-
PDF
- Split View
-
Views
-
Cite
Cite
Reece Parry, Kamran Majeed, Fiona Pixley, Graham Scott Hillis, Roslyn Jane Francis, Carl Johann Schultz, Unravelling the role of macrophages in cardiovascular inflammation through imaging: a state-of-the-art review, European Heart Journal - Cardiovascular Imaging, Volume 23, Issue 12, December 2022, Pages e504–e525, https://doi.org/10.1093/ehjci/jeac167
- Share Icon Share
Abstract
Cardiovascular disease remains the leading cause of death and disability for patients across the world. Our understanding of atherosclerosis as a primary cholesterol issue has diversified, with a significant dysregulated inflammatory component that largely remains untreated and continues to drive persistent cardiovascular risk. Macrophages are central to atherosclerotic inflammation, and they exist along a functional spectrum between pro-inflammatory and anti-inflammatory extremes. Recent clinical trials have demonstrated a reduction in major cardiovascular events with some, but not all, anti-inflammatory therapies. The recent addition of colchicine to societal guidelines for the prevention of recurrent cardiovascular events in high-risk patients with chronic coronary syndromes highlights the real-world utility of this class of therapies. A highly targeted approach to modification of interleukin-1-dependent pathways shows promise with several novel agents in development, although excessive immunosuppression and resulting serious infection have proven a barrier to implementation into clinical practice. Current risk stratification tools to identify high-risk patients for secondary prevention are either inadequately robust or prohibitively expensive and invasive. A non-invasive and relatively inexpensive method to identify patients who will benefit most from novel anti-inflammatory therapies is required, a role likely to be fulfilled by functional imaging methods. This review article outlines our current understanding of the inflammatory biology of atherosclerosis, upcoming therapies and recent landmark clinical trials, imaging modalities (both invasive and non-invasive) and the current landscape surrounding functional imaging including through targeted nuclear and nanobody tracer development and their application.
Introduction
Despite significant advances in the detection and treatment of cardiovascular disease, it remains the leading cause of death worldwide.1 The short- and long-term implications are wide-ranging—if a patient survives an acute coronary syndrome (ACS) there is a reduction in quality of life, increased risk of death, heart failure and arrhythmia, and a more than two-fold increased risk of mental health disorders including depression.2–7 Despite receiving optimal preventative therapies based on current evidence, up to 25% of patients have a repeated cardiovascular event within 5 years.8 Both primary and residual cardiovascular risks are driven by unmitigated thrombotic, inflammatory, and metabolic perturbations.
Atherosclerosis is a chronic inflammatory disease involving medium- and large-sized arteries that results from a diverse number of cellular and molecular processes. Tissue-resident macrophages occupy a central role in the maintenance of tissue integrity and healing where they switch between pro- and anti-inflammatory functions as required.9 When pathological stimuli modulate macrophage function, the subsequent loss of tissue homeostasis leads to a persistent inflammatory response and progressive injury. Advances in imaging continue to expand upon our understanding of the dysfunctional macrophage in cardiovascular disease. In this review, we discuss the role of macrophages in atherosclerosis, and imaging modalities used for studying macrophages with an emphasis on translation to in-human imaging and potential therapies.
Macrophages and the developing plaque
Recent macrophage studies in both animal and human models report a considerable functional spectrum between the classical extremes of M1 (pro-inflammatory) and M2 (anti-inflammatory) phenotypes.10–12 In healthy arteries and during the early fatty-streak phase of atherosclerosis, macrophages polarize towards the largely M2-like phenotype.13 As atherosclerosis progresses and cholesterol crystals accumulate, incoming monocyte-derived macrophages and tissue macrophages adopt an M1-like phenotype on exposure to lipid-derived metabolites such as oxidized low-density lipoprotein (LDL), with activation of nuclear factor-κB, up-regulation of endothelial cell adhesion molecules and release of monocyte and macrophage chemokines such as chemokine (C–C motif) ligand 2 and colony-stimulating factor 1.12,14,15 These ‘activated’ macrophages upregulate inflammatory metabolic pathways leading to activation of the NLR family pyrin domain containing 3 (NLRP3) inflammasome, succinylation of intra-cellular proteins (including hypoxia-inducible factor-alpha), and release of interleukin-1β (IL-1β).16 Cytokines also attract other circulating immune cells such as neutrophils which scavenge, oxidize, and attempt to clear lipoproteins and other pro-inflammatory crystalloids (including calcium phosphate).17 The accumulation of oxidized phospholipids additionally results in the formation of M(ox) macrophages (which have weaker phagocytic capabilities) and the transdifferentiation of vascular smooth muscle cells into macrophage-like cells.18,19 Eventually, the loss of macrophage ability to create high-density lipoprotein (HDL) via reverse cholesterol pathways leads to their transformation into foam cells and eventually apoptotic cell death.20–22
In the early atherosclerotic lesion, macrophage efferocytosis facilitates efficient clearance of apoptotic cells and lesion cellularity remains limited.23 As atherosclerosis progresses, efferocytosis becomes impaired due to macrophage cellular reprogramming, a failure of recruitment signal secretion and improper presentation of apoptotic body ligands.24–26 Eventually, the loss of effective efferocytosis leads to cell death by necrosis, the release of immunogenic cytoplasmic material and the formation of a necrotic core within atherosclerotic plaques. In a self-propagating cycle of inflammation, dead and dying macrophages release damage-associated molecular proteins, which promote local and systemic cytokine release, and impaired oxidative phosphorylation inhibits the ability of M1-like macrophages to switch back to the anti-inflammatory M2-like phenotype.27–29 The increased presence of M2-like macrophages with specialized iron handling functions at areas of intraplaque haemorrhage suggests ongoing attempts to arrest plaque progression, promote plaque regression and maintain tissue integrity.30,31 In addition, repeated exposure of macrophages to oxidized phospholipids induces innate immune memory via epigenetic reprogramming and leads to the increased production of pro-inflammatory cytokines, including tumour necrosis factor-alpha (TNFα) and IL-6.32 Finally, secretion of matrix metalloproteinases by M1-like macrophages degrades the extracellular matrix of the thin fibrous cap contributing to plaque instability, potential cap rupture, and ACS.33
Therapies that have significantly improved outcomes over the last several decades have targeted multiple pathways within lipid and energy metabolism and thrombosis.
Pharmacological therapy for cardiovascular inflammation
A cornerstone of treatment for atherosclerosis involves the use of lipid-lowering therapies, including statins and proprotein convertase subtilisin/kexin type 9 (PCSK9) inhibitors. These medications exert their effects in a pleiotropic fashion, largely through action on hepatic cholesterol biosynthesis and receptor recycling with subsequent reductions in LDL cholesterol levels. Inflammation and the role of the errant macrophage remain under-targeted, though recent studies demonstrate well-established lipid-lowering therapies exert unanticipated immunomodulating effects (such as inhibition of the NLRP3 inflammasome or toll-like receptor pathways), and reductions in plaque volume, macrophage burden, and modification of the thin fibrous (by intracoronary imaging).34–36
In 2017, the randomized, placebo-controlled, double-blind Canakinumab Anti-inflammatory Thrombosis Outcome Study (CANTOS) trial provided the first proof-of-principle data that targeted inhibition of inflammation by canakinumab, an IL-1β inhibitor, reduces cardiovascular event rates independent of lipid-lowering treatments.37 However, clinical application remains limited by the cost of the drug and an increased risk of severe respiratory infections although other immunomodulating medications are being evaluated. In the Cardiovascular Inflammation Reduction Trial in 2018, methotrexate failed to demonstrate an improvement in cardiovascular outcomes and the likely reasons have been extensively discussed.38
The subsequent COLCOT study published in 2019 demonstrated a significant reduction in adverse cardiovascular events post-myocardial infarction with the use of low-dose colchicine (0.5 mg once daily).39 Patients with recent myocardial infarction were randomized to either placebo or colchicine in addition to guideline-directed medical therapy. After a median follow-up time of 22.6 months, there was a reduction in the composite primary outcome (death from cardiovascular causes, resuscitated cardiac arrest, myocardial infarction, stroke, or urgent hospitalization for angina leading to revascularization) in the colchicine treated group of 23% compared with placebo, driven largely by reductions in stroke and urgent revascularization. Less than 5% of patients in the trial underwent biomarker assessment, limiting the overall interpretation of observed C-reactive protein (CRP) levels.
A more promising result was reported by the LoDoCo2 randomized clinical trial, which demonstrated a significant reduction in cardiovascular events with the use of colchicine at a low dose (0.5 mg once daily) in patients with stable coronary artery disease.40 Colchicine disrupts tubulin, thereby reducing the migration and replication of inflammatory cells, affecting endothelial function, and inhibiting the NLRP3 inflammasome, but is now also thought to have broader mechanisms of action that are incompletely understood.41 One effect is the indirect reduction in IL-1β activation and downstream reductions in IL-6 and CRP, inflammatory mediators which are known to activate macrophages and propagate atherosclerosis.42 Following these results, colchicine now receives a Class IIb recommendation for the prevention of recurrent cardiovascular events in select high-risk patients.43 The observed trend towards increased non-cardiovascular and all-cause mortality in LoDoCo2 continues to be discussed, although long-term data from familial Mediterranean fever patients and a large metanalysis published in 2021 are very reassuring.44,45
The above-mentioned studies have established the importance of specific inflammatory pathways in atherosclerosis, such as IL-1β and downstream cytokines and the potential for therapeutic inhibition. However, not all patients benefit from immune modulation, which also carries inherent risks. Blood biomarkers have been used to try to identify populations with active inflammation who may be more likely to benefit, but they have been ineffective or lacked specificity thus far. For example, the greatest benefit in CANTOS occurred in those patients who had significant reductions in IL-6 and CRP. Surrogate measures of IL-1β activation such as high-sensitivity CRP, IL-18, and IL-6 have been shown to independently predict cardiovascular events, while others such as serum amyloid A, TNFα, and soluble intercellular adhesion molecule type 1 do not.46,47 Also moderated by IL-1β inhibition, a high neutrophil-lymphocyte ratio independently predicts cardiovascular risk and all-cause mortality though (similarly to soluble biomarkers) has not shown sufficient specificity to be widely applied in clinical practice.48
Improved detection of specific inflammatory pro-atherosclerotic processes or components in vivo could better identify patients with a favourable benefit-to-risk ratio for immune-modulating treatments and offer potential intermediate endpoints for assessing promising therapies in human populations before proceeding to large outcome trials.
Current methods for imaging inflammation and cardiovascular macrophages
In vivo imaging of plaque in human atherosclerosis offers a novel approach to the assessment of inflammation and includes both non-invasive and invasive techniques that could have application in diagnosis, prognosis, and research settings. Indications for clinically available techniques vary widely depending on patient characteristics and clinical context (such as chest pain, ACS, or in guiding a revascularization strategy). In general, invasive coronary techniques are used in the setting of ACS, where the risk stratification with coronary angiography to guide decisions on revascularization improves prognosis. Non-invasive imaging approaches may image multiple vascular territories and may be more useful in imaging chronic cardiovascular syndromes. The following sections outline current methods for imaging inflammation and their potential for detecting macrophages, both directly and indirectly, to better understand their central role in the process of inflammation.
Optical coherence tomography
Coronary optical coherence tomography (OCT) is a catheter-based invasive imaging system used in adjunct to fluoroscopy during percutaneous coronary intervention (PCI).49 During automated pullback along the length of the artery, OCT uses near infrared light, to produce high-resolution, tomographic images and can be used to plan and optimize stent placement.50 Since the first in vivo characterization of coronary atherosclerotic plaque in 2002 using high-resolution OCT, analysis of various cellular structures such as macrophages, vasa-vasorum and cholesterol crystals in near-microscopic detail has been possible.50,51
OCT assessment of macrophages is based on the characteristic visual appearance of punctate regions with high signal attenuation.49 Because of their size and the different refractory index of phagolysosomes and surrounding intra-cellular fluid, investigators have hypothesized that macrophages have a higher OCT signal than the neighbouring tissues.52 However, histologically correlated findings of macrophages on OCT can vary and include appearing as ‘bright spots’ or as ‘shadow/dark regions’.52–55 Some groups have tried to develop algorithms that automatically detect macrophage infiltrations on OCT and that may improve detection compared with the expert reader (see Figure 1).53,54
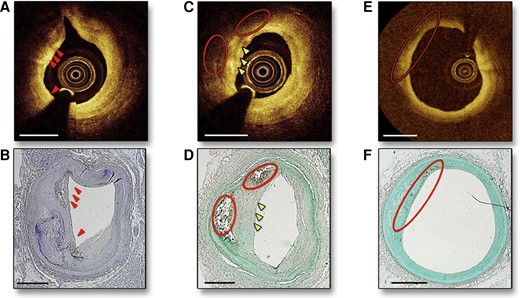
Intravascular coronary OCT demonstrating macrophages visible as bright spots (A, arrows) with CD68 stain confirmation (B). Macrophages are also visualized as bright spots and dark regions (C, arrows and circles, respectively) with histological confirmation of macrophages by CD68 staining (D). A region far from the OCT catheter is depicted in (E) without evidence of bright spots, but with CD68 stain confirmation of macrophages in (F) likely reflecting loss of information at greater distances from the catheter. Adapted with permission from Phipps et al.53
The use of these specialized algorithms requires dedicated software such that automated real time quantification of plaque macrophages in the catheter laboratory is not yet possible. A summary of various studies using specialized off-line algorithms to study macrophages along with histological validation is presented in Table 1.
