-
PDF
- Split View
-
Views
-
Cite
Cite
Zoe A Pritchard, Harmen P Hendriksma, Ashley L St Clair, David S Stein, Adam G Dolezal, Matthew E O’Neal, Amy L Toth, Do Viruses From Managed Honey Bees (Hymenoptera: Apidae) Endanger Wild Bees in Native Prairies?, Environmental Entomology, Volume 50, Issue 2, April 2021, Pages 455–466, https://doi.org/10.1093/ee/nvaa181
- Share Icon Share
Abstract
Populations of wild and managed pollinators are declining in North America, and causes include increases in disease pressure and decreases in flowering resources. Tallgrass prairies can provide floral resources for managed honey bees (Hymenoptera: Apidae, Apis mellifera Linnaeus) and wild bees. Honey bees kept near prairies may compete with wild bees for floral resources, and potentially transfer viral pathogens to wild bees. Measurements of these potential interactions are lacking, especially in the context of native habitat conservation. To address this, we assessed abundance and richness of wild bees in prairies with and without honey bee hives present, and the potential spillover of several honey bee viruses to bumble bees (Hymenoptera: Apidae, Bombus Latrielle). We found no indication that the presence of honey bee hives over 2 yr had a negative effect on population size of wild bee taxa, though a potential longer-term effect remains unknown. All levels of viruses quantified in bumble bees were lower than those observed in honey bees. Higher levels of deformed wing virus and Israeli acute paralysis virus were found in Bombus griseocollis DeGeer (Hymenoptera: Apidae) collected at sites with hives than those without hives. These data suggest that the presence of honey bees in tallgrass prairie could increase wild bee exposure to viruses. Additional studies on cross-species transmission of viruses are needed to inform decisions regarding the cohabitation of managed bees within habitat utilized by wild bees.
Many wild pollinators, including several bumble bee (Hymenoptera: Apidae, Bombus Latrielle) species, are in decline from multiple stressors (Goulson et al. 2003, 2008, 2015; Potts et al. 2010; Morales et al. 2013; Klein et al. 2017; Aizen et al. 2020; Soroye et al. 2020). For example, in North America, the abundance and range of Bombus occidentalis Greene, Bombus pensylvanicus DeGeer, Bombus affinis Cresson, and Bombus terricola Kirby have declined during the last 20 yr (Cameron et al. 2011). These declines are most notable in the Midwestern United States, with the state of Illinois experiencing declines in half of its bumble bee species in the last century (Grixti et al. 2009). Recently, B. affinis was listed as a critically endangered species due in part to a substantial range reduction in the continental United States, and several other once common species are listed as vulnerable to similar declines (Hatfield et al. 2015). Potential causes of bumble bee decline are global warming (Soroye et al. 2020), loss of habitat to urbanization and intensive agriculture (Colla and Packer 2008, Grixti et al. 2009), insecticides (Baron et al. 2017), and within and cross species transmission of pathogens from managed bumble bees (Williams and Osborne 2009, Cameron et al. 2011, Meeus et al. 2011, Graystock et al. 2015) and managed honey bees (Williams and Osborne 2009, McMahon et al. 2015, Alger et al. 2019).
The honey bee (Hymenoptera: Apidae, Apis mellifera Linneaus) is a managed eusocial insect, which is kept and managed in hives by beekeepers worldwide—including in areas where they are non-native, such as North and South America. Honey bee colonies are perennial superorganisms that contain up to 50,000 workers, a subset of which forage outside the hive and can interact with wild bees (Hymenoptera: Apoidea) (Seeley 1995). The vast majority of wild bees are solitary species or live in smaller, annual colonies (Michener 2007). Honey bees are floral generalists and have been documented visiting ~40,000 different plant species globally (Crane 1990). Honey bees often overlap with wild bees in the flowers they visit (Thorp et al. 1994, Thorp 1996). In the absence of honey bee colonies, bumble bees were observed using a wider variety of flowers, suggesting resource competition (Pleasants 1981). This competition may be more acute in areas with limited floral resources, and floral resource availability has been linked to bee reproductive success (Thomson and Page 2020). For example, Thomson showed reduced Bombus occidentalis reproductive success with closer proximity to honey bee hives (Thomson 2004). However, there are few experimental studies that explain how wild bee communities respond to the presence of multiple honey bee hives (but see Thomson 2004, Alger et al. 2019), especially in the context of native, nonagricultural habitats. In a review of the impacts managed bees have on wild bees, 53% of 146 studies reported negative effects of managed bees on wild bees (Mallinger et al. 2017). These emerging results suggest a need for more information on direct impacts of managed honey bees on wild bee communities and health.
