D
, McInnes
MDF
, Schieda
N
.
Safety of off-label use of ferumoxtyol as a contrast agent for MRI: a systematic review and meta-analysis of adverse events
.
J Magn Reson Imaging
2021
;
53
:
840
–
58
.
196Trusty
PM
, Slesnick
TC
, Wei
ZA
, Rossignac
J
, Kanter
KR
, Fogel
MA
et al.
Fontan surgical planning: previous accomplishments, current challenges, and future directions
.
J Cardiovasc Transl Res
2018
;
11
:
133
–
44
.
197Rijnberg
FM
, van ‘t Hul
LC
, Hazekamp
MG
, van den Boogaard
PJ
, Juffermans
JF
, Lamb
HJ
et al.
Haemodynamic performance of 16–20-mm extracardiac Goretex conduits in adolescent Fontan patients at rest and during simulated exercise
.
Eur J Cardiothorac Surg
2022
;
63
:.
198LaDisa
JF
Jr, Alberto Figueroa
C
, Vignon-Clementel
IE
, Kim
HJ
, Xiao
N
, Ellwein
LM
et al.
Computational simulations for aortic coarctation: representative results from a sampling of patients
.
J Biomech Eng
2011
;
133
:
091008
.
199Pons
R
, Guala
A
, Rodríguez-Palomares
JF
, Cajas
JC
, Dux-Santoy
L
, Teixidó-Tura
G
et al.
Fluid-structure interaction simulations outperform computational fluid dynamics in the description of thoracic aorta haemodynamics and in the differentiation of progressive dilation in Marfan syndrome patients
.
R Soc Open Sci
2020
;
7
:
191752
.
200Frieberg
P
, Aristokleous
N
, Sjöberg
P
, Töger
J
, Liuba
P
, Carlsson
M
.
Computational fluid dynamics support for Fontan planning in minutes, not hours: the next step in clinical pre-interventional simulations
.
J Cardiovasc Transl Res
2022
;
15
:
708
–
20
.
201Hauptmann
A
, Arridge
S
, Lucka
F
, Muthurangu
V
, Steeden
JA
.
Real-time cardiovascular MR with spatio-temporal artifact suppression using deep learning-proof of concept in congenital heart disease
.
Magn Reson Med
2019
;
81
:
1143
–
56
.
202Zucker
EJ
, Sandino
CM
, Kino
A
, Lai
P
, Vasanawala
SS
.
Free-breathing accelerated cardiac MRI using deep learning: validation in children and young adults
.
Radiology
2021
;
300
:
539
–
48
.
203Jaubert
O
, Steeden
J
, Montalt-Tordera
J
, Arridge
S
, Kowalik
GT
, Muthurangu
V
.
Deep artifact suppression for spiral real-time phase contrast cardiac magnetic resonance imaging in congenital heart disease
.
Magn Reson Imaging
2021
;
83
:
125
–
32
.
204Oscanoa
JA
, Middione
MJ
, Syed
AB
, Sandino
CM
, Vasanawala
SS
, Ennis
DB
.
Accelerated two-dimensional phase-contrast for cardiovascular MRI using deep learning-based reconstruction with complex difference estimation
.
Magn Reson Med
2023
;
89
:
356
–
69
.
205Qi
H
, Hajhosseiny
R
, Cruz
G
, Kuestner
T
, Kunze
K
, Neji
R
et al.
End-to-end deep learning nonrigid motion-corrected reconstruction for highly accelerated free-breathing coronary MRA
.
Magn Reson Med
2021
;
86
:
1983
–
96
.
206Steeden
JA
, Quail
M
, Gotschy
A
, Mortensen
KH
, Hauptmann
A
, Arridge
S
et al.
Rapid whole-heart CMR with single volume super-resolution
.
J Cardiovasc Magn Reson
2020
;
22
:
56
.
207Karimi-Bidhendi
S
, Arafati
A
, Cheng
AL
, Wu
Y
, Kheradvar
A
, Jafarkhani
H
.