Summary of studies investigating the qualitative assessment of macrophages using OCT
Author . | Study aim . | Sample . | Methods . | Results . |
---|---|---|---|---|
Tearney et al (2003)52 | To investigate the use of OCT for identifying macrophages in fibrous caps | 26 lipid-rich atherosclerotic arterial segments obtained at autopsy | Macrophage density was quantified morphometrically by immunoperoxidase staining with CD68 and then compared with the NSD of the OCT signal intensity |
|
Phipps et al (2015)53 | To quantitatively identify macrophages using a novel algorithm including tissue depth, distance from light source, and signal-to-noise ratio | Fresh 14 coronary arteries from 10 human hearts | Use of specific OCT algorithm (an intensity threshold was employed on the normalized algorithm OCT data, considering the distance between the catheter and the surface of the vessel) in 1599 OCT cross-sectional images and validated with histology |
|
Di Vito et al (2015)54 | To investigate the capability of OCT to identify coronary plaque macrophage presence using tissue property indexes | 15 epicardial coronary arteries | OCT analysis of histologically correlated regions of interest (ROI) was performed in a stepwise manner The ROI after histological evaluation were divided in to inflamed (ROI having a macrophage percentage >10) or non-inflamed (ROI having a macrophage percentage <10) The application of OCT-derived tissue property indexes including signal attenuation, NSD and granulometry index were again applied in a stepwise manner |
|
Gutierrez-Chico et al (2017)56 | To investigate whether imaging of macrophages in OCT could be enhanced by means of superparamagnetic nanoparticles | Cell pellets | Comparison of optical backscattering and attenuation of cell pellets containing RAW 264.7 macrophages with those of macrophagic cell pellets labelled with very small superparamagnetic oxidized nanoparticles (VSOP) by means of light intensity analysis in OCT The backscattering was estimated by the peak normalization intensity, whilst the attenuation was estimated by the number of pixels between the peak and normalized intensity (PNI) |
|
To evaluate the accuracy of OCT in tissue characterization in vivo | 25 patients with stable angina undergoing directional coronary atherectomy | OCT was performed before and after a single debulking. The debulked region was determined on OCT and classified into fibrous tissue, lipid, calcification, thrombus and macrophage accumulation, which were compared with histology |
| |
Shimokado et al (2018)57 | To assess agreement between OCT and healed coronary plaques (HCP) ex vivo, and to evaluate the prevalence and characteristics of HCP’s in vivo | In a subset clinical study 60 lesions (in 60 patients) were compared based on presence or absence of HCP | 73 coronary arteries were examined by OCT from cadavers. The left main coronary and the proximal and middle portion of the 3 coronary arteries were examined One or more heterogonous signal-rich layers of different optical density located close to luminal surface with clear demarcation from the underlying tissue, which was deemed OCT-derived HCP |
|
Rico-Jimenez et al (2019)58 | To introduce and validate a simple intravascular OCT image processing method for automated, accurate and fast detection of macrophage infiltration with atherosclerotic plaque | OCT images acquired from 28 cadaveric human coronary artery segments | The ratio of the NSD was estimated over two axially adjacent regions of interest in OCT cross-sectional images (B-Scan) When applied to entire OCT B-scans, the areas of plaque with high NSD ratio were highlighted, which was demonstrated to be correlated with the degree of coronary plaque macrophage infiltration |
|
Nicol et al (2021)59 | To investigate whether tissue attenuation differs between regions with and without neointimal foam cell infiltration, and whether tissue attenuation index could reliably identify patients with neointimal foamy macrophage infiltration as an early sign of atherosclerosis | 13 autopsy samples of stented coronary arteries. 29 patients with in-stent restenosis undergoing OCT were included | 13 autopsy samples of stented coronary arteries were assessed to determine attenuation index of neointima with and without foam cells. Based on this, a threshold for homogenous and non-homogenous neointima was determined The established attenuation index derived from autopsy cases was applied to detect neointimal foam cells in clinical cases presenting with in-stent restenosis |
|
Author . | Study aim . | Sample . | Methods . | Results . |
---|---|---|---|---|
Tearney et al (2003)52 | To investigate the use of OCT for identifying macrophages in fibrous caps | 26 lipid-rich atherosclerotic arterial segments obtained at autopsy | Macrophage density was quantified morphometrically by immunoperoxidase staining with CD68 and then compared with the NSD of the OCT signal intensity |
|
Phipps et al (2015)53 | To quantitatively identify macrophages using a novel algorithm including tissue depth, distance from light source, and signal-to-noise ratio | Fresh 14 coronary arteries from 10 human hearts | Use of specific OCT algorithm (an intensity threshold was employed on the normalized algorithm OCT data, considering the distance between the catheter and the surface of the vessel) in 1599 OCT cross-sectional images and validated with histology |
|
Di Vito et al (2015)54 | To investigate the capability of OCT to identify coronary plaque macrophage presence using tissue property indexes | 15 epicardial coronary arteries | OCT analysis of histologically correlated regions of interest (ROI) was performed in a stepwise manner The ROI after histological evaluation were divided in to inflamed (ROI having a macrophage percentage >10) or non-inflamed (ROI having a macrophage percentage <10) The application of OCT-derived tissue property indexes including signal attenuation, NSD and granulometry index were again applied in a stepwise manner |
|
Gutierrez-Chico et al (2017)56 | To investigate whether imaging of macrophages in OCT could be enhanced by means of superparamagnetic nanoparticles | Cell pellets | Comparison of optical backscattering and attenuation of cell pellets containing RAW 264.7 macrophages with those of macrophagic cell pellets labelled with very small superparamagnetic oxidized nanoparticles (VSOP) by means of light intensity analysis in OCT The backscattering was estimated by the peak normalization intensity, whilst the attenuation was estimated by the number of pixels between the peak and normalized intensity (PNI) |
|
To evaluate the accuracy of OCT in tissue characterization in vivo | 25 patients with stable angina undergoing directional coronary atherectomy | OCT was performed before and after a single debulking. The debulked region was determined on OCT and classified into fibrous tissue, lipid, calcification, thrombus and macrophage accumulation, which were compared with histology |
| |
Shimokado et al (2018)57 | To assess agreement between OCT and healed coronary plaques (HCP) ex vivo, and to evaluate the prevalence and characteristics of HCP’s in vivo | In a subset clinical study 60 lesions (in 60 patients) were compared based on presence or absence of HCP | 73 coronary arteries were examined by OCT from cadavers. The left main coronary and the proximal and middle portion of the 3 coronary arteries were examined One or more heterogonous signal-rich layers of different optical density located close to luminal surface with clear demarcation from the underlying tissue, which was deemed OCT-derived HCP |
|
Rico-Jimenez et al (2019)58 | To introduce and validate a simple intravascular OCT image processing method for automated, accurate and fast detection of macrophage infiltration with atherosclerotic plaque | OCT images acquired from 28 cadaveric human coronary artery segments | The ratio of the NSD was estimated over two axially adjacent regions of interest in OCT cross-sectional images (B-Scan) When applied to entire OCT B-scans, the areas of plaque with high NSD ratio were highlighted, which was demonstrated to be correlated with the degree of coronary plaque macrophage infiltration |
|
Nicol et al (2021)59 | To investigate whether tissue attenuation differs between regions with and without neointimal foam cell infiltration, and whether tissue attenuation index could reliably identify patients with neointimal foamy macrophage infiltration as an early sign of atherosclerosis | 13 autopsy samples of stented coronary arteries. 29 patients with in-stent restenosis undergoing OCT were included | 13 autopsy samples of stented coronary arteries were assessed to determine attenuation index of neointima with and without foam cells. Based on this, a threshold for homogenous and non-homogenous neointima was determined The established attenuation index derived from autopsy cases was applied to detect neointimal foam cells in clinical cases presenting with in-stent restenosis |
|
AUC, area under the ROC curve; CD, cluster of differentiation; HCP, healed coronary plaque; NSD, normalized-intensity standard deviation; OCT, optical coherence tomography; PNI, peak and normalized intensity; ROC, receiver operating characteristic; ROI, region of interest; SD, standard deviation; VSOP, very small superparamagnetic oxidized nanoparticles.