In addition to resource competition, honey bee colonies could pose a risk of pathogen transmission. The majority (70%) of pathogen transmission studies have reported negative impacts of managed bees on wild bees (Mallinger et al. 2017). Honey bee colony health is threatened by a variety of pathogens (VanEngelsdorp et al. 2009, Wilfert et al. 2016, McMenamin and Flenniken 2018), including a suite of RNA viruses contributing to honey bee colony declines globally. Black queen cell virus (BQCV), deformed wing virus (DWV), Israeli acute paralysis virus (IAPV), Lake Sinai virus (LSV), and sac brood virus (SBV) are honey bee viruses that have been detected in bumble bees and other wild bee genera (Li et al. 2011, Peng et al. 2011, Fürst et al. 2014, Meeus 2014, Dolezal et al. 2016, Parmentier et al. 2016, Radzevičiūtė et al 2017, Wang et al. 2018). Because RNA viruses have high mutation rates and short generation times, they can easily adapt to new host species (Drake and Holland 1999, Li et al 2014), and transmission is likely facilitated by foraging for pollen and nectar on shared floral resources (Singh et al. 2010, Goulson et al. 2015, Alger et al. 2019).
The viruses found in honey bees are potentially harmful to bumble bees. For example, DWV infected bumble bees can suffer from wing deformations that impede their ability to fly (Genersch 2006). Bombus terrestris Linnaeus injected or fed IAPV inocula exhibited increased mortality rates (Wang et al. 2018). However, the infectivity, prevalence, and health impacts of many honey bee viruses are unknown, and the directionality of virus transmission between bee species is not always certain (McMahon et al. 2015, Galbraith et al. 2018). Based on their prevalence, BQCV and DWV seem to be linked to honey bees and ABPV and slow bee paralysis virus to bumble bees, but we still lack conclusive evidence to be sure of primary reservoir hosts (Fürst et al. 2014, McMahon et al. 2015).
Honey bees and bumble bees are both in subfamily Apinae (Bossert et al. 2019), can harbor some of the same viruses (Dolezal et al. 2016, Alger et al. 2019), and share many floral resources that are potential sites of pathogen transmission (Durrer and Schmid-Hempel 1994, Graystock et al. 2015, Alger et al. 2019). For example, IAPV transmission between honey bees and three common bumble bee species (Bombus impatiens Cresson, Bombus vagans Smith, and Bombus ternarius Say) occurred by shared flower visitations (Singh et al. 2010). With a growing body of research on pathogen transmission within Apinae, the interactions between honey bees and bumble bees deserve further exploration.
Our goal was to understand the competition and health risks associated with wild bees sharing habitat with honey bee hives. We studied this in the context of native prairie in Iowa because this habitat has been pinpointed as an area of major concern for bee health and conservation (Grixti et al. 2009, Koh et al. 2016) and honey bee viruses were previously detected in a variety of wild bee species from this region (Dolezal et al 2016). We predicted that the presence of honey bee hives would increase wild bee exposure to honey bee viruses, resulting in higher virus quantities in wild bees found foraging at prairies with apiaries. We hypothesized that managed honey bees in native habitats will negatively impact the wild bee community due to competition for resources and viral transmission. To test our hypotheses, we placed honey bee hives at restored tallgrass prairie sites in Iowa, assayed the wild bee community, and measured viral levels of DWV, IAPV, and LSV in two bumble bee species. We aimed to provide a more in-depth understanding of the potential interaction between managed honey bees and wild bees in the context of native habitat restoration.
Methods
Site Selection and Honey Bee Hive Placement
We selected seven prairie sites in the Chichaqua Bottoms Greenbelt (managed by Polk County Conservation) in Polk County (IA) during the summer of 2017. Prairie sites were restorations composed of at least 19.4 ha of prairie and were located between 1.1 and 33.71 km apart (Supp Table 1 and Supp Fig. 1 [online only]). To avoid foraging overlap of honey bee hives, and create independent sites, our minimum distance between prairie sites was based on an estimated average honey bee foraging distance in similar landscapes (Couvillon et al. 2015, Carr-Markell et al. 2020). Four of the seven prairies received honey bee hives (hive (+)), while the remaining three did not (hive (−)). The year 2017 was the first year that these sites had honey bee hives present, based on conversations with the land area manager (ALT, personal communication). At hive (−) sites, we confirmed that there were no managed hives present within 1 km by checking the Iowa voluntary hive registry (https://driftwatch.org/) and through visual inspection of the surrounding region. During the summer of 2018, we revisited the same seven prairie sites, and hive treatments (i.e., hive (+) and hive (−) locations) were assigned to the same prairies as in 2017. In 2018, we added four additional prairie sites; three at Chichaqua Bottoms and one in Story County, Iowa, for a total of 11 sites (6 hive(+) and 5 hive(−)). Pan trapping was conducted at the same six sites in 2017 and in 2018 for the wild bee community data, while hand netting was conducted at all sites (see Supp Table 1 [online] for site use details).