Fully-automated deep-learning segmentation of pediatric cardiovascular magnetic resonance of patients with complex congenital heart diseases
.
J Cardiovasc Magn Reson
2020
;
22
:
80
.
208Govil
S
, Crabb
BT
, Deng
Y
, Dal Toso
L
, Puyol-Antón
E
, Pushparajah
K
et al.
A deep learning approach for fully automated cardiac shape modeling in tetralogy of Fallot
.
J Cardiovasc Magn Reson
2023
;
25
:
15
.
209Montalt-Tordera
J
, Pajaziti
E
, Jones
R
, Sauvage
E
, Puranik
R
, Singh
AAV
et al.
Automatic segmentation of the great arteries for computational hemodynamic assessment
.
J Cardiovasc Magn Reson
2022
;
24
:
57
.
210Hagdorn
QAJ
, Vos
JDL
, Beurskens
NEG
, Gorter
TM
, Meyer
SL
, van Melle
JP
et al.
CMR feature tracking left ventricular strain-rate predicts ventricular tachyarrhythmia, but not deterioration of ventricular function in patients with repaired tetralogy of Fallot
.
Int J Cardiol
2019
;
295
:
1
–
6
.
211Kammerlander
AA
, Kraiger
JA
, Nitsche
C
, Donà
C
, Duca
F
, Zotter-Tufaro
C
et al.
Global longitudinal strain by CMR feature tracking is associated with outcome in HFPEF
.
JACC Cardiovasc Imaging
2019
;
12
(
8 Pt 1
):
1585
–
7
.
212Romano
S
, Judd
RM
, Kim
RJ
, Heitner
JF
, Shah
DJ
, Shenoy
C
et al.
Feature-Tracking global longitudinal strain predicts mortality in patients with preserved ejection fraction: a multicenter study
.
JACC Cardiovasc Imaging
2020
;
13
:
940
–
7
.
213Pedrizzetti
G
, Claus
P
, Kilner
PJ
, Nagel
E
.
Principles of cardiovascular magnetic resonance feature tracking and echocardiographic speckle tracking for informed clinical use
.
J Cardiovasc Magn Reson
2016
;
18
:
51
.
214Hor
KN
, Baumann
R
, Pedrizzetti
G
, Tonti
G
, Gottliebson
WM
, Taylor
M
et al.
Magnetic resonance derived myocardial strain assessment using feature tracking
.
J Vis Exp
2011
:
2356
.
215Kutty
S
, Rangamani
S
, Venkataraman
J
, Li
L
, Schuster
A
, Fletcher
SE
et al.
Reduced global longitudinal and radial strain with normal left ventricular ejection fraction late after effective repair of aortic coarctation: a CMR feature tracking study
.
Int J Cardiovasc Imaging
2013
;
29
:
141
–
50
.
216Kawel-Boehm
N
, Hetzel
SJ
, Ambale-Venkatesh
B
, Captur
G
, Francois
CJ
, Jerosch-Herold
M
et al.
Reference ranges (“normal values”) for cardiovascular magnetic resonance (CMR) in adults and children: 2020 update
.
J Cardiovasc Magn Reson
2020
;
22
:
87
.
217André
F
, Robbers-Visser
D
, Helling-Bakki
A
, Föll
A
, Voss
A
, Katus
HA
et al.
Quantification of myocardial deformation in children by cardiovascular magnetic resonance feature tracking: determination of reference values for left ventricular strain and strain rate
.
J Cardiovasc Magn Reson
2016
;
19
:
8
.
218Shang
Q
, Patel
S
, Steinmetz
M
, Schuster
A
, Danford
DA
, Beerbaum
P
et al.
Myocardial deformation assessed by longitudinal strain: chamber specific normative data for CMR-feature tracking from the German competence network for congenital heart defects
.
Eur Radiol
2018
;
28
:
1257
–
66
.
219Voges
I
, Negwer
I
, Caliebe
A
, Boroni Grazioli
S
, Daubeney
PEF
, Uebing
A
et al.