Summary of studies investigating the qualitative assessment of macrophages using OCT
Author . | Study aim . | Sample . | Methods . | Results . |
---|---|---|---|---|
Tearney et al (2003)52 | To investigate the use of OCT for identifying macrophages in fibrous caps | 26 lipid-rich atherosclerotic arterial segments obtained at autopsy | Macrophage density was quantified morphometrically by immunoperoxidase staining with CD68 and then compared with the NSD of the OCT signal intensity |
|
Phipps et al (2015)53 | To quantitatively identify macrophages using a novel algorithm including tissue depth, distance from light source, and signal-to-noise ratio | Fresh 14 coronary arteries from 10 human hearts | Use of specific OCT algorithm (an intensity threshold was employed on the normalized algorithm OCT data, considering the distance between the catheter and the surface of the vessel) in 1599 OCT cross-sectional images and validated with histology |
|
Di Vito et al (2015)54 | To investigate the capability of OCT to identify coronary plaque macrophage presence using tissue property indexes | 15 epicardial coronary arteries | OCT analysis of histologically correlated regions of interest (ROI) was performed in a stepwise manner The ROI after histological evaluation were divided in to inflamed (ROI having a macrophage percentage >10) or non-inflamed (ROI having a macrophage percentage <10) The application of OCT-derived tissue property indexes including signal attenuation, NSD and granulometry index were again applied in a stepwise manner |
|
Gutierrez-Chico et al (2017)56 | To investigate whether imaging of macrophages in OCT could be enhanced by means of superparamagnetic nanoparticles | Cell pellets | Comparison of optical backscattering and attenuation of cell pellets containing RAW 264.7 macrophages with those of macrophagic cell pellets labelled with very small superparamagnetic oxidized nanoparticles (VSOP) by means of light intensity analysis in OCT The backscattering was estimated by the peak normalization intensity, whilst the attenuation was estimated by the number of pixels between the peak and normalized intensity (PNI) |
|
To evaluate the accuracy of OCT in tissue characterization in vivo | 25 patients with stable angina undergoing directional coronary atherectomy | OCT was performed before and after a single debulking. The debulked region was determined on OCT and classified into fibrous tissue, lipid, calcification, thrombus and macrophage accumulation, which were compared with histology |
| |
Shimokado et al (2018)57 | To assess agreement between OCT and healed coronary plaques (HCP) ex vivo, and to evaluate the prevalence and characteristics of HCP’s in vivo | In a subset clinical study 60 lesions (in 60 patients) were compared based on presence or absence of HCP | 73 coronary arteries were examined by OCT from cadavers. The left main coronary and the proximal and middle portion of the 3 coronary arteries were examined One or more heterogonous signal-rich layers of different optical density located close to luminal surface with clear demarcation from the underlying tissue, which was deemed OCT-derived HCP |
|
Rico-Jimenez et al (2019)58 | To introduce and validate a simple intravascular OCT image processing method for automated, accurate and fast detection of macrophage infiltration with atherosclerotic plaque | OCT images acquired from 28 cadaveric human coronary artery segments | The ratio of the NSD was estimated over two axially adjacent regions of interest in OCT cross-sectional images (B-Scan) When applied to entire OCT B-scans, the areas of plaque with high NSD ratio were highlighted, which was demonstrated to be correlated with the degree of coronary plaque macrophage infiltration |
|
Nicol et al (2021)59 | To investigate whether tissue attenuation differs between regions with and without neointimal foam cell infiltration, and whether tissue attenuation index could reliably identify patients with neointimal foamy macrophage infiltration as an early sign of atherosclerosis | 13 autopsy samples of stented coronary arteries. 29 patients with in-stent restenosis undergoing OCT were included | 13 autopsy samples of stented coronary arteries were assessed to determine attenuation index of neointima with and without foam cells. Based on this, a threshold for homogenous and non-homogenous neointima was determined The established attenuation index derived from autopsy cases was applied to detect neointimal foam cells in clinical cases presenting with in-stent restenosis |
|
Author . | Study aim . | Sample . | Methods . | Results . |
---|---|---|---|---|
Tearney et al (2003)52 | To investigate the use of OCT for identifying macrophages in fibrous caps | 26 lipid-rich atherosclerotic arterial segments obtained at autopsy | Macrophage density was quantified morphometrically by immunoperoxidase staining with CD68 and then compared with the NSD of the OCT signal intensity |
|
Phipps et al (2015)53 | To quantitatively identify macrophages using a novel algorithm including tissue depth, distance from light source, and signal-to-noise ratio | Fresh 14 coronary arteries from 10 human hearts | Use of specific OCT algorithm (an intensity threshold was employed on the normalized algorithm OCT data, considering the distance between the catheter and the surface of the vessel) in 1599 OCT cross-sectional images and validated with histology |
|
Di Vito et al (2015)54 | To investigate the capability of OCT to identify coronary plaque macrophage presence using tissue property indexes | 15 epicardial coronary arteries | OCT analysis of histologically correlated regions of interest (ROI) was performed in a stepwise manner The ROI after histological evaluation were divided in to inflamed (ROI having a macrophage percentage >10) or non-inflamed (ROI having a macrophage percentage <10) The application of OCT-derived tissue property indexes including signal attenuation, NSD and granulometry index were again applied in a stepwise manner |
|
Gutierrez-Chico et al (2017)56 | To investigate whether imaging of macrophages in OCT could be enhanced by means of superparamagnetic nanoparticles | Cell pellets | Comparison of optical backscattering and attenuation of cell pellets containing RAW 264.7 macrophages with those of macrophagic cell pellets labelled with very small superparamagnetic oxidized nanoparticles (VSOP) by means of light intensity analysis in OCT The backscattering was estimated by the peak normalization intensity, whilst the attenuation was estimated by the number of pixels between the peak and normalized intensity (PNI) |
|
To evaluate the accuracy of OCT in tissue characterization in vivo | 25 patients with stable angina undergoing directional coronary atherectomy | OCT was performed before and after a single debulking. The debulked region was determined on OCT and classified into fibrous tissue, lipid, calcification, thrombus and macrophage accumulation, which were compared with histology |
| |
Shimokado et al (2018)57 | To assess agreement between OCT and healed coronary plaques (HCP) ex vivo, and to evaluate the prevalence and characteristics of HCP’s in vivo | In a subset clinical study 60 lesions (in 60 patients) were compared based on presence or absence of HCP | 73 coronary arteries were examined by OCT from cadavers. The left main coronary and the proximal and middle portion of the 3 coronary arteries were examined One or more heterogonous signal-rich layers of different optical density located close to luminal surface with clear demarcation from the underlying tissue, which was deemed OCT-derived HCP |
|
Rico-Jimenez et al (2019)58 | To introduce and validate a simple intravascular OCT image processing method for automated, accurate and fast detection of macrophage infiltration with atherosclerotic plaque | OCT images acquired from 28 cadaveric human coronary artery segments | The ratio of the NSD was estimated over two axially adjacent regions of interest in OCT cross-sectional images (B-Scan) When applied to entire OCT B-scans, the areas of plaque with high NSD ratio were highlighted, which was demonstrated to be correlated with the degree of coronary plaque macrophage infiltration |
|
Nicol et al (2021)59 | To investigate whether tissue attenuation differs between regions with and without neointimal foam cell infiltration, and whether tissue attenuation index could reliably identify patients with neointimal foamy macrophage infiltration as an early sign of atherosclerosis | 13 autopsy samples of stented coronary arteries. 29 patients with in-stent restenosis undergoing OCT were included | 13 autopsy samples of stented coronary arteries were assessed to determine attenuation index of neointima with and without foam cells. Based on this, a threshold for homogenous and non-homogenous neointima was determined The established attenuation index derived from autopsy cases was applied to detect neointimal foam cells in clinical cases presenting with in-stent restenosis |
|
AUC, area under the ROC curve; CD, cluster of differentiation; HCP, healed coronary plaque; NSD, normalized-intensity standard deviation; OCT, optical coherence tomography; PNI, peak and normalized intensity; ROC, receiver operating characteristic; ROI, region of interest; SD, standard deviation; VSOP, very small superparamagnetic oxidized nanoparticles.
Visual detection and quantification of macrophages on OCT is reproducible and requires in-depth knowledge of the varied OCT appearance of numerous plaque features.60–62 Macrophage accumulation may also be confused on occasion with microcalcification, cholesterol crystals and the internal or external elastic membrane.50 Macrophages attenuate the OCT light significantly, and as a result, superficial macrophages can shadow underlying tissue, giving it the appearance of a necrotic core.63 Despite these limitations, the detection of macrophages on OCT is associated with clinical cardiovascular risk.64–66
Prati et al.64 performed a prospective, observational study which included 1003 patients who were referred for coronary angiography and underwent OCT imaging in an untreated proximal left anterior descending coronary artery. This study elegantly explored whether multiple high-risk OCT plaque features predict cardiovascular events at the patient-level. The hard composite endpoint of cardiac death and target vessel myocardial infarction was 7.5 × higher in patients who had lesions with a thin cap fibroatheroma (TCFA), lipid arc >180°, minimum lumen area <3.5 mm2 and macrophage accumulation, when compared with patients without high-risk plaque characteristics (18.9 vs. 3.0%). Notably, the prevalence of all four high-risk features was 3.6%, and 19% of these high-risk plaques caused a subsequent cardiovascular event within 1 year.
In a Retrospective2 centre study of patients, who were found to have plaque erosion and were studied with OCT, the presence of macrophages was a marker of cardiovascular events over the subsequent 2.5 years.65 However, the prevalence of macrophages is considerably lower in patients with erosion when compared with those with rupture as underlying mechanism for ACS, which would also attenuate the predictive value of macrophage-rich plaque morphology in the overall population.66 The presence of plaque macrophages on OCT is strongly correlated with other high-risk features including lipids, calcification, thin fibrous caps, and vasa-vasorum so that it is not known whether or not macrophages is an independent marker of risk. OCT is currently by far the most studied and validated method to depict macrophages and it is available for clinical use. Studies investigating the quantitative assessment of macrophages on OCT and associations with clinical coronary disease including predictive value are further summarized in Table 2. The subsequent section discusses other experimental techniques.
Summary of studies investigating the clinical significance of macrophages in various coronary syndromes
Author . | Aim . | Sample . | Methods . | Results . |
---|---|---|---|---|
MacMeill (2004)67 | Using OCT to investigate the relationship between macrophages distributions and clinical coronary syndromes | 49 subjects undergoing single vessel stenting for de novo coronary artery disease | OCT images were acquired at 4 frames/s during intermittent saline injections After automated segmentation of fibroatheroma cap comparison between the surface (<50 μm from the lumen) and subsurface (>50 μm) macrophage densities of the was performed. Macrophage density was studied using the NSD of the OCT signal |
|
Raffel (2007)68 | To evaluate the relationship between peripheral WBC count, plaque fibrous cap macrophage density and the presence of TCFA on OCT | 43 patients undergoing coronary angiography | Macrophage density was assessed as the average of the NSD values within the segmented/ROI of the cap (mean NSD) For individual plaques, the value of the highest macrophage density obtained from the 3 sampling sites was used for subsequent data analysis WBC counts were obtained in all cases |
|
Minami (2015)69 | To investigate the clinical significance of bright spots in coronary plaque detected by OCT in patients with coronary artery disease | 112 patients (50 ACS and 62 SAP) | Macrophage density was calculated as the number of bright spots divided by the total number of analysed pixels with the 5mm span of vessel centred around the culprit frame (a total of 25 cross-sectional frames, including the culprit frame and 12 frames on both proximal and distal sides). Macrophage location was considered superficial (≤250 μm) or deep (≤1000 μm) |
|
Galon (2015)70 | To compare OCT volumetric quantification of fibrous cap and macrophage detection using visual assessment and automated image processing algorithms in non-culprit lesions | 67 consecutive patients with STEMI and SAP | FC was manually delineated by a computer-aided method and automatically classified into three thickness categories: fibrous cap <65 μm, TCFA 65–150 μm, and >150 μm. Automated detection and quantification of macrophage was also performed |
|
Park (2017)71 | To investigate whether OCT markers of plaque vulnerability predict cardiac allograft vasculopathy after heart transplantation | 34 consecutive patients | Presence of vulnerability markers (lipid pools, TCFA, macrophages and microchannels) was assessed in 100 consecutive frames in 20 mm proximal segments of the LAD The total number of appearances of vulnerable markers was defined as a vulnerability score (VS). Plaque volume (PV) was measured in the same coronary segment using IVUS at baseline and 1-year follow-up and the association between the baseline VS, and the change in percent PV (PV/vessel volume × 100 [%PV]) was evaluated |
|
Taglieri (2017)72 | To evaluate the relationship between aortic inflammation (as assessed by 18F-FDG PET) and features of plaque vulnerability (as assessed by OCT) | 30 consecutive patients with ACS undergoing PCI | All patients underwent 3 vessel OCT before intervention and 18F-FGD-PET before discharge 18F-FDG uptake in both ascending and descending aorta was measured as averaged mean and maximum TBR or as active slices (TBRmax ≥1.6) |
|
Lannaccone (2018)73 | To evaluate the clinical impact of OCT-detected culprit plaque features in ACS | 209 patients with ACS | Retrospective analysis of a multi-centre registry All ACS patients undergoing OCT of culprit and non-culprit plaque with follow-up conducted through clinical visits or phone calls at 6 months |
|
Joner (2018)74 | To assess neo-atherosclerosis in patients presenting with stent thrombosis using OCT | 134 patients presenting with very late stent thrombosis | Plaque morphology was analysed in every other frame. The presence of fibroatheroma and signal attenuation indicative of macrophages (at least 1 quadrant) was recorded |