At hive (+) sites, we placed four honey bee hives on a wooden pallet located 20 m from the edge of the prairie (Fig. 1). We transported the hives to hive (+) prairies on 10 August 2017. In 2018, hives were moved to Lloyd Bailey, Engeldinger, and Kunze on 6 June 2018 (sites not involved in wild bee community pre/post hive-move comparisons) and to Barrer, Sandhill, and Darnell Holy Cross on 10 August 2018. Prior to placement at the prairie, the hives were kept within soybean fields (around Ames, IA). Throughout the summer, we inspected hives using methods from Dolezal et al. (2019) as follows: every other week we checked colony weights, queen presence, levels of Varroa destructor, and provided more hive space when needed. Mean monthly hive weights and mite counts are provided in Supp Tables 2 and 3 (online only).
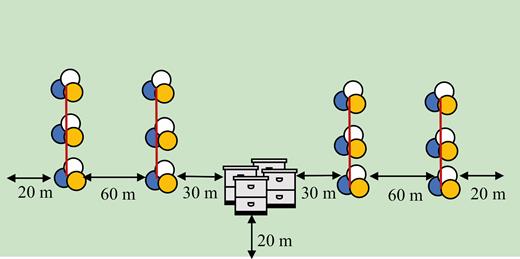
Placement of hives and trap stand transects at prairie sites in central Iowa during 2017 and 2018. Each cluster of circles represents one trap stand containing one pan trap of each color: blue, white, and yellow. Trap stands were placed in this orientation regardless of whether hives were present or not. At each pan trap collection, all bowls from each trap stand were combined resulting in one collection of bees per site per day. (For color version of this figure, see online).
All prairie sites were embedded in a larger agricultural landscape. In Iowa, approximately 85% of the landscape is in use as farmland, mainly for corn and soybean production (NASS-USDA 2017). Pesticides were not applied at our sites, but there is possibility of pesticide use in the adjacent landscape that we did not control for. Descriptions of the floral resources present at these sites are provided in another paper (Zhang et al. in review), thus they are not included here. That study gave no indication that floral resources differ systematically between honey bee and nonhoney bee sites.
Wild Bee Abundance and Taxon Richness
To assess the abundance and richness of the wild bee community at the hive (+) and hive (−) sites, we used pan traps (i.e., bee bowls) as modified by Gill and O’Neal (2015). Traps were 3.2 oz Solo brand cups painted either fluorescent blue, fluorescent yellow, or left white. This color combination attracts a robust community of bees (Droege et al. 2010). We assembled three pan traps, one of each color, on a stand at vegetation height (these are hereafter referred to as trap stands). We placed trap stands on either end of a 60-m transect, with two transects within a prairie (Fig. 1). Each transect consisted of a total of six trap stands, three on each end of the transect. We placed trap stands 10 m apart from each other and 20 m from the prairie edge. Each transect was located 60 m apart. At hive (+) prairies, each transect was placed such that the nearest end of the transect was 30 m from the hives and the farthest end was 90 m from the hives (Fig. 1). This set up resulted in a total of 36 individual pan traps placed in each prairie site.
We collected wild bees in pan traps once a week over a 7-wk period in 2017 (26 July to 20 September 2017) and over a 6-wk period in 2018 (24 July to 27 September 2018). We set pan traps between 7 and 10 am by filling pan traps with 50 ml of 0.2% soapy water solution. We collected the trap contents after 24 h. Each week we adjusted the pan traps on the stand such that traps were level with the flowering plants. At hive (+) sites, three collections occurred prior to the placement of honey bee hives (premove) and three collections took place after the hives were placed (postmove).
We processed bees by washing and drying each specimen using methods developed by Droege (2015). After processing, bees were pinned and identified utilizing keys developed by Michener et al. (1994) and Ascher and Pickering (2017). We identified bees to species, excluding those belonging to genus Lasioglossum, Curtis which were identified to subgenus. ‘Taxon richness’ is, therefore, defined as total number of species or Lasioglossum subgenera. Supplementary Table 4 (online only) lists bee taxa caught at each site through pan trapping.
Bumble bee and honey bee sampling for virus analysis
Throughout the pan trap sampling in 2017, we occasionally captured individual bumble bees, but we did not achieve capture rates that provided sample sizes suitable for viral analysis. To increase sample size, we sweep-netted additional bumble bees at each site in late summer of 2017. An observer walked within the prairie for 15 min, pausing the timer to collect bumble bees foraging on flowers. Collections occurred between 9 am to 5 pm, during peak pollinator activity on days when conditions are optimal for bee foraging activity (e.g., limited cloud cover, temperatures >19°C, low to no wind) per previously established methods (Hines and Hendrix 2005). The sweep net collection in 2017 took place across six dates from 30 September to 12 October 2017. We focused on collecting the relatively abundant male B. impatiens Cresson bees, since female bumble bee workers had become relatively rare this late in the season. At hive (+) sites, an ~100 worker honey bees were sampled from the brood nest from each hive (due to their location in the brood nest, these can be putatively assigned to the brood caring subcaste known as ‘nurse bees’). The netted bumble bees and sampled nurse honey bees were kept on ice for no more than 6 h, and then transferred to a −80°C freezer before further processing (as in Dolezal et al. 2016).