Myocardial deformation in the pediatric age group: normal values for strain and strain rate using 2D magnetic resonance feature tracking
.
J Magn Reson Imaging
2022
;
56
:
1382
–
92
.
220Obokata
M
, Nagata
Y
, Wu
VC
, Kado
Y
, Kurabayashi
M
, Otsuji
Y
et al.
Direct comparison of cardiac magnetic resonance feature tracking and 2D/3D echocardiography speckle tracking for evaluation of global left ventricular strain
.
Eur Heart J Cardiovasc Imaging
2016
;
17
:
525
–
32
.
221Bourfiss
M
, Vigneault
DM
, Aliyari Ghasebeh
M
, Murray
B
, James
CA
, Tichnell
C
et al.
Feature tracking CMR reveals abnormal strain in preclinical arrhythmogenic right ventricular dysplasia/cardiomyopathy: a multisoftware feasibility and clinical implementation study
.
J Cardiovasc Magn Reson
2017
;
19
:
66
.
222Dobrovie
M
, Barreiro-Pérez
M
, Curione
D
, Symons
R
, Claus
P
, Voigt
JU
et al.
Inter-vendor reproducibility and accuracy of segmental left ventricular strain measurements using CMR feature tracking
.
Eur Radiol
2019
;
29
:
6846
–
57
.
223Yang
W
, Xu
J
, Zhu
L
, Zhang
Q
, Wang
Y
, Zhao
S
et al.
Myocardial strain measurements derived from MR feature-tracking: influence of sex, age, field strength, and vendor
.
JACC Cardiovasc Imaging
2023
;
17
:
364
–
79
.
224Amzulescu
MS
, De Craene
M
, Langet
H
, Pasquet
A
, Vancraeynest
D
, Pouleur
AC
et al.
Myocardial strain imaging: review of general principles, validation, and sources of discrepancies
.
Eur Heart J Cardiovasc Imaging
2019
;
20
:
605
–
19
.
225Ghonim
S
, Voges
I
, Gatehouse
PD
, Keegan
J
, Gatzoulis
MA
, Kilner
PJ
et al.
Myocardial architecture, mechanics, and fibrosis in congenital heart disease
.
Front Cardiovasc Med
2017
;
4
:
30
.
226Hasan
H
, Chouvarine
P
, Diekmann
F
, Diedrich
N
, Koestenberger
M
, Hansmann
G
.
Validation of the new paediatric pulmonary hypertension risk score by CMR and speckle tracking echocardiography
.
Eur J Clin Invest
2022
;
52
:
e13835
.
227Vigneault
DM
, Yang
E
, Jensen
PJ
, Tee
MW
, Farhad
H
, Chu
L
et al.
Left ventricular strain is abnormal in preclinical and overt hypertrophic cardiomyopathy: cardiac MR feature tracking
.
Radiology
2019
;
290
:
640
–
8
.
228Pi
SH
, Kim
SM
, Choi
JO
, Kim
EK
, Chang
SA
, Choe
YH
et al.
Prognostic value of myocardial strain and late gadolinium enhancement on cardiovascular magnetic resonance imaging in patients with idiopathic dilated cardiomyopathy with moderate to severely reduced ejection fraction
.
J Cardiovasc Magn Reson
2018
;
20
:
36
.
229Siegel
B
, Olivieri
L
, Gordish-Dressman
H
, Spurney
CF
.
Myocardial strain using cardiac MR feature tracking and speckle tracking echocardiography in Duchenne muscular dystrophy patients
.
Pediatr Cardiol
2018
;
39
:
478
–
83
.
230Dardeer
AM
, Hudsmith
L
, Wesolowski
R
, Clift
P
, Steeds
RP
.
The potential role of feature tracking in adult congenital heart disease: advantages and disadvantages in measuring myocardial deformation by cardiovascular magnetic resonance
.
J Congenital Cardiol
2018
;
2
:
3
.
231Latus
H
, Stammermann
J
, Voges
I
, Waschulzik
B
, Gutberlet
M
, Diller
GP
et al.