|
Okamoto (2019)75 | To study the relationship between healed plaques and the development of significant coronary stenosis in SAP | 205 SAP patients in retrospective arm 42 samples from 18 SAP in prospective arm | In the retrospective arm, the prevalence and clinical characteristics of OCT-based layered plaque at the culprit site in patients with SAP was assessed In the prospective arm, the histopathological characteristics of layered plaques in SAP lesions were identified using DCA samples |
|
Wu et al (2019)76 | To investigate the correlation between insulin resistance and culprit plaque characteristics using OCT in patients with ACS | 145 patients with ACS | 159 culprit plaques in 145 patients were identified. Patient were identified into 4 groups according to the homeostasis model assessment of insulin resistance (HOMA-IR) |
|
Raber et al (2019)77 | To study changes in OCT-defined morphological changes in patients with STEMI receiving high-intensity statin therapy | 103 patients with STEMI | 103 patients with STEMI undergoing IVUS and OCT of two non-infarct related arteries and a repeat coronary angiography at 13 months were recruited Co-primary endpoints for OCT analysis were change in minimum FCT and macrophage accumulation angle Patients received 20 mg of rosuvastatin daily for the first 2 weeks and were then up-titrated to 40 mg daily |
|
Prati et al (2020)78 | To investigate the predictive value of high-risk OCT plaque features including macrophages | 1003 patient with coronary artery disease undergoing PCI | The predictive value of high-risk OCT plaque features including MLA, FCT, lipid arc circumferential extension and the presence of OCT-detected macrophages was studied in a proximal LAD segment The primary endpoint of target-segment MI and/or cardiac death was studied at 1-year follow-up |
|
Gatto et al (2020)60 | To revise the clinical and demographic variables of patients who have coronary plaques with macrophages, and investigate the reproducibility of quantitative assessment | Post-hoc analysis of the CLIMA study | A total of 577 patients out of 1003 showed macrophage accumulation. Three groups were identified: Group 1 (426)—without macrophages Group 2 (296)—patients with low macrophage content (less than median value of 67° of circumferential arc) Group 3 (281)—patients with high macrophage content (more than median value of 67° of circumferential arc) |
|
Taglieri et al (2020)79 | To investigate the prevalence and the features of OCT-detected macrophage accumulation in culprit plaques as compared with non-culprit plaques | 32 patients with an index NSTEMI who successfully underwent three vessel OCT | 32 consecutive patients in a single centre who were undergoing PCI of at least one coronary lesion were prospectively enrolled Patients underwent three vessel OCT at the time of PCI with a 20 mm/s pullback speed that was applied during automated injection of intracoronary iso-osmolar contrast media |
|
Majeed et al (2020)80 | To study the association between NaF PET uptake and high-risk plaque features on OCT and CTCA, and the potential application to patient-level risk stratification | 62 patients with ACS were recruited prospectively | Patients underwent multivessel OCT at the time of angiography, with subsequent CTCA and NaF PET within a maximum time window of up to 4 weeks A low-risk cohort (n = 10) with no prior coronary artery disease and calcium score of 0 were used to determine the upper limit of the normal reference range of radiotracer activity for each coronary segment based on 18- segment model. After co-registration, a total of 286 coronary segments were analysed for association between NaF activity and high-risk OCT and CTCA plaque features Patient-level data were analysed based on number of coronary segments with NaF positivity |
|
Author . | Aim . | Sample . | Methods . | Results . |
---|---|---|---|---|
MacMeill (2004)67 | Using OCT to investigate the relationship between macrophages distributions and clinical coronary syndromes | 49 subjects undergoing single vessel stenting for de novo coronary artery disease | OCT images were acquired at 4 frames/s during intermittent saline injections After automated segmentation of fibroatheroma cap comparison between the surface (<50 μm from the lumen) and subsurface (>50 μm) macrophage densities of the was performed. Macrophage density was studied using the NSD of the OCT signal |
|
Raffel (2007)68 | To evaluate the relationship between peripheral WBC count, plaque fibrous cap macrophage density and the presence of TCFA on OCT | 43 patients undergoing coronary angiography | Macrophage density was assessed as the average of the NSD values within the segmented/ROI of the cap (mean NSD) For individual plaques, the value of the highest macrophage density obtained from the 3 sampling sites was used for subsequent data analysis WBC counts were obtained in all cases |
|
Minami (2015)69 | To investigate the clinical significance of bright spots in coronary plaque detected by OCT in patients with coronary artery disease | 112 patients (50 ACS and 62 SAP) | Macrophage density was calculated as the number of bright spots divided by the total number of analysed pixels with the 5mm span of vessel centred around the culprit frame (a total of 25 cross-sectional frames, including the culprit frame and 12 frames on both proximal and distal sides). Macrophage location was considered superficial (≤250 μm) or deep (≤1000 μm) |
|
Galon (2015)70 | To compare OCT volumetric quantification of fibrous cap and macrophage detection using visual assessment and automated image processing algorithms in non-culprit lesions | 67 consecutive patients with STEMI and SAP | FC was manually delineated by a computer-aided method and automatically classified into three thickness categories: fibrous cap <65 μm, TCFA 65–150 μm, and >150 μm. Automated detection and quantification of macrophage was also performed |
|
Park (2017)71 | To investigate whether OCT markers of plaque vulnerability predict cardiac allograft vasculopathy after heart transplantation | 34 consecutive patients | Presence of vulnerability markers (lipid pools, TCFA, macrophages and microchannels) was assessed in 100 consecutive frames in 20 mm proximal segments of the LAD The total number of appearances of vulnerable markers was defined as a vulnerability score (VS). Plaque volume (PV) was measured in the same coronary segment using IVUS at baseline and 1-year follow-up and the association between the baseline VS, and the change in percent PV (PV/vessel volume × 100 [%PV]) was evaluated |
|
Taglieri (2017)72 | To evaluate the relationship between aortic inflammation (as assessed by 18F-FDG PET) and features of plaque vulnerability (as assessed by OCT) | 30 consecutive patients with ACS undergoing PCI | All patients underwent 3 vessel OCT before intervention and 18F-FGD-PET before discharge 18F-FDG uptake in both ascending and descending aorta was measured as averaged mean and maximum TBR or as active slices (TBRmax ≥1.6) |
|
Lannaccone (2018)73 | To evaluate the clinical impact of OCT-detected culprit plaque features in ACS | 209 patients with ACS | Retrospective analysis of a multi-centre registry All ACS patients undergoing OCT of culprit and non-culprit plaque with follow-up conducted through clinical visits or phone calls at 6 months |
|
Joner (2018)74 | To assess neo-atherosclerosis in patients presenting with stent thrombosis using OCT | 134 patients presenting with very late stent thrombosis | Plaque morphology was analysed in every other frame. The presence of fibroatheroma and signal attenuation indicative of macrophages (at least 1 quadrant) was recorded |
|
Okamoto (2019)75 | To study the relationship between healed plaques and the development of significant coronary stenosis in SAP | 205 SAP patients in retrospective arm 42 samples from 18 SAP in prospective arm | In the retrospective arm, the prevalence and clinical characteristics of OCT-based layered plaque at the culprit site in patients with SAP was assessed In the prospective arm, the histopathological characteristics of layered plaques in SAP lesions were identified using DCA samples |
|
Wu et al (2019)76 | To investigate the correlation between insulin resistance and culprit plaque characteristics using OCT in patients with ACS | 145 patients with ACS | 159 culprit plaques in 145 patients were identified. Patient were identified into 4 groups according to the homeostasis model assessment of insulin resistance (HOMA-IR) |
|
Raber et al (2019)77 | To study changes in OCT-defined morphological changes in patients with STEMI receiving high-intensity statin therapy | 103 patients with STEMI | 103 patients with STEMI undergoing IVUS and OCT of two non-infarct related arteries and a repeat coronary angiography at 13 months were recruited Co-primary endpoints for OCT analysis were change in minimum FCT and macrophage accumulation angle Patients received 20 mg of rosuvastatin daily for the first 2 weeks and were then up-titrated to 40 mg daily |
|
Prati et al (2020)78 | To investigate the predictive value of high-risk OCT plaque features including macrophages | 1003 patient with coronary artery disease undergoing PCI | The predictive value of high-risk OCT plaque features including MLA, FCT, lipid arc circumferential extension and the presence of OCT-detected macrophages was studied in a proximal LAD segment The primary endpoint of target-segment MI and/or cardiac death was studied at 1-year follow-up |
|
Gatto et al (2020)60 | To revise the clinical and demographic variables of patients who have coronary plaques with macrophages, and investigate the reproducibility of quantitative assessment | Post-hoc analysis of the CLIMA study | A total of 577 patients out of 1003 showed macrophage accumulation. Three groups were identified: Group 1 (426)—without macrophages Group 2 (296)—patients with low macrophage content (less than median value of 67° of circumferential arc) Group 3 (281)—patients with high macrophage content (more than median value of 67° of circumferential arc) |
|
Taglieri et al (2020)79 | To investigate the prevalence and the features of OCT-detected macrophage accumulation in culprit plaques as compared with non-culprit plaques | 32 patients with an index NSTEMI who successfully underwent three vessel OCT | 32 consecutive patients in a single centre who were undergoing PCI of at least one coronary lesion were prospectively enrolled Patients underwent three vessel OCT at the time of PCI with a 20 mm/s pullback speed that was applied during automated injection of intracoronary iso-osmolar contrast media |
|
Majeed et al (2020)80 | To study the association between NaF PET uptake and high-risk plaque features on OCT and CTCA, and the potential application to patient-level risk stratification | 62 patients with ACS were recruited prospectively | Patients underwent multivessel OCT at the time of angiography, with subsequent CTCA and NaF PET within a maximum time window of up to 4 weeks A low-risk cohort (n = 10) with no prior coronary artery disease and calcium score of 0 were used to determine the upper limit of the normal reference range of radiotracer activity for each coronary segment based on 18- segment model. After co-registration, a total of 286 coronary segments were analysed for association between NaF activity and high-risk OCT and CTCA plaque features Patient-level data were analysed based on number of coronary segments with NaF positivity |
|
ACS, acute coronary syndrome; CI, confidence interval; CTCA, CT coronary angiography; FCT, fibrous cap thickness; HR, hazard ratio; IVUS, intravascular ultrasound; LAD, left anterior descending artery; MI, myocardial infarction; MLA, minimal luminal area; NaF, 18F-sodium fluoride; NSD, normalized-intensity standard deviation; OCT, optical coherence tomography; PCI, percutaneous coronary intervention; PET, positron emission tomography; ROI, region of interest; SAP, stable angina pectoris; STEMI, ST-segment elevation myocardial infarction; TBR, target-to-background ratio; TCFA, thin cap fibroatheroma; WBC, white blood cell.
Summary of studies investigating the clinical significance of macrophages in various coronary syndromes
Author . | Aim . | Sample . | Methods . | Results . |
---|---|---|---|---|
MacMeill (2004)67 | Using OCT to investigate the relationship between macrophages distributions and clinical coronary syndromes | 49 subjects undergoing single vessel stenting for de novo coronary artery disease | OCT images were acquired at 4 frames/s during intermittent saline injections After automated segmentation of fibroatheroma cap comparison between the surface (<50 μm from the lumen) and subsurface (>50 μm) macrophage densities of the was performed. Macrophage density was studied using the NSD of the OCT signal |
|
Raffel (2007)68 | To evaluate the relationship between peripheral WBC count, plaque fibrous cap macrophage density and the presence of TCFA on OCT | 43 patients undergoing coronary angiography | Macrophage density was assessed as the average of the NSD values within the segmented/ROI of the cap (mean NSD) For individual plaques, the value of the highest macrophage density obtained from the 3 sampling sites was used for subsequent data analysis WBC counts were obtained in all cases |
|
Minami (2015)69 | To investigate the clinical significance of bright spots in coronary plaque detected by OCT in patients with coronary artery disease | 112 patients (50 ACS and 62 SAP) | Macrophage density was calculated as the number of bright spots divided by the total number of analysed pixels with the 5mm span of vessel centred around the culprit frame (a total of 25 cross-sectional frames, including the culprit frame and 12 frames on both proximal and distal sides). Macrophage location was considered superficial (≤250 μm) or deep (≤1000 μm) |
|
Galon (2015)70 | To compare OCT volumetric quantification of fibrous cap and macrophage detection using visual assessment and automated image processing algorithms in non-culprit lesions | 67 consecutive patients with STEMI and SAP | FC was manually delineated by a computer-aided method and automatically classified into three thickness categories: fibrous cap <65 μm, TCFA 65–150 μm, and >150 μm. Automated detection and quantification of macrophage was also performed |
|
Park (2017)71 | To investigate whether OCT markers of plaque vulnerability predict cardiac allograft vasculopathy after heart transplantation | 34 consecutive patients | Presence of vulnerability markers (lipid pools, TCFA, macrophages and microchannels) was assessed in 100 consecutive frames in 20 mm proximal segments of the LAD The total number of appearances of vulnerable markers was defined as a vulnerability score (VS). Plaque volume (PV) was measured in the same coronary segment using IVUS at baseline and 1-year follow-up and the association between the baseline VS, and the change in percent PV (PV/vessel volume × 100 [%PV]) was evaluated |
|
Taglieri (2017)72 | To evaluate the relationship between aortic inflammation (as assessed by 18F-FDG PET) and features of plaque vulnerability (as assessed by OCT) | 30 consecutive patients with ACS undergoing PCI | All patients underwent 3 vessel OCT before intervention and 18F-FGD-PET before discharge 18F-FDG uptake in both ascending and descending aorta was measured as averaged mean and maximum TBR or as active slices (TBRmax ≥1.6) |
|
Lannaccone (2018)73 | To evaluate the clinical impact of OCT-detected culprit plaque features in ACS | 209 patients with ACS | Retrospective analysis of a multi-centre registry All ACS patients undergoing OCT of culprit and non-culprit plaque with follow-up conducted through clinical visits or phone calls at 6 months |