In 2018, we increased our sampling effort of bumble bees for viral analysis by conducting targeted sweep netting as in 2017 starting on 20 July 2018 and ending 30 September 2018. This allowed us to collect not only female workers, but also multiple species which were abundantly foraging during that time. Additionally, this earlier sampling time frame overlaps more with a period during which honey bees are known to actively forage in prairie landscapes (Carr-Markell et al. 2020), making it potentially more useful for detecting viral spillover. We collected B. impatiens and B. griseocollis worker (female) bumble bees at all sites approximately once per week, resulting in six sample dates. We were especially interested in worker bumble bees because they forage for both pollen and nectar, unlike male bumble bees, and would therefore be more likely to be exposed to honey bee viruses transferred in the environment via floral resource sharing.
In 2018, we collected nurse honey bees from the brood nest of hives at hive (+) sites, as in 2017. We additionally captured honey bee foragers from flowers at hive (+) sites by sweep-netting—since they may potentially transfer viruses from their hives to other bee species. Collected honey and bumble bees were stored as described above. No threatened or endangered species were collected. Bumble bee species caught at each site via netting are listed in Supp Table 5 (online only).
Virus Extraction and Quantification
In 2017, we measured viral titers of DWV in male B. impatiens (n = 53) and nurse honey bees (n = 21). In 2018, we measured DWV, LSV, and IAPV in female B. griseocollis (n = 22), female B. impatiens (n = 16), as well as nurse honey bees (n = 20) and foraging honey bees (n = 16). To extract RNA from all bee samples, we crushed an individual whole bee body in Trizol, removed DNA contamination with a DNAse treatment, and standardized to a constant RNA concentration. We detected viruses using real-time qRT-PCR methods (Sybr Green with a BioRad CFX-384 real-time quantitative PCR machine) using previously published primers for deformed wing virus (DWV), Israeli acute paralysis virus (IAPV), and Lake Sinai virus (LSV) (see Supp Table 6 [online only]; Carrillo-Tripp et al. 2016, Dolezal et al. 2016). Following the methods of Carrillo-Tripp et al. 2016, we estimated virus titers (genome equivalents/100 ng RNA) using a standard curve. The standard curve was based on a known quantity of viral fragment RNA, previously generated based on RT–PCR and cloning of each virus (Carrillo-Tripp et. al. 2016). We then serially diluted the RNA (1:10) with molecular grade water to create a range of viral titers. This standard curve allowed us to calculate genome equivalents (from the quantity of viral fragment RNA and viral reference sequence for each virus) for each sample for each target virus, which were calculated on the Bio-Rad CFX software. On each qPCR plate, we ran two replicates of the standard curve, as well as molecular grade water samples as negative controls alongside the samples. The qPCR program used 45 cycles. We examined melting curves of qPCR products to ensure that target products were amplified. Any curves that amplified nontarget products were removed from analysis, as these could be due to primer dimerization or other qPCR artifacts. We examined cycle threshold (Ct) values of the samples and report these values for each qPCR run in Supp Table 7 (online only). Although some samples had high Ct values, we included them as they showed target product amplification, and prior research corroborates the validity of including data with high Ct values (McCall et al. 2014). In 2017, we tested male B. impatiens and hive-collected nurse honey bees for quantities of DWV. In 2018, we tested female B. impatiens, female B. griseocollis, honey bee foragers, and hive-collected nurse honey bees for quantities of DWV, IAPV, and LSV.
Previous studies set a conservative ‘virus detection threshold’ for honey bees based on the technical limits of detection for each primer; these limits were 4.92E + 02 viral genome equivalents for LSV and IAPV, and 4.92E + 03 viral genome equivalents for DWV (Carrillo-Tripp et al. 2016, Dolezal et al. 2016). We used the same primers and standard curve procedure as Dolezal et al. (2016) to determine levels of viral genome equivalents in our samples. According to levels detected in previous papers, virus titers in the current study were generally very low, with many samples below the previously set threshold, especially in bumble bee samples of IAPV and DWV.
Although viral titers were generally low, we were interested to further explore the full dataset, including samples below the previously set conservative honey bee threshold, but still showing evidence of amplification. We did this for two reasons: first, there was still some variability among the titers and we wanted to investigate whether this variation was meaningful (i.e., variation between site types). Second, we wanted to explore these samples to include all data to address possible low-level exposure to viruses that may have the potential to still be biologically relevant. To do this, we calculated titers based off of the curve for all samples that amplified, including samples that were below the previously published threshold.