Impact of right ventricular pressure load after repair of tetralogy of Fallot
.
J Am Heart Assoc
2022
;
11
:
e022694
.
232Orwat
S
, Diller
GP
, Kempny
A
, Radke
R
, Peters
B
, Kühne
T
et al.
Myocardial deformation parameters predict outcome in patients with repaired tetralogy of Fallot
.
Heart
2016
;
102
:
209
–
15
.
233Masutani
EM
, Chandrupatla
RS
, Wang
S
, Zocchi
C
, Hahn
LD
, Horowitz
M
et al.
Deep learning synthetic strain: quantitative assessment of regional myocardial wall motion at MRI
.
Radiol Cardiothorac Imaging
2023
;
5
:
e220202
.
234Krupickova
S
, Bautista-Rodriguez
C
, Hatipoglu
S
, Kang
H
, Fraisse
A
, Di Salvo
G
et al.
Myocardial deformation assessed by CMR in children after multisystem inflammatory syndrome (MIS-C)
.
Int J Cardiol
2022
;
346
:
105
–
6
.
235Sunagawa
K
, Maughan
WL
, Burkhoff
D
, Sagawa
K
.
Left ventricular interaction with arterial load studied in isolated canine ventricle
.
Am J Physiol
1983
;
245
(
5 Pt 1
):
H773
–
80
.
236Kubota
T
, Alexander
J
Jr, Itaya
R
, Todaka
K
, Sugimachi
M
, Sunagawa
K
et al.
Dynamic effects of carotid sinus baroreflex on ventriculoarterial coupling studied in anesthetized dogs
.
Circ Res
1992
;
70
:
1044
–
53
.
237Yokoyama
H
, Yamanaka
F
, Shishido
K
, Ochiai
T
, Yokota
S
, Moriyama
N
et al.
Prognostic value of ventricular-arterial coupling after transcatheter aortic valve replacement on midterm clinical outcomes
.
J Am Heart Assoc
2021
;
10
:
e019267
.
238Hsu
S
, Simpson
CE
, Houston
BA
, Wand
A
, Sato
T
, Kolb
TM
et al.
Multi-beat right ventricular-arterial coupling predicts clinical worsening in pulmonary arterial hypertension
.
J Am Heart Assoc
2020
;
9
:
e016031
.
239Chen
CH
, Fetics
B
, Nevo
E
, Rochitte
CE
, Chiou
KR
, Ding
PA
et al.
Noninvasive single-beat determination of left ventricular end-systolic elastance in humans
.
J Am Coll Cardiol
2001
;
38
:
2028
–
34
.
240Ky
B
, French
B
, May Khan
A
, Plappert
T
, Wang
A
, Chirinos
JA
et al.
Ventricular-arterial coupling, remodeling, and prognosis in chronic heart failure
.
J Am Coll Cardiol
2013
;
62
:
1165
–
72
.
241Egbe
AC
, Anavekar
NS
, Connolly
HM
.
Abnormal pulmonary arterial elastance is associated with reduced exercise capacity in tetralogy of Fallot
.
J Am Heart Assoc
2019
;
8
:
e011731
.
242Sanz
J
, García-Alvarez
A
, Fernández-Friera
L
, Nair
A
, Mirelis
JG
, Sawit
ST
et al.
Right ventriculo-arterial coupling in pulmonary hypertension: a magnetic resonance study
.
Heart
2012
;
98
:
238
–
43
.
243Sandeep
B
, Huang
X
, Li
Y
, Wang
X
, Mao
L
, Kan
Y
et al.
Evaluation of right ventricle pulmonary artery coupling on right ventricular function in post operative tetralogy of Fallot patients underwent for pulmonary valve replacement
.
J Cardiothorac Surg
2020
;
15
:
241
.
244Panaioli
E
, Birritella
L
, Graziani
F
, Lillo
R
, Grandinetti
M
, Di Molfetta
A
et al.
Right ventricle-pulmonary artery coupling in repaired tetralogy of Fallot with pulmonary regurgitation: clinical implications
.