|
Joner (2018)74 | To assess neo-atherosclerosis in patients presenting with stent thrombosis using OCT | 134 patients presenting with very late stent thrombosis | Plaque morphology was analysed in every other frame. The presence of fibroatheroma and signal attenuation indicative of macrophages (at least 1 quadrant) was recorded |
|
Okamoto (2019)75 | To study the relationship between healed plaques and the development of significant coronary stenosis in SAP | 205 SAP patients in retrospective arm 42 samples from 18 SAP in prospective arm | In the retrospective arm, the prevalence and clinical characteristics of OCT-based layered plaque at the culprit site in patients with SAP was assessed In the prospective arm, the histopathological characteristics of layered plaques in SAP lesions were identified using DCA samples |
|
Wu et al (2019)76 | To investigate the correlation between insulin resistance and culprit plaque characteristics using OCT in patients with ACS | 145 patients with ACS | 159 culprit plaques in 145 patients were identified. Patient were identified into 4 groups according to the homeostasis model assessment of insulin resistance (HOMA-IR) |
|
Raber et al (2019)77 | To study changes in OCT-defined morphological changes in patients with STEMI receiving high-intensity statin therapy | 103 patients with STEMI | 103 patients with STEMI undergoing IVUS and OCT of two non-infarct related arteries and a repeat coronary angiography at 13 months were recruited Co-primary endpoints for OCT analysis were change in minimum FCT and macrophage accumulation angle Patients received 20 mg of rosuvastatin daily for the first 2 weeks and were then up-titrated to 40 mg daily |
|
Prati et al (2020)78 | To investigate the predictive value of high-risk OCT plaque features including macrophages | 1003 patient with coronary artery disease undergoing PCI | The predictive value of high-risk OCT plaque features including MLA, FCT, lipid arc circumferential extension and the presence of OCT-detected macrophages was studied in a proximal LAD segment The primary endpoint of target-segment MI and/or cardiac death was studied at 1-year follow-up |
|
Gatto et al (2020)60 | To revise the clinical and demographic variables of patients who have coronary plaques with macrophages, and investigate the reproducibility of quantitative assessment | Post-hoc analysis of the CLIMA study | A total of 577 patients out of 1003 showed macrophage accumulation. Three groups were identified: Group 1 (426)—without macrophages Group 2 (296)—patients with low macrophage content (less than median value of 67° of circumferential arc) Group 3 (281)—patients with high macrophage content (more than median value of 67° of circumferential arc) |
|
Taglieri et al (2020)79 | To investigate the prevalence and the features of OCT-detected macrophage accumulation in culprit plaques as compared with non-culprit plaques | 32 patients with an index NSTEMI who successfully underwent three vessel OCT | 32 consecutive patients in a single centre who were undergoing PCI of at least one coronary lesion were prospectively enrolled Patients underwent three vessel OCT at the time of PCI with a 20 mm/s pullback speed that was applied during automated injection of intracoronary iso-osmolar contrast media |
|
Majeed et al (2020)80 | To study the association between NaF PET uptake and high-risk plaque features on OCT and CTCA, and the potential application to patient-level risk stratification | 62 patients with ACS were recruited prospectively | Patients underwent multivessel OCT at the time of angiography, with subsequent CTCA and NaF PET within a maximum time window of up to 4 weeks A low-risk cohort (n = 10) with no prior coronary artery disease and calcium score of 0 were used to determine the upper limit of the normal reference range of radiotracer activity for each coronary segment based on 18- segment model. After co-registration, a total of 286 coronary segments were analysed for association between NaF activity and high-risk OCT and CTCA plaque features Patient-level data were analysed based on number of coronary segments with NaF positivity |
|
Author . | Aim . | Sample . | Methods . | Results . |
---|---|---|---|---|
MacMeill (2004)67 | Using OCT to investigate the relationship between macrophages distributions and clinical coronary syndromes | 49 subjects undergoing single vessel stenting for de novo coronary artery disease | OCT images were acquired at 4 frames/s during intermittent saline injections After automated segmentation of fibroatheroma cap comparison between the surface (<50 μm from the lumen) and subsurface (>50 μm) macrophage densities of the was performed. Macrophage density was studied using the NSD of the OCT signal |
|
Raffel (2007)68 | To evaluate the relationship between peripheral WBC count, plaque fibrous cap macrophage density and the presence of TCFA on OCT | 43 patients undergoing coronary angiography | Macrophage density was assessed as the average of the NSD values within the segmented/ROI of the cap (mean NSD) For individual plaques, the value of the highest macrophage density obtained from the 3 sampling sites was used for subsequent data analysis WBC counts were obtained in all cases |
|
Minami (2015)69 | To investigate the clinical significance of bright spots in coronary plaque detected by OCT in patients with coronary artery disease | 112 patients (50 ACS and 62 SAP) | Macrophage density was calculated as the number of bright spots divided by the total number of analysed pixels with the 5mm span of vessel centred around the culprit frame (a total of 25 cross-sectional frames, including the culprit frame and 12 frames on both proximal and distal sides). Macrophage location was considered superficial (≤250 μm) or deep (≤1000 μm) |
|
Galon (2015)70 | To compare OCT volumetric quantification of fibrous cap and macrophage detection using visual assessment and automated image processing algorithms in non-culprit lesions | 67 consecutive patients with STEMI and SAP | FC was manually delineated by a computer-aided method and automatically classified into three thickness categories: fibrous cap <65 μm, TCFA 65–150 μm, and >150 μm. Automated detection and quantification of macrophage was also performed |
|
Park (2017)71 | To investigate whether OCT markers of plaque vulnerability predict cardiac allograft vasculopathy after heart transplantation | 34 consecutive patients | Presence of vulnerability markers (lipid pools, TCFA, macrophages and microchannels) was assessed in 100 consecutive frames in 20 mm proximal segments of the LAD The total number of appearances of vulnerable markers was defined as a vulnerability score (VS). Plaque volume (PV) was measured in the same coronary segment using IVUS at baseline and 1-year follow-up and the association between the baseline VS, and the change in percent PV (PV/vessel volume × 100 [%PV]) was evaluated |
|
Taglieri (2017)72 | To evaluate the relationship between aortic inflammation (as assessed by 18F-FDG PET) and features of plaque vulnerability (as assessed by OCT) | 30 consecutive patients with ACS undergoing PCI | All patients underwent 3 vessel OCT before intervention and 18F-FGD-PET before discharge 18F-FDG uptake in both ascending and descending aorta was measured as averaged mean and maximum TBR or as active slices (TBRmax ≥1.6) |
|
Lannaccone (2018)73 | To evaluate the clinical impact of OCT-detected culprit plaque features in ACS | 209 patients with ACS | Retrospective analysis of a multi-centre registry All ACS patients undergoing OCT of culprit and non-culprit plaque with follow-up conducted through clinical visits or phone calls at 6 months |
|
Joner (2018)74 | To assess neo-atherosclerosis in patients presenting with stent thrombosis using OCT | 134 patients presenting with very late stent thrombosis | Plaque morphology was analysed in every other frame. The presence of fibroatheroma and signal attenuation indicative of macrophages (at least 1 quadrant) was recorded |
|
Okamoto (2019)75 | To study the relationship between healed plaques and the development of significant coronary stenosis in SAP | 205 SAP patients in retrospective arm 42 samples from 18 SAP in prospective arm | In the retrospective arm, the prevalence and clinical characteristics of OCT-based layered plaque at the culprit site in patients with SAP was assessed In the prospective arm, the histopathological characteristics of layered plaques in SAP lesions were identified using DCA samples |
|
Wu et al (2019)76 | To investigate the correlation between insulin resistance and culprit plaque characteristics using OCT in patients with ACS | 145 patients with ACS | 159 culprit plaques in 145 patients were identified. Patient were identified into 4 groups according to the homeostasis model assessment of insulin resistance (HOMA-IR) |
|
Raber et al (2019)77 | To study changes in OCT-defined morphological changes in patients with STEMI receiving high-intensity statin therapy | 103 patients with STEMI | 103 patients with STEMI undergoing IVUS and OCT of two non-infarct related arteries and a repeat coronary angiography at 13 months were recruited Co-primary endpoints for OCT analysis were change in minimum FCT and macrophage accumulation angle Patients received 20 mg of rosuvastatin daily for the first 2 weeks and were then up-titrated to 40 mg daily |
|
Prati et al (2020)78 | To investigate the predictive value of high-risk OCT plaque features including macrophages | 1003 patient with coronary artery disease undergoing PCI | The predictive value of high-risk OCT plaque features including MLA, FCT, lipid arc circumferential extension and the presence of OCT-detected macrophages was studied in a proximal LAD segment The primary endpoint of target-segment MI and/or cardiac death was studied at 1-year follow-up |
|
Gatto et al (2020)60 | To revise the clinical and demographic variables of patients who have coronary plaques with macrophages, and investigate the reproducibility of quantitative assessment | Post-hoc analysis of the CLIMA study | A total of 577 patients out of 1003 showed macrophage accumulation. Three groups were identified: Group 1 (426)—without macrophages Group 2 (296)—patients with low macrophage content (less than median value of 67° of circumferential arc) Group 3 (281)—patients with high macrophage content (more than median value of 67° of circumferential arc) |
|
Taglieri et al (2020)79 | To investigate the prevalence and the features of OCT-detected macrophage accumulation in culprit plaques as compared with non-culprit plaques | 32 patients with an index NSTEMI who successfully underwent three vessel OCT | 32 consecutive patients in a single centre who were undergoing PCI of at least one coronary lesion were prospectively enrolled Patients underwent three vessel OCT at the time of PCI with a 20 mm/s pullback speed that was applied during automated injection of intracoronary iso-osmolar contrast media |
|
Majeed et al (2020)80 | To study the association between NaF PET uptake and high-risk plaque features on OCT and CTCA, and the potential application to patient-level risk stratification | 62 patients with ACS were recruited prospectively | Patients underwent multivessel OCT at the time of angiography, with subsequent CTCA and NaF PET within a maximum time window of up to 4 weeks A low-risk cohort (n = 10) with no prior coronary artery disease and calcium score of 0 were used to determine the upper limit of the normal reference range of radiotracer activity for each coronary segment based on 18- segment model. After co-registration, a total of 286 coronary segments were analysed for association between NaF activity and high-risk OCT and CTCA plaque features Patient-level data were analysed based on number of coronary segments with NaF positivity |
|
ACS, acute coronary syndrome; CI, confidence interval; CTCA, CT coronary angiography; FCT, fibrous cap thickness; HR, hazard ratio; IVUS, intravascular ultrasound; LAD, left anterior descending artery; MI, myocardial infarction; MLA, minimal luminal area; NaF, 18F-sodium fluoride; NSD, normalized-intensity standard deviation; OCT, optical coherence tomography; PCI, percutaneous coronary intervention; PET, positron emission tomography; ROI, region of interest; SAP, stable angina pectoris; STEMI, ST-segment elevation myocardial infarction; TBR, target-to-background ratio; TCFA, thin cap fibroatheroma; WBC, white blood cell.
Intracoronary polarimetry using modified optical frequency domain imaging
An emerging technology that utilizes the polarization of light is based on a modification of existing optical frequency domain OCT systems. The polarization of infrared light is influenced by microscopic structures of the arterial wall. Modification of the OCT apparatus and image reconstruction methods have allowed polarimetric measurements to be obtained simultaneously with conventional cross-sectional imaging through standard OCT catheters.
Villiger et al.81 performed the first histological validation of this technique utilizing coronaries arteries of 5 human cadaveric hearts, demonstrating different polarization signatures caused by various tissue types including macrophages. Subsequently, Otsuka et al.82 validated first-in-human intracoronary polarimetry in 12 patients with ACS and 18 patients with stable angina. This study demonstrated that culprit lesions in ACS and or ruptured plaques exhibited lower birefringence than in the caps of target lesions in patients with stable angina. Furthermore, birefringence of these fibrous caps was associated with increased normalized standard deviation (NSD) on OCT analysis, suggesting macrophage accumulation in these lesions. Current data are limited and further validation studies are needed.
Combined OCT and near-infrared autofluorescence
Inflammation increases the risk of (and is exacerbated by) intraplaque haemorrhage, resulting in macrophage-dependent breakdown of extra-vascular red blood cells. Htun et al.83 in pre-clinical work demonstrated that near-infrared autofluorescence (NIRAF) can identify intraplaque haemorrhage and haeme-degradation products, a feature of plaque instability using a mouse model and 50 human carotid endarterectomy samples. Ughi et al.84 for the first time demonstrated the safety and feasibility of high-quality OCT and NIRAF images simultaneously, using a combined catheter in 12 patients undergoing PCI. High NIRAF signals (defined as maximum normalized NIRAF intensity >0.4) were associated with more vulnerable plaque phenotypes as characterized by OCT, namely TCFA and plaque rupture. Comparison of OCT and NIRAF lipid arcs revealed that high NIRAF intensity was restricted to regions of plaque that had dense macrophage accumulations, as determined by the OCT-based NSD parameters (see Figure 2). This report is of major interest because it indicates the ability to deliver complementary imaging techniques simultaneously and safely within the coronary arteries. These novel intracoronary optical imaging-based plaque characterization techniques have potential clinical applications in guiding treatment and prognostication but are awaiting longitudinal outcome studies to determine the potential additive clinical value.
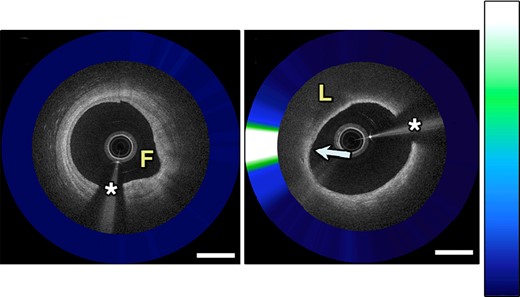
Combined intravascular coronary OCT with NIRAF demonstrates a fibrotic plaque with low NIRAF signal (A) and a fibroatheroma with high NIRAF signal corresponding to dense macrophage accumulation (B). Adapted with permission from Ughi et al.84 The asterisk (*) corresponds with catheter artefact. F, fibrotic; L, lipid.