Statistical Analysis
To analyze the abundance and taxon richness of wild bees, we combined pan traps from both transects within a site at each date, resulting in one measure of bee abundance and taxon richness per site per date. We defined abundance as the number of individual specimens captured per site per date, and taxon richness as number of bee species, or Lasioglossum subgenera, captured per site per date (as in Stein et al. 2020). We removed counts of honey bees from pan traps from the wild bee analysis because specimens caught were likely from our hive treatments (St. Clair et al. 2020a). However, we separately compared honey bee abundance between site types to validate whether we achieved the desired effect of adding hives to the selected prairies.
We checked for normality of each abundance or taxon richness response variable using PROC UNIVARIATE (Supp Table 8 [online only]). If response variables did not meet the assumptions of normality, we used a logarithmic transformation to improve normality and ran the analysis with the log-transformed data. Some log-transformed responses were still not normal (P < 0.05), so the data distributions were visualized. If distributions were improved, the log-transformed data were used in the ANOVA test, otherwise, the untransformed data were used. We compared overall wild bee abundance and taxon richness between hive (+) sites and hive (−) sites using a mixed model analysis of variance (ANOVA) in SAS 9.4 (PROC GLIMMIX). PROC GLIMMIX was used as it is capable of working with non-normal data. The statistical model used included main effects of hive presence and date, with location as a random variable, and a separate model was run for each year of the study. To compare the bee community between hive (+) and hive (−) sites, and between premove and postmove dates, we conducted post hoc comparisons of least squared means using a Tukey adjustment within the model.
As we were interested in potential competitive effects of honey bees on closely related wild bees, we conducted separate analyses of abundance and taxon richness using the same SAS model as above for the subset of wild bees in family Apidae as well as family Halictidae. We did not conduct separate analyses for bees in families Colletidae or Megachilidae because of insufficient sample sizes for bees within those families.
To analyze viral quantities in bumble bees and honey bees, we averaged the viral estimated genome equivalent (titer) measurement between the two qPCR replicates for each individual bee. In order to be able to normalize the data through log transformation, we calculated log10 (virus titer+1), which allowed us to retain zero values in the dataset. Zero values were defined as samples producing no amplification in the qPCR. We used RStudio (RStudio Team 2019) packages ‘nlme’ (Pinheiro et al. 2020) and ‘yarrr’ (Phillips 2017) to analyze viral data with linear mixed models and to produce pirate plots, showing raw data (points), descriptive statistics (lines), and inferential statistics (intervals) (Figs. 6 and 7). We compared viral levels between honey bees and bumble bees, and within bumble bees at the two site types using ANOVA and Tukey post hoc analyses.
Results
Honey Bee Abundance
Pan traps can be used to provide rough assessments of honey bee abundance (St. Clair et al. 2020a); thus, we used this method to validate that hive (+) sites had increased honey bee activity density compared to hive (−) sites. Over the two years we collected a total of 69 honey bees in pan traps (15 in 2017 and 54 in 2018). In both years, we observed an order of magnitude more honey bees in pan traps at hive (+) sites compared to hive (−) sites (13 vs 2 in 2017; 50 vs 4 in 2018, respectively). During 2017, we observed significantly more honey bees in traps in hive (+) sites compared to hive (−) (F1,38 = 6.53; P = 0.02; Fig. 2A). Collections varied significantly by date (i.e., pre- or postmove), with more bees captured postmove (F1,38 = 5.12; P = 0.03; Fig. 2A) but no interactions of hive presence and hive move (F1,38 = 1.93; P = 0.17; Fig. 2A). Within the hive (+) sites, there was significantly higher abundance of honey bees caught postmove compared to premove (T38 = 2.58; P = 0.01; Fig. 2A), whereas there was no significant difference between dates in hive (−) sites (T38 = 0.62; P = 0.54; Fig. 2A). At postmove dates, there were significantly more honey bees captured at hive (+) sites than hive (−) sites (T38 = 3.01; P = 0.01; Fig. 2A) while there was no difference between site types at premove dates (T38 = 0.77; P = 0.44; Fig. 2A). We observed the same pattern in 2018, i.e., a significant effect of hive presence (F1,38 = 7.97; P = 0.01; Fig. 2B) with more honey bees captured in hive (+) sites, a significant effect of date (F1,38 = 6.34; P = 0.02; Fig. 2B) with more bees captured posthive move, and a significant interaction of hive* date (F1,38 = 5.4; P = 0.03; Fig. 2B). Within the hive presence treatments, we observed significantly more honey bees at hive (+) sites postmove compared to premove (T38 = 3.52; P < 0.01; Fig. 2B), whereas there was not a significant difference between pre- and postmove for hive (−) sites (T38= 0.13; P = 0.89; Fig. 2B). When comparing between hive presence treatments we observed no significant difference between hive (+) and hive (-) premove (T38 = 0.32; P = 0.75; Fig. 2B), but postmove, there were significantly more honey bees captured in hive (+) sites (T38= 4.05; P < 0.01; Fig. 2B).