Arch Cardiovasc Dis
2022
;
115
:
67
–
77
.
245Aubert
R
, Venner
C
, Huttin
O
, Haine
D
, Filippetti
L
, Guillaumot
A
et al.
Three-Dimensional echocardiography for the assessment of right ventriculo-arterial coupling
.
J Am Soc Echocardiogr
2018
;
31
:
905
–
15
.
246Nakaya
T
, Ohira
H
, Sato
T
, Watanabe
T
, Nishimura
M
, Oyama-Manabe
N
et al.
Right ventriculo–pulmonary arterial uncoupling and poor outcomes in pulmonary arterial hypertension
.
Pulm Circ
2020
;
10
:.
247Vanderpool
RR
, Pinsky
MR
, Naeije
R
, Deible
C
, Kosaraju
V
, Bunner
C
et al.
RV-pulmonary arterial coupling predicts outcome in patients referred for pulmonary hypertension
.
Heart
2015
;
101
:
37
–
43
.
248Ikonomidis
I
, Aboyans
V
, Blacher
J
, Brodmann
M
, Brutsaert
DL
, Chirinos
JA
et al.
The role of ventricular–arterial coupling in cardiac disease and heart failure: assessment, clinical implications and therapeutic interventions. A consensus document of the European Society of Cardiology Working Group on Aorta & Peripheral Vascular Diseases, European Association of Cardiovascular Imaging, and Heart Failure Association
.
Eur J Heart Fail
2019
;
21
:
402
–
24
.
249Arya
N
, Schievano
S
, Caputo
M
, Taylor
AM
, Biglino
G
.
Relationship between pulmonary regurgitation and ventriculo-arterial interactions in patients with post-early repair of tetralogy of Fallot: insights from wave-intensity analysis
.
J Clin Med
2022
;
11
:
6186
.
250Milano
EG
, Neumann
S
, Sophocleous
F
, Pontecorboli
G
, Curtis
SL
, Bedair
R
et al.
Wave reflection and ventriculo-arterial coupling in bicuspid aortic valve patients with repaired aortic coarctation
.
Front Pediatr
2021
;
9
:
770754
.
251Quail
MA
, Short
R
, Pandya
B
, Steeden
JA
, Khushnood
A
, Taylor
AM
et al.
Abnormal wave reflections and left ventricular hypertrophy late after coarctation of the aorta repair
.
Hypertension
2017
;
69
:
501
–
9
.
252Schäfer
M
, Frank
BS
, Jacobsen
R
, Rausch
CM
, Mitchell
MB
, Jaggers
J
et al.
Patients with Fontan circulation have abnormal aortic wave propagation patterns: a wave intensity analysis study
.
Int J Cardiol
2021
;
322
:
158
–
67
.
253Schäfer
M
, Wilson
N
, Ivy
DD
, Ing
R
, Abman
S
, Browne
LP
et al.
Noninvasive wave intensity analysis predicts functional worsening in children with pulmonary arterial hypertension
.
Am J Physiol Heart Circ Physiol
2018
;
315
:
H968
–
H77
.
254Ikonomidis
I
, Katsanos
S
, Triantafyllidi
H
, Parissis
J
, Tzortzis
S
, Pavlidis
G
et al.
Pulse wave velocity to global longitudinal strain ratio in hypertension
.
Eur J Clin Invest
2019
;
49
:
e13049
.
255Illmann
CF
, Ghadiry-Tavi
R
, Hosking
M
, Harris
KC
.
Utility of 3D printed cardiac models in congenital heart disease: a scoping review
.
Heart
2020
;
106
:
1631
–
7
.
256Valverde
I
, Gomez-Ciriza
G
, Hussain
T
, Suarez-Mejias
C
, Velasco-Forte
MN
, Byrne
N
et al.
Three-dimensional printed models for surgical planning of complex congenital heart defects: an international multicentre study
.
Eur J Cardiothorac Surg
2017
;
52
:
1139
–
48
.