Quantification of plaque characteristics on OCT including macrophages is time-consuming and remains at present a research tool confined to specialist centres. Progress is being made with automated image segmentation and plaque quantification that will democratize these techniques in the near future.85 Light-based invasive imaging techniques provide very high-resolution information on a broad range of high-risk plaque characteristics but is limited to the proximal and mid arterial segments where the imaging catheters can be safely located (which fortuitously, is also where most high-risk plaque are anatomically located). OCT has persuasive evidence that it could be used clinically for further nuanced risk prediction, particularly in in-patients with acute coronary syndromes or other settings where there is a clinical indication for invasive coronary angiography. In the outpatient setting, where stable coronary disease predominates, complimentary approaches are required based on non-invasive imaging techniques.
Computed tomography imaging
Quantification of plaque burden using computed tomography (CT) calcium scoring predicts cardiovascular outcomes across multiple vascular beds including the aorta, carotid, femoral, and coronary arteries.86 Recent developments indicate that CT can also indirectly detect inflammation by examining perivascular fat. Perivascular fat exists in two phases, an ‘aqueous’ phase and a ‘lipid’ phase. Inflammation in epicardial fat causes the aqueous phase to dominate with an associated increase in X-ray attenuation. It has been proposed that this represents the effects of a nearby inflammatory coronary artery lesion (see Figure 3). Perivascular fat on CT independently predicts the progression of non-calcified and total plaque burden on follow-up CT, correlates with 18F-sodium fluoride (NaF) positron emission tomography (PET) uptake in coronary plaque and may improve risk prediction of cardiovascular mortality compared with standard risk assessment models.87–89 However, there are still inherent limitations including a lack of specificity (as it may simply represent the effects of visceral fat rather than arterial inflammation) and is not assessable in many vascular territories.87
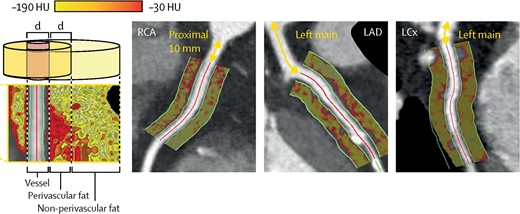
Perivascular fat attenuation analysis of the proximal epicardial coronary vessels as defined by fat within a radial distance of the diameter (d) of the vessel. Adapted with permission from Oikonomou et al.87 HU, Hounsfield units; RCA, right coronary artery; LAD, left anterior descending artery; LCx, left circumflex artery.
Perivascular fat imaging with CT will be improved by machine learning methods to provide more accurate and less demanding workflows.90 While machine learning remains in its infancy, it is likely to surpass conventional image assessment.91 At present, conventional assessment of cardiovascular disease by CT is validated, yet imprecise at the patient-level, providing downstream assessment of already significantly advanced cardiovascular disease. Molecular imaging provides an alternative and uniquely targeted imaging approach to gain fine insight into coronary physiology and inflammation.
Functional imaging of atherosclerotic inflammation by PET
Several PET tracers are being assessed for their role in understanding atherosclerosis, and to find translational opportunities for future clinical therapies. Many/most targets for molecular imaging have been developed through in vitro experiments and animal studies and have not been validated in humans in vivo. Tracers that have been studied in humans may be non-specific, e.g. targeting metabolism or a consequence of inflammation (such as minerals and hypoxia) whilst a small number detected macrophage-specific receptors or functions. These tracers and their targets will be discussed in more detail in the following section and are highlighted in Figure 4.
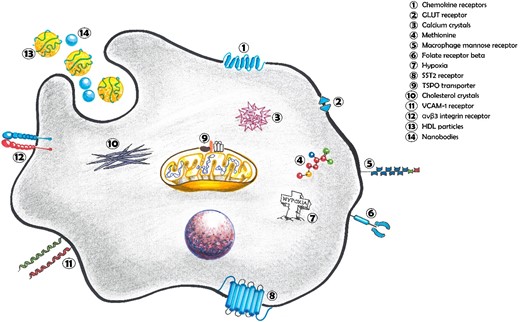
Macrophage PET tracer targets in atherosclerosis. Chemokine receptors, major regulator of macrophage chemotaxis; GLUT receptor, transport protein involved in glucose translocation across the cell membrane; Calcium crystals, accumulated intra-cellular calcium phosphate crystals; Methionine, essential amino acid associated with polarization of M1-like macrophages; Macrophage mannose receptor, acts as a pattern recognition receptor for various carbohydrates resulting in immune activation against a variety of pathogens; Folate receptor beta, high-affinity receptor for the uptake of folate into macrophages; Hypoxia, Hypoxia-inducible factor-1α is a key transcription factor in cellular responses to hypoxia and promotes M1-like polarization; SST2 receptor, influences cell proliferation, cytokine release and immunoglobulin production; TSPO transporter, mitochondrial membrane protein that handles cholesterol and impacts on immune cell function; cholesterol crystals, phagocytosed intra-cellular cholesterol forming ‘foam cells’; VCAM-1 receptor, modulates cellular adhesion and facilitates migration of macrophages; αvβ3 integrin receptor, regulates macrophage differentiation and transendothelial migration; HDL particles, circulating lipoproteins that carry accumulated lipid molecules; Nanobodies; engineered high-affinity antigen-binding fragments.
18F-fluorodeoxyglucose (FDG) is a tracer taken up by metabolically active cells due to its similar structure to glucose and is most commonly used for diagnosis and surveillance of cancer. It is widely available with well-established methods, is relatively undemanding to produce and has been used as an endpoint in trials of novel pharmaceutical agents.92,93 Uptake of FDG in large arteries was first reported in 2001, with a subsequent study demonstrating that patients with ≥1 cardiovascular risk factor showed higher FDG uptake in the iliac and femoral arteries (but not the abdominal aorta), when compared with patients without risk factors.94,95 A landmark prospective study in 2013 demonstrated that significant vascular uptake of FDG at baseline was associated with subsequent arterial calcification at the same location within a period of 1–5 years, a finding that reflects increasing cardiovascular risk.96,97 FDG uptake in large arteries is associated with increased macrophage accumulation and statin therapy results in a reduced arterial wall FDG uptake.98,99
Unfortunately, FDG PET has several significant limitations that prevent reliable assessment of coronary arteries. These include variable and high-intensity myocardial uptake of FDG with significant spillover preventing coronary assessment, poor tolerability and reliability of dietary techniques to switch the myocardium to free fatty acid metabolism, and lack of cell specificity due to FDG uptake in all metabolically active tissue.100–102 While FDG PET has served a valuable role in advancing our knowledge of vascular inflammation, a more specific and reliable tracer that can overcome these limitations is highly desirable.
18F-sodium fluoride (NaF) binds hydroxyapatite (the dominant form of calcium in the body) and localizes to microcalcifications in the arterial wall. In a prospective study, both FDG and NaF PET scans were performed in 80 patients with either ACS or stable angina. Culprit plaques showed significant NaF uptake in >90% of patients with ACS and in nearly half of patients with stable angina, with high-risk plaque characteristics by intravascular ultrasound (IVUS), such as positive remodelling, a large necrotic core and greater microcalcification more likely to be demonstrated in these lesions. NaF PET was superior to FDG PET in identifying vulnerable coronary artery plaques, largely due to difficulties with interpreting FDG uptake despite techniques aimed to suppress myocardial activity.101 NaF uptake in coronary lesions is likewise associated with high-risk plaque features on OCT and IVUS, closely correlates to the Framingham risk score for the prediction of cardiovascular events and predicts 2-year cardiovascular event rates (see Figure 5).80,104–109
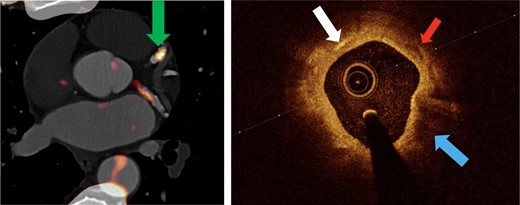
OCT features of high-risk atherosclerotic plaque associated with 18F-NaF PET uptake. The region of increased 18F-NaF activity in the left anterior descending artery (left), green arrow, is imaged with OCT (right). This region has multiple high-risk plaque features on OCT. Adapted with permission from Bellinge et al.103 White arrow indicates cholesterol crystals; red arrow indicates microcalcifications; blue arrow indicates lipid-rich plaque.
There are disadvantages—however—while NaF detects microcalcification activity, a process correlated with atherosclerosis, it does not directly measure inflammation but rather a downstream consequence of chronic inflammation. The assessment of structures adjacent to bone, such as the aorta, can also be difficult due to significant spillover from the bone. Nonetheless NaF imaging has conclusively shown that PET imaging can reliably detect high-risk plaque in coronary arteries and has led to the development and continued refinement of coronary-specific PET imaging, reconstruction, and image analysis techniques. Several centres around the world have independently implemented these approaches successfully, representing a breakthrough of our understanding of coronary PET imaging and which can also be applied to other PET tracers more specific to inflammatory markers.110 Progress is also being made with volumetric quantification of tracer uptake rather than peak intensity measurements which, intuitively, is more relevant to vascular assessment.
Local lesional hypoxia has also led to hypoxia-specific PET tracers, currently used for oncology purposes, being examined in atherosclerosis. 18F-fluoromisonidazole (FMISO) is the first and most widely studied of these tracers and has shown promise in both rabbit and human models though does not seem to correlate with cardiovascular risk factors.111,112 Unfortunately, hypoxia-specific tracers such as FMISO may remain limited in their application to atherosclerosis by their poor sensitivity in identifying subtle vascular hypoxia, rather than hypoxia as typically identified in the necrotic tumour core. Additionally, the slow blood pool clearance of FMISO is unfavourable for patient imaging as a delay of several hours from injection to scanning is required, combined with a relatively high administered activity resulting in a moderate radiation dose to patients. More refined tracers that attempt to address these concerns continue to be developed in the hope of addressing these technical considerations.113
Identifying macrophages using targeted tracers
Currently used in the diagnosis and monitoring of patients with neuroendocrine tumours, gallium-68-labeled DOTATATE is a PET ligand with high-specificity binding affinity to somatostatin receptor subtype-2 (SST2). Importantly, up-regulation of SST2 has been demonstrated on the surface of activated macrophages and the highly-specific binding of 68Ga-DOTATATE to M1 macrophages in humans was prospectively validated in 2017.114
Tarkin et al. examined 42 patients with atherosclerosis and compared the discriminatory ability of DOTATATE PET to FDG PET.115 In patients with symptomatic carotid artery disease, DOTATATE PET was able to discriminate culprit vs. non-culprit carotid specimens with histological localization to macrophages in the necrotic core and plaque shoulders. In patients with both stable angina and recent ACS, DOTATATE PET was able to correctly identify culprit plaques and was more accurate than FDG PET in discriminating high-risk vs. low-risk plaques. Promisingly, both inter- and intra-observer reliability was excellent and interpretation of DOTATATE uptake was possible in all coronary lesions without myocardial or bone spillover (as occurs with FDG and NaF PET, respectively).114 These very promising results are yet to be validated by other groups. Several other tracers also target SST2 receptors on macrophages and may have some advantages and disadvantages but are in earlier stages of development (see Table 3).