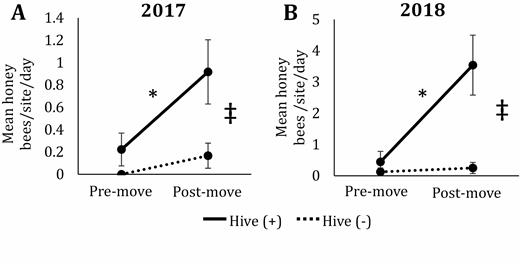
Mean abundance of honey bees captured in pan traps per site per date in 2017 (A) and 2018 (B) in prairies with (solid line) and without (dotted line) the presence of honey bee hives in central Iowa. Premove indicates collections made before hives were moved onto hive (+) sites and includes three dates during 2017 and 2018. Postmove indicates collections made after hives were moved onto hive (+) sites and includes three dates in 2017 and four dates in 2018. Results based on ANOVA and Tukey post hoc comparisons. Error bars represent one standard error of the mean. Asterisks signify significant differences (P < 0.05) between pre- and postmove within a hive presence treatment and the double cross indicates significant differences between hive presence treatments at pre- and postmove time points.
Wild Bee Abundance and Taxon Richness
In 2017, we caught a total of 974 wild bees in pan traps, representing at least 50 species. We trapped 565 specimens at sites with honey bee hives, and 409 at sites without hives. Of these, we identified 635 Halictidae (16 species or Lasioglossum subgenera), 272 Apidae (22 species), 47 Colletidae (3 species), and 20 Megachilidae (9 species). We identified 86 Bombus specimens representing 8 different species. In 2018, we caught a total of 149 wild bees in pan traps, representing at least 22 species. We trapped 79 specimens at hive (+) sites, and 70 from at (−) sites where hives were absent. Of these wild bees, we identified 59 Halictidae (8 species or Lasioglossum subgenera), 88 Apidae (13 species), and 2 Megachilidae (1 species). We identified 37 specimens of Bombus representing 4 species. Honey bees were excluded from all analyses of wild bee abundance and taxon richness.
In 2017, the overall wild bee abundance varied significantly with both hive presence and date (premove or postmove) (F1,38 = 10.31; P < 0.01 for hive presence, F1,38 = 8.20; P = 0.01 for hive date; Fig. 3A), with no significant interaction (F1,38 = 0.64; P = 0.43; Fig. 3A). Postmove dates had significantly lower abundance than premove dates in hive (−) sites, and slightly lower abundance during postmove dates compared to postmove dates in hive (+) sites (T38 = 2.59; P = 0.01 for hive (−); T38 = 1.46; P = 0.15 for hive (+); Fig. 3A). Surprisingly, wild bee abundance at postmove dates was higher at hive (+) sites, but there was no significant difference for premove dates (T38 = 3.06; P < 0.01 for postmove dates; T38 = 1.59; P = 0.12 for premove dates; Fig. 3A). Taxon richness of the wild bee community significantly varied by hive presence, but not by date nor the interaction of date and hive presence in 2017 (F1,4.17 = 12.53; P = 0.02 for hive; F1,34 = 1.49; P = 0.23 for date; F1,34 = 0.75; P = 0.39 for interaction; Fig. 3B). Again, contrary to predictions, we observed a trend of higher taxon richness of bees collected in hive (+) sites compared to hive (−) sites (T17.77 = 1.78; P = 0.09 for premove dates; T11.35 = 3.37; P < 0.01 for postmove dates; Fig. 3B).
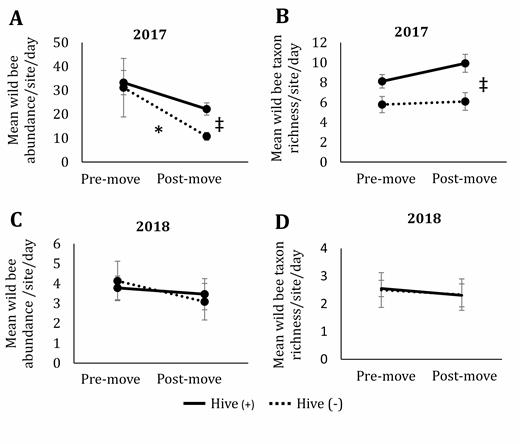
Mean abundance of wild bees collected per site per date in 2017 (A) and 2018 (B) as well as mean taxon richness of wild bees collected in 2017 (C) and 2018 (D) in prairies with (solid line) and without (dotted line) the presence of honey bee hives in central Iowa. Premove indicates collections made before hives were moved onto hive (+) sites and includes three dates during 2017 and 2018. Postmove indicates collections made after hives were moved onto hive (+) sites and includes three dates in 2017 and four dates in 2018. Results based on ANOVA and Tukey post hoc comparisons. Error bars represent one standard error of the mean. Asterisks signify significant differences (P < 0.05) between pre- and postmove within a hive presence treatment and the double cross indicates significant differences between hive presence treatments at pre- and postmove time points.