257Giannopoulos
AA
, Mitsouras
D
, Yoo
S-J
, Liu
PP
, Chatzizisis
YS
, Rybicki
FJ
.
Applications of 3D printing in cardiovascular diseases
.
Nat Rev Cardiol
2016
;
13
:
701
–
18
.
258Celi
S
, Gasparotti
E
, Capellini
K
, Vignali
E
, Fanni
BM
, Ali
LA
et al.
3D printing in modern cardiology
.
Curr Pharm Des
2021
;
27
:
1918
–
30
.
259Fotaki
A
, Pushparajah
K
, Hajhosseiny
R
, Schneider
A
, Alam
H
, Ferreira
J
et al.
Free-breathing, contrast agent-free whole-heart MTC-BOOST imaging: single-center validation study in adult congenital heart disease
.
Radiol Cardiothorac Imaging
2023
;
5
:
e220146
.
260Fotaki
A
, Munoz
C
, Emanuel
Y
, Hua
A
, Bosio
F
, Kunze
KP
et al.
Efficient non-contrast enhanced 3D Cartesian cardiovascular magnetic resonance angiography of the thoracic aorta in 3 min
.
J Cardiovasc Magn Reson
2022
;
24
:
5
.
261Yoo
S-J
, Thabit
O
, Kim
EK
, Ide
H
, Yim
D
, Dragulescu
A
et al.
3D printing in medicine of congenital heart diseases
.
3D Print Med
2016
;
2
:
3
.
262Townsend
K
, Pietila
T
.
3D printing and modeling of congenital heart defects: a technical review
.
Birth Defects Res
2018
;
110
:
1091
–
7
.
263Vigil
C
, Lasso
A
, Ghosh
RM
, Pinter
C
, Cianciulli
A
, Nam
HH
et al.
Modeling tool for rapid virtual planning of the intracardiac baffle in double-outlet right ventricle
.
Ann Thorac Surg
2021
;
111
:
2078
–
83
.
264Ghosh
RM
, Jolley
MA
, Mascio
CE
, Chen
JM
, Fuller
S
, Rome
JJ
et al.
Clinical 3D modeling to guide pediatric cardiothoracic surgery and intervention using 3D printed anatomic models, computer aided design and virtual reality
.
3D Print Med
2022
;
8
:
11
.
265Bertelli
F
, Raimondi
F
, Godard
C
, Bergonzoni
E
, Cattapan
C
, Gastino
E
et al.
Fast-track virtual reality software to facilitate 3-dimensional reconstruction in congenital heart disease
.
Interdiscip Cardiovasc Thorac Surg
2023
;
36
:
ivad087
.
266Raimondi
F
, Vida
V
, Godard
C
, Bertelli
F
, Reffo
E
, Boddaert
N
et al.
Fast-track virtual reality for cardiac imaging in congenital heart disease
.
J Card Surg
2021
;
36
:
2598
–
602
.
267Jung
C
, Wolff
G
, Wernly
B
, Bruno
RR
, Franz
M
, Schulze
PC
et al.
Virtual and augmented reality in cardiovascular care: state-of-the-art and future perspectives
.
JACC Cardiovasc Imaging
2022
;
15
:
519
–
32
.
268Awori
J
, Friedman
SD
, Howard
C
, Kronmal
R
, Buddhe
S
.
Comparative effectiveness of virtual reality (VR) vs 3D printed models of congenital heart disease in resident and nurse practitioner educational experience
.
3D Print Med
2023
;
9
:
2
.
269Beams
R
, Brown
E
, Cheng
WC
, Joyner
JS
, Kim
AS
, Kontson
K
et al.
Evaluation challenges for the application of extended reality devices in medicine
.
J Digit Imaging
2022
;
35
:
1409
–
18
.
Author notes
© The Author(s) 2024. Published by Oxford University Press on behalf of the European Society of Cardiology. All rights reserved. For commercial re-use, please contact
[email protected] for reprints and translation rights for reprints. All other permissions can be obtained through our RightsLink service via the Permissions link on the article page on our site—for further information please contact
[email protected].