Imaging modality . | . | . | Mechanism of action . | Advantages . | Disadvantages . |
---|---|---|---|---|---|
PET tracers | Cell metabolism | 18F-FDG94–96,116,117 | Uptake into cells with increased metabolic rates | Inexpensive and clinically available Prospectively studied in multiple human trials | Myocardial spillover frequently precludes coronary assessment Poor specificity—uptake by all metabolically active cells |
11C-methionine118 | Amino acid metabolism of macrophages | Strongly correlated with cardiovascular risk factors and CRP | Limited observational human data Uptake also seen in other cells (such as myofibroblasts) | ||
Microcalcification | 18F-NaF101 | Microcalcification as a result of necrosis or inflammation | Widely available with well-established safety High signal uptake with low background activity Reliably distinguishes culprit plaques | Downstream assessment of effects of chronic inflammation Validity of risk prediction and longitudinal monitoring capabilities not yet established | |
Hypoxia | 18F-HX4119 18F-FMISO111,112 62Cu-ATSM120 | Trapped within cells in hypoxic state | Correlates well with areas of vessel hypoxia and increased macrophage density Validated in humans against 18F-FDG uptake in symptomatic carotid artery disease | Indirect assessment of atherosclerosis Requires further prospective and comparative research | |
Cell surface receptors Cell surface receptors Cell surface receptors | 68Ga-DOTATATE114,121,122 64Cu-DOTATATE123,124 68Ga-DOTANOC122 18F-FDR-NOC122 68Ga-DOTATOC124,125 | Activated macrophages expressing somatostatin receptor 2 (SST2) | Widely available with well-established safety High signal uptake with low background activity Minimal spillover by adjacent structures | Validity of risk prediction and longitudinal monitoring capabilities not yet established | |
68Ga-pentixafor126–131 | C-X-C chemokine receptor 4 (CXCR4) | Histological data confirming uptake in atherosclerotic plaque Retrospective observational human data | Precise role of CXCR4 in inflammation remains conflicting Low specificity with broad uptake by numerous cell types involved in atherosclerosis | ||
64Cu-DOTA-DAPTA-comb nanoparticles132 | C–C chemokine receptor 5 (CCR5) | Sensitive and specific for plaque in mouse models of atherosclerosis | No in-human data High blood pool signal Longitudinal cell expression of CCR5 in the progression of atherosclerosis remains unclear | ||
64Cu-DOTA-ECL1i133,134 | C–C chemokine receptor 2 (CCR2) | Validated in mice experiments of aortic transplantation and plaque regression Largely targets M2-like macrophages which may enable assessment of response to therapies | No in-human data available Further studies required to assess fate and role of cells highlighted | ||
64Cu-DOTA-vMIP-II135 64Cu-vMIP-II-comb nanoparticles136 | Multiple simultaneous chemokine receptors | Broad chemokine receptor targeting improves specificity and sensitivity Promising detection of vascular injury in mice models of atherosclerosis | No in-human data available | ||
18F-FOL137 | Folate receptor β selectively expressed on macrophages | Rapid blood pool clearance Low liver, lung and myocardial uptake Proof-of-principle ex vivo human study | Limited immunohistochemical correlation data available Further assessment of receptor expression required in human atherosclerosis | ||
18F-4V138 64Cu-VCAM nanobody139 | Vascular cell adhesion molecule-1 (VCAM-1) | Highly correlated with increasing disease severity in animal models of atherosclerosis Rapid renal excretion of nanobodies promotes improved blood pool characteristics | No in-human data | ||
68Ga-MMR139 | Macrophage mannose receptor (MMR) | Correlates well when compared with 18F-FDG in rabbit aortic atherosclerosis Rapid renal excretion of nanobodies promotes improved blood pool characteristics | Poorly established role in the assessment of atherosclerosis given predominant uptake in M2-like macrophages No in-human data | ||
18F-fluciclatide140 18F-Galacto-RGD141,142 18F-Flotegatide143 | Upregulated αvβ3 integrin receptor at areas of intraplaque angiogenesis and neovascularization | Highly correlated and co-localizes with macrophage-rich atherosclerotic plaque Validated uptake in both histopathological and in vivo human studies | Validity of risk prediction and longitudinal monitoring capabilities not yet established | ||
Mitochondrial membrane protein | 11C-PK11195144 18F-FEDAA1106145 18F-FEMPA146 18F-GE-180147 | Translocator protein (TSPO) | Highly expressed in human macrophages (though not uniquely) Rapid blood clearance | Low target-to-background ratio (similar to FDG) Genetic polymorphisms influence binding in some tracers High uptake in lung and myocardium | |
Phagocytosis | 89Zr-modified dextran nanoparticles148 | Sugar-based nanoparticle | Clinically approved components Nanoparticles can simultaneously deliver therapeutic payloads | No in vivo human data Poor specificity for macrophages Slow clearance from blood pool | |
18F-Macroflor149 | Rapid clearance from blood pool Nanoparticles can simultaneously deliver therapeutic payloads Low myocardial uptake Highly localized to macrophage-rich plaques in multiple animal models | No in-human data (though assessed in primates) Uptake by both M1 and M2-like macrophages Safety and translation in humans require further research | |||
89Zr-HDL nanoparticles150 | HDL | Localizes to atherosclerotic plaque in multiple animal models | Very long circulating time No in-human data Influenced by circulating lipoprotein concentration | ||
MRI | Ultrasmall paramagnetic particles of iron oxide151–153 | Accumulates in macrophages after being phagocytosed | Coincides with active plaque disease and intense macrophage infiltration | Non-specific uptake into both M1 and M2-like macrophages Less sensitive than PET |
Imaging modality . | . | . | Mechanism of action . | Advantages . | Disadvantages . |
---|---|---|---|---|---|
PET tracers | Cell metabolism | 18F-FDG94–96,116,117 | Uptake into cells with increased metabolic rates | Inexpensive and clinically available Prospectively studied in multiple human trials | Myocardial spillover frequently precludes coronary assessment Poor specificity—uptake by all metabolically active cells |
11C-methionine118 | Amino acid metabolism of macrophages | Strongly correlated with cardiovascular risk factors and CRP | Limited observational human data Uptake also seen in other cells (such as myofibroblasts) | ||
Microcalcification | 18F-NaF101 | Microcalcification as a result of necrosis or inflammation | Widely available with well-established safety High signal uptake with low background activity Reliably distinguishes culprit plaques | Downstream assessment of effects of chronic inflammation Validity of risk prediction and longitudinal monitoring capabilities not yet established | |
Hypoxia | 18F-HX4119 18F-FMISO111,112 62Cu-ATSM120 | Trapped within cells in hypoxic state | Correlates well with areas of vessel hypoxia and increased macrophage density Validated in humans against 18F-FDG uptake in symptomatic carotid artery disease | Indirect assessment of atherosclerosis Requires further prospective and comparative research | |
Cell surface receptors Cell surface receptors Cell surface receptors | 68Ga-DOTATATE114,121,122 64Cu-DOTATATE123,124 68Ga-DOTANOC122 18F-FDR-NOC122 68Ga-DOTATOC124,125 | Activated macrophages expressing somatostatin receptor 2 (SST2) | Widely available with well-established safety High signal uptake with low background activity Minimal spillover by adjacent structures | Validity of risk prediction and longitudinal monitoring capabilities not yet established | |
68Ga-pentixafor126–131 | C-X-C chemokine receptor 4 (CXCR4) | Histological data confirming uptake in atherosclerotic plaque Retrospective observational human data | Precise role of CXCR4 in inflammation remains conflicting Low specificity with broad uptake by numerous cell types involved in atherosclerosis | ||
64Cu-DOTA-DAPTA-comb nanoparticles132 | C–C chemokine receptor 5 (CCR5) | Sensitive and specific for plaque in mouse models of atherosclerosis | No in-human data High blood pool signal Longitudinal cell expression of CCR5 in the progression of atherosclerosis remains unclear | ||
64Cu-DOTA-ECL1i133,134 | C–C chemokine receptor 2 (CCR2) | Validated in mice experiments of aortic transplantation and plaque regression Largely targets M2-like macrophages which may enable assessment of response to therapies | No in-human data available Further studies required to assess fate and role of cells highlighted | ||
64Cu-DOTA-vMIP-II135 64Cu-vMIP-II-comb nanoparticles136 | Multiple simultaneous chemokine receptors | Broad chemokine receptor targeting improves specificity and sensitivity Promising detection of vascular injury in mice models of atherosclerosis | No in-human data available | ||
18F-FOL137 | Folate receptor β selectively expressed on macrophages | Rapid blood pool clearance Low liver, lung and myocardial uptake Proof-of-principle ex vivo human study | Limited immunohistochemical correlation data available Further assessment of receptor expression required in human atherosclerosis | ||
18F-4V138 64Cu-VCAM nanobody139 | Vascular cell adhesion molecule-1 (VCAM-1) | Highly correlated with increasing disease severity in animal models of atherosclerosis Rapid renal excretion of nanobodies promotes improved blood pool characteristics | No in-human data | ||
68Ga-MMR139 | Macrophage mannose receptor (MMR) | Correlates well when compared with 18F-FDG in rabbit aortic atherosclerosis Rapid renal excretion of nanobodies promotes improved blood pool characteristics | Poorly established role in the assessment of atherosclerosis given predominant uptake in M2-like macrophages No in-human data | ||
18F-fluciclatide140 18F-Galacto-RGD141,142 18F-Flotegatide143 | Upregulated αvβ3 integrin receptor at areas of intraplaque angiogenesis and neovascularization | Highly correlated and co-localizes with macrophage-rich atherosclerotic plaque Validated uptake in both histopathological and in vivo human studies | Validity of risk prediction and longitudinal monitoring capabilities not yet established | ||
Mitochondrial membrane protein | 11C-PK11195144 18F-FEDAA1106145 18F-FEMPA146 18F-GE-180147 | Translocator protein (TSPO) | Highly expressed in human macrophages (though not uniquely) Rapid blood clearance | Low target-to-background ratio (similar to FDG) Genetic polymorphisms influence binding in some tracers High uptake in lung and myocardium | |
Phagocytosis | 89Zr-modified dextran nanoparticles148 | Sugar-based nanoparticle | Clinically approved components Nanoparticles can simultaneously deliver therapeutic payloads | No in vivo human data Poor specificity for macrophages Slow clearance from blood pool | |
18F-Macroflor149 | Rapid clearance from blood pool Nanoparticles can simultaneously deliver therapeutic payloads Low myocardial uptake Highly localized to macrophage-rich plaques in multiple animal models | No in-human data (though assessed in primates) Uptake by both M1 and M2-like macrophages Safety and translation in humans require further research | |||
89Zr-HDL nanoparticles150 | HDL | Localizes to atherosclerotic plaque in multiple animal models | Very long circulating time No in-human data Influenced by circulating lipoprotein concentration | ||
MRI | Ultrasmall paramagnetic particles of iron oxide151–153 | Accumulates in macrophages after being phagocytosed | Coincides with active plaque disease and intense macrophage infiltration | Non-specific uptake into both M1 and M2-like macrophages Less sensitive than PET |
Imaging modality . | . | . | Mechanism of action . | Advantages . | Disadvantages . |
---|---|---|---|---|---|
PET tracers | Cell metabolism | 18F-FDG94–96,116,117 | Uptake into cells with increased metabolic rates | Inexpensive and clinically available Prospectively studied in multiple human trials | Myocardial spillover frequently precludes coronary assessment Poor specificity—uptake by all metabolically active cells |
11C-methionine118 | Amino acid metabolism of macrophages | Strongly correlated with cardiovascular risk factors and CRP | Limited observational human data Uptake also seen in other cells (such as myofibroblasts) | ||
Microcalcification | 18F-NaF101 | Microcalcification as a result of necrosis or inflammation | Widely available with well-established safety High signal uptake with low background activity Reliably distinguishes culprit plaques | Downstream assessment of effects of chronic inflammation Validity of risk prediction and longitudinal monitoring capabilities not yet established | |
Hypoxia | 18F-HX4119 18F-FMISO111,112 62Cu-ATSM120 | Trapped within cells in hypoxic state | Correlates well with areas of vessel hypoxia and increased macrophage density Validated in humans against 18F-FDG uptake in symptomatic carotid artery disease | Indirect assessment of atherosclerosis Requires further prospective and comparative research | |
Cell surface receptors Cell surface receptors Cell surface receptors | 68Ga-DOTATATE114,121,122 64Cu-DOTATATE123,124 68Ga-DOTANOC122 18F-FDR-NOC122 68Ga-DOTATOC124,125 | Activated macrophages expressing somatostatin receptor 2 (SST2) | Widely available with well-established safety High signal uptake with low background activity Minimal spillover by adjacent structures | Validity of risk prediction and longitudinal monitoring capabilities not yet established | |
68Ga-pentixafor126–131 | C-X-C chemokine receptor 4 (CXCR4) | Histological data confirming uptake in atherosclerotic plaque Retrospective observational human data | Precise role of CXCR4 in inflammation remains conflicting Low specificity with broad uptake by numerous cell types involved in atherosclerosis | ||
64Cu-DOTA-DAPTA-comb nanoparticles132 | C–C chemokine receptor 5 (CCR5) | Sensitive and specific for plaque in mouse models of atherosclerosis | No in-human data High blood pool signal Longitudinal cell expression of CCR5 in the progression of atherosclerosis remains unclear | ||