In 2018, wild bee abundance did not vary significantly by hive presence, date, nor an interaction between the two (F1,3.99 = 0.00; P = 0.98 for hive; F1,34.06 = 0.77; P = 0.39 for date; F1,34.06 = 0.22; P = 0.64 for interaction; Fig. 3C). Taxon richness of all wild bees in 2018 did not vary significantly by hive presence, date, nor their interaction (F1,38 = 0.00; P = 0.98 for hive; F1,38 = 0.17; P = 0.68 for date; F1,38 = 0.01; P = 0.94 for interaction; Fig. 3D).
Apidae Only
Apidae abundance in 2017 did not vary significantly by hive presence, date, nor their interaction (F1,4.08 = 4.96; P = 0.09 for hive presence; F1,34 = 0.04; P = 0.85 for date; F1,34 = 0.78; P = 0.38 for interaction). At postmove dates, Apidae abundance was higher at hive (+) sites compared to hive (−; T7.10 = 2.45; P = 0.04; Fig. 4A), which is in line with the results for all bees mentioned above. Apidae taxon richness in 2017 (Fig. 4B) varied significantly by hive presence (F1,38 = 13.35; P < 0.01) but not by date or the interaction (F1,38 = 1.77; P = 0.19 and F1,38 = 0.90; P = 0.35, resp.). Hive (+) sites showed higher species richness at both pre- and postmove dates, but only the postmove dates showed a significant difference between site types (T38 = 3.51; P < 0.01 at postmove dates; T38 = 1.79; P = 0.08 at premove dates; Fig. 4B).
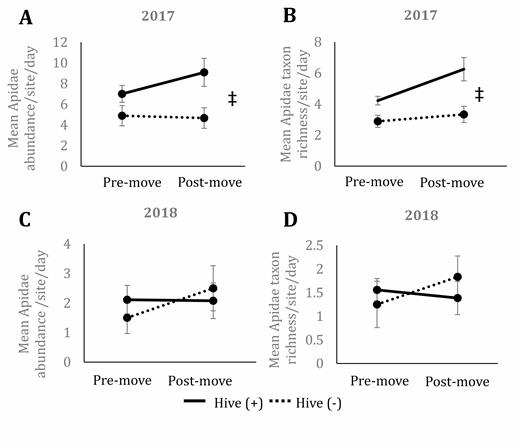
Mean abundance of Apidae collected per site per date in 2017 (A) and 2018 (B) as well as mean species richness of Apidae collected in 2017 (C) and 2018 (D) in prairies with (solid line) and without (dotted line) the presence of honey bee hives in central Iowa. Premove indicates collections made before hives were moved onto hive (+) sites and includes three dates during 2017 and 2018. Postmove indicates collections made after hives were moved onto hive (+) sites and includes three dates in 2017 and four dates in 2018. Results based on ANOVA and Tukey post hoc comparisons. Error bars represent one standard error of the mean. Asterisks signify significant differences (P < 0.05) between premove and postmove within a hive presence treatment and the double cross indicates significant differences between hive presence treatments at premove and postmove time points.
In 2018, Apidae abundance did not vary significantly by hive presence, date, or the interaction between the two (F1,38 = 0.18; P = 0.67 for hive; F1,38 = 0.07; P = 0.7921 for date; F1,38 = 1.22; P = 0.277 for interaction; Fig. 4C). Apidae taxon richness did not vary significantly by hive presence, date, nor the interaction (F1,38 = 0.06; P = 0.81 for hive; F1,38 = 0.06; P = 0.81 for date; F1,38 = 1.71; P = 0.20 for interaction; Fig. 4D).
Halictidae Only
Halictidae abundance in 2017 varied significantly by date, but not by hive presence or the interaction (F1,4.06 = 2.57; P = 0.18 for hive presence; F1,34 = 24.13; P < 0.01 for date; F1,34 = 0.22; P = 0.64 for interaction; Fig. 5A). There was strong evidence for more Halictidae at premove dates compared to postmove dates in both hive (+) and hive (-) sites (T34 = 3.14; P < 0.01 at hive (+); T34 = 3.80; P < 0.01 at hive (−) sites; Fig. 5A). There was no significant difference between site types at either premove or postmove dates. Halictidae taxon richness did not vary significantly by hive, date, nor interaction in the model, but trended towards higher taxon richness at hive (+) sites and at premove dates in 2017 (F1,4.1 = 3.63; P = 0.13 for hive presence; F1,34 = 3.92; P = 0.06 for date; F1,34 = 0.07; P = 0.80 for interaction; Fig. 5B).