64Cu-DOTA-ECL1i133,134 | C–C chemokine receptor 2 (CCR2) | Validated in mice experiments of aortic transplantation and plaque regression Largely targets M2-like macrophages which may enable assessment of response to therapies | No in-human data available Further studies required to assess fate and role of cells highlighted | ||
64Cu-DOTA-vMIP-II135 64Cu-vMIP-II-comb nanoparticles136 | Multiple simultaneous chemokine receptors | Broad chemokine receptor targeting improves specificity and sensitivity Promising detection of vascular injury in mice models of atherosclerosis | No in-human data available | ||
18F-FOL137 | Folate receptor β selectively expressed on macrophages | Rapid blood pool clearance Low liver, lung and myocardial uptake Proof-of-principle ex vivo human study | Limited immunohistochemical correlation data available Further assessment of receptor expression required in human atherosclerosis | ||
18F-4V138 64Cu-VCAM nanobody139 | Vascular cell adhesion molecule-1 (VCAM-1) | Highly correlated with increasing disease severity in animal models of atherosclerosis Rapid renal excretion of nanobodies promotes improved blood pool characteristics | No in-human data | ||
68Ga-MMR139 | Macrophage mannose receptor (MMR) | Correlates well when compared with 18F-FDG in rabbit aortic atherosclerosis Rapid renal excretion of nanobodies promotes improved blood pool characteristics | Poorly established role in the assessment of atherosclerosis given predominant uptake in M2-like macrophages No in-human data | ||
18F-fluciclatide140 18F-Galacto-RGD141,142 18F-Flotegatide143 | Upregulated αvβ3 integrin receptor at areas of intraplaque angiogenesis and neovascularization | Highly correlated and co-localizes with macrophage-rich atherosclerotic plaque Validated uptake in both histopathological and in vivo human studies | Validity of risk prediction and longitudinal monitoring capabilities not yet established | ||
Mitochondrial membrane protein | 11C-PK11195144 18F-FEDAA1106145 18F-FEMPA146 18F-GE-180147 | Translocator protein (TSPO) | Highly expressed in human macrophages (though not uniquely) Rapid blood clearance | Low target-to-background ratio (similar to FDG) Genetic polymorphisms influence binding in some tracers High uptake in lung and myocardium | |
Phagocytosis | 89Zr-modified dextran nanoparticles148 | Sugar-based nanoparticle | Clinically approved components Nanoparticles can simultaneously deliver therapeutic payloads | No in vivo human data Poor specificity for macrophages Slow clearance from blood pool | |
18F-Macroflor149 | Rapid clearance from blood pool Nanoparticles can simultaneously deliver therapeutic payloads Low myocardial uptake Highly localized to macrophage-rich plaques in multiple animal models | No in-human data (though assessed in primates) Uptake by both M1 and M2-like macrophages Safety and translation in humans require further research | |||
89Zr-HDL nanoparticles150 | HDL | Localizes to atherosclerotic plaque in multiple animal models | Very long circulating time No in-human data Influenced by circulating lipoprotein concentration | ||
MRI | Ultrasmall paramagnetic particles of iron oxide151–153 | Accumulates in macrophages after being phagocytosed | Coincides with active plaque disease and intense macrophage infiltration | Non-specific uptake into both M1 and M2-like macrophages Less sensitive than PET |
Imaging modality . | . | . | Mechanism of action . | Advantages . | Disadvantages . |
---|---|---|---|---|---|
PET tracers | Cell metabolism | 18F-FDG94–96,116,117 | Uptake into cells with increased metabolic rates | Inexpensive and clinically available Prospectively studied in multiple human trials | Myocardial spillover frequently precludes coronary assessment Poor specificity—uptake by all metabolically active cells |
11C-methionine118 | Amino acid metabolism of macrophages | Strongly correlated with cardiovascular risk factors and CRP | Limited observational human data Uptake also seen in other cells (such as myofibroblasts) | ||
Microcalcification | 18F-NaF101 | Microcalcification as a result of necrosis or inflammation | Widely available with well-established safety High signal uptake with low background activity Reliably distinguishes culprit plaques | Downstream assessment of effects of chronic inflammation Validity of risk prediction and longitudinal monitoring capabilities not yet established | |
Hypoxia | 18F-HX4119 18F-FMISO111,112 62Cu-ATSM120 | Trapped within cells in hypoxic state | Correlates well with areas of vessel hypoxia and increased macrophage density Validated in humans against 18F-FDG uptake in symptomatic carotid artery disease | Indirect assessment of atherosclerosis Requires further prospective and comparative research | |
Cell surface receptors Cell surface receptors Cell surface receptors | 68Ga-DOTATATE114,121,122 64Cu-DOTATATE123,124 68Ga-DOTANOC122 18F-FDR-NOC122 68Ga-DOTATOC124,125 | Activated macrophages expressing somatostatin receptor 2 (SST2) | Widely available with well-established safety High signal uptake with low background activity Minimal spillover by adjacent structures | Validity of risk prediction and longitudinal monitoring capabilities not yet established | |
68Ga-pentixafor126–131 | C-X-C chemokine receptor 4 (CXCR4) | Histological data confirming uptake in atherosclerotic plaque Retrospective observational human data | Precise role of CXCR4 in inflammation remains conflicting Low specificity with broad uptake by numerous cell types involved in atherosclerosis | ||
64Cu-DOTA-DAPTA-comb nanoparticles132 | C–C chemokine receptor 5 (CCR5) | Sensitive and specific for plaque in mouse models of atherosclerosis | No in-human data High blood pool signal Longitudinal cell expression of CCR5 in the progression of atherosclerosis remains unclear | ||
64Cu-DOTA-ECL1i133,134 | C–C chemokine receptor 2 (CCR2) | Validated in mice experiments of aortic transplantation and plaque regression Largely targets M2-like macrophages which may enable assessment of response to therapies | No in-human data available Further studies required to assess fate and role of cells highlighted | ||
64Cu-DOTA-vMIP-II135 64Cu-vMIP-II-comb nanoparticles136 | Multiple simultaneous chemokine receptors | Broad chemokine receptor targeting improves specificity and sensitivity Promising detection of vascular injury in mice models of atherosclerosis | No in-human data available | ||
18F-FOL137 | Folate receptor β selectively expressed on macrophages | Rapid blood pool clearance Low liver, lung and myocardial uptake Proof-of-principle ex vivo human study | Limited immunohistochemical correlation data available Further assessment of receptor expression required in human atherosclerosis | ||
18F-4V138 64Cu-VCAM nanobody139 | Vascular cell adhesion molecule-1 (VCAM-1) | Highly correlated with increasing disease severity in animal models of atherosclerosis Rapid renal excretion of nanobodies promotes improved blood pool characteristics | No in-human data | ||
68Ga-MMR139 | Macrophage mannose receptor (MMR) | Correlates well when compared with 18F-FDG in rabbit aortic atherosclerosis Rapid renal excretion of nanobodies promotes improved blood pool characteristics | Poorly established role in the assessment of atherosclerosis given predominant uptake in M2-like macrophages No in-human data | ||
18F-fluciclatide140 18F-Galacto-RGD141,142 18F-Flotegatide143 | Upregulated αvβ3 integrin receptor at areas of intraplaque angiogenesis and neovascularization | Highly correlated and co-localizes with macrophage-rich atherosclerotic plaque Validated uptake in both histopathological and in vivo human studies | Validity of risk prediction and longitudinal monitoring capabilities not yet established | ||
Mitochondrial membrane protein | 11C-PK11195144 18F-FEDAA1106145 18F-FEMPA146 18F-GE-180147 | Translocator protein (TSPO) | Highly expressed in human macrophages (though not uniquely) Rapid blood clearance | Low target-to-background ratio (similar to FDG) Genetic polymorphisms influence binding in some tracers High uptake in lung and myocardium | |
Phagocytosis | 89Zr-modified dextran nanoparticles148 | Sugar-based nanoparticle | Clinically approved components Nanoparticles can simultaneously deliver therapeutic payloads | No in vivo human data Poor specificity for macrophages Slow clearance from blood pool | |
18F-Macroflor149 | Rapid clearance from blood pool Nanoparticles can simultaneously deliver therapeutic payloads Low myocardial uptake Highly localized to macrophage-rich plaques in multiple animal models | No in-human data (though assessed in primates) Uptake by both M1 and M2-like macrophages Safety and translation in humans require further research | |||
89Zr-HDL nanoparticles150 | HDL | Localizes to atherosclerotic plaque in multiple animal models | Very long circulating time No in-human data Influenced by circulating lipoprotein concentration | ||
MRI | Ultrasmall paramagnetic particles of iron oxide151–153 | Accumulates in macrophages after being phagocytosed | Coincides with active plaque disease and intense macrophage infiltration | Non-specific uptake into both M1 and M2-like macrophages Less sensitive than PET |
Various other tracers are under development and have been further summarized in Table 3. Many remain in their infancy and are being studied in animal models of atherosclerosis. For example, several tracers targeting either single or multiple chemokine receptors require further assessment in humans, although the precise and complex role of chemokine receptors and their variable expression across the macrophage polarization spectrum may make interpretation difficult.126–136 Other approaches make use of nanoparticles to utilize the phagocytic properties of macrophages.
PET/magnetic resonance imaging and nanomaterials
Most clinical nanomaterials are between 10 and 300 nm in size. Large nanoparticles preferentially accumulate in lung and liver tissue while nanoparticles <8 nm can be filtered through the kidneys. Rapid filtration of tiny nanoparticles makes this platform an attractive modality for imaging in atherosclerosis due to their rapid clearance from the blood pool and lack of uptake in structures adjacent to the vasculature (such as the lungs). 18F-Macroflor utilizes lysine cross-linked low molecular weight carboxymethyl polyglucose polymers, each containing 22 glucose units as a basis for a 5 nm nanoparticle. Due to the rapid blood pool clearance, the nanoparticle can be radiolabelled with 18F (which has a short half-life of 110 min) to facilitate the short tracer injection to scanning time required. A comprehensive series of proof-of-principle animal studies performed by Keliher et al.149 reported rapid excretion of 18F-Macroflor by the kidneys in mice, rabbits, and primates, with tracer uptake visualized in cardiac macrophages and confirmed by autoradiography and fluorescence.
Translatability to humans as well as toxicology studies are required but, overall, the safety of nanoparticles studied thus far is reassuring. Longer-term safety data will also be important as the effect of high concentrations of nanoparticles in tissue (particularly the renal tubules) remains unknown. With the development of hybrid PET and magnetic resonance imaging (MRI) platforms, ultrasmall superparamagnetic iron oxide particles can be combined with radionuclide tracers to increase sensitivity and reduce the required dose for optimal resolution given the soft-tissue resolution capabilities of MRI.151 These paramagnetic nanoparticles accumulate at sites of high macrophage concentration, including high-risk or ruptured atherosclerotic plaques.149 Continued assessment and refinement of multimodality tracers in humans remains in its infancy though is among the most promising vectors for unravelling the complexity of cardiovascular inflammation and personalizing diagnosis and therapy.
While beyond the scope of this review, technological advances in PET with total body PET (which encompasses the whole body in the field of view to improve image acquisition time, reduce radiation exposure and improve image quality), new image reconstruction techniques and hybrid tracer imaging will continue to improve sensitivity.154,155 This is particularly relevant in fields such as atherosclerosis where small lesions are prone to the partial volume effect and low target-to-background ratios and the close proximity of the blood pool can make assessment challenging.
Conclusion
While cost and access to PET limits use in routine, large-scale clinical practice, identification of high-risk individuals who would benefit from aggressive treatment to reduce cardiovascular events may lead to focused adoption, individualized cardiovascular care and an overall reduction in cost—especially considering the worldwide economic impact of cardiovascular disease over the next decade is expected to exceed US$1 trillion annually.156 New pharmacotherapies that are likely to come with considerable prices require precise strategies to quantify individual and residual inflammatory risk in a variety of clinical settings. As outlined in Figure 6, a suite of imaging modalities is available to consider with further advances in invasive imaging, total body PET, molecular targeted tracers, simultaneous contrast techniques, integrated high-resolution PET/CT and PET/MRI imaging platforms, machine learning and concurrent targeted therapy with nanoparticles in our near future. The identification of those patients who are most vulnerable to the devastating effects of uncontrolled inflammation is closer than ever.
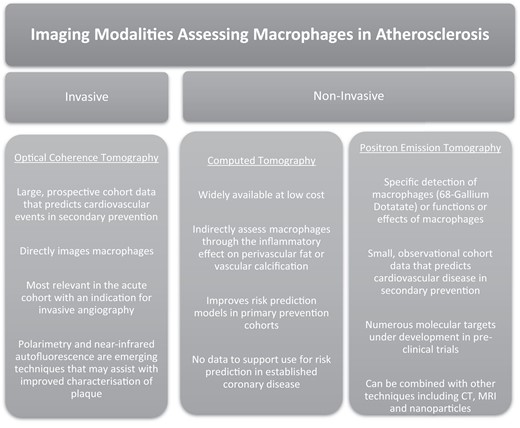
A summarized current landscape of the imaging assessment of macrophages in atherosclerosis.
Acknowledgements
We wish to thank James Down for his artistic contribution to this manuscript. All authors contributed to the final manuscript and approved the use of their respective names for publication.
Funding
This research is supported by a Western Australian Department of Health Research Registrar Fellowship, an Australian Government Research Training Program Scholarship and grants from the University of Western Australia and the Royal Perth Hospital Medical Research Foundation.
Data availability
No new data were generated or analysed in support of this research.
References
Author notes
Conflict of interest: None declared.