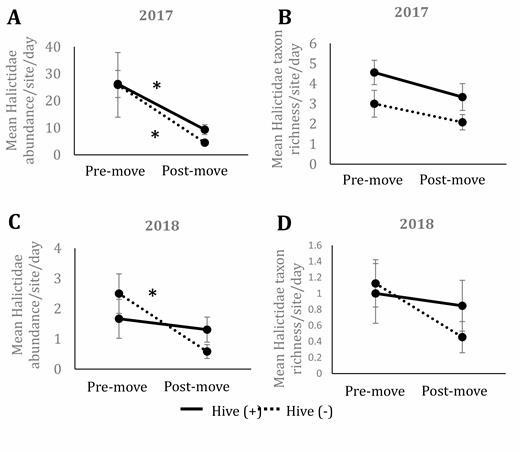
Mean abundance of Halictidae collected per site per date in 2017 (A) and 2018 (B) as well as mean taxon richness of Halictidae collected in 2017 (C) and 2018 (D) in prairies with (solid line) and without (dotted line) the presence of honey bee hives in central Iowa. Premove indicates collections made before hives were moved onto hive (+) sites and includes three dates during 2017 and 2018. Postmove indicates collections made after hives were moved onto hive (+) sites and includes three dates in 2017 and four dates in 2018. Results based on ANOVA and Tukey post hoc comparisons. Error bars represent one standard error of the mean. Asterisks signify significant differences (P < 0.05) between pre- and postmove within a hive presence treatment and the double cross indicates significant differences between hive presence treatments at pre- and postmove time points.
In 2018, Halictidae abundance varied significantly by date, but not by hive presence or the interaction between date and hive presence (F1,34.06 = 6.84; P = 0.01 for date; F1,4.01 = 0.02; P = 0.89 for hive presence; F34.06 = 3.15; P = 0.09 for the interaction). At hive (−) sites, the premove dates had significantly higher abundance than postmove dates (T34.13 = 3.03; P < 0.01; Fig. 5C), but there was not a significant difference at hive (+) sites. Halictidae taxon richness in 2018 did not vary significantly with hive presence, date, nor the interaction (Fig. 5D).
Viral Prevalence and Titers
Prevalence
Consistent with previous research showing that wild bees can harbor honey bee viruses (Dolezal et al. 2016), we did detect all three viruses in bumble bees. Viral prevalence, or the number of bumble bees with detectable viral levels above a previously established honey bee viral presence threshold, was generally very low. No bumble bees had IAPV over the prior threshold. DWV was detected over threshold in 4% of B. impatiens male bumble bees in 2017, and 0% of female B. impatiens and B. griseocollis collected in 2018. However, LSV was found at higher prevalence, detected over threshold in 100% of B. impatiens and 100% B. griseocollis females. Because we found mostly low titers in bumble bees, we next compared threshold-free viral titers between honey bees and bumble bees, and then examined differences in titers between bumble bees at the two site types.
Viral Titers
Both forager honey bees and hive-collected nurse honey bees had significantly higher DWV levels than B. griseocollis (P < 0.01; P < 0.01, respectively, Fig. 6A; Supp Table 9 [online only]) and B. impatiens (P < 0.01; P < 0.01, respectively, Fig. 6A; Supp Table 9 [online only]) in 2018. Nurse honey bees had higher DWV levels than foraging honey bees (P < 0.01; Fig. 6A; Supp Table 9 [online only]). For LSV, there were higher viral levels in hive honey bees than in both B. griseocollis and B. impatiens (P = 0.01; P = 0.03 respectively; Fig. 6B; Supp Table 9 [online only]). For LSV, there was no difference between hive honey bees and forager honey bees (P = 0.14; Fig. 6B; Supp Table 9 [online only]). IAPV did not show any significant differences between bee categories (Fig. 6C).
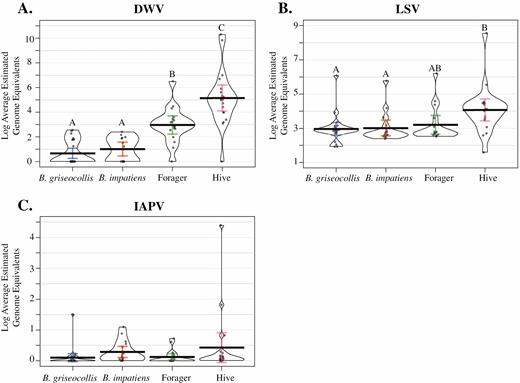
Log average estimated genome equivalents for the DWV (A), LSV (B), and IAPV (C) in 2018, compared between the two bumble bee species, honey bee foragers, and nurse honey bees from the hive. Letters denote estimated genome equivalents significantly different from other bee categories (P < 0.05). In IAPV, no category was significantly different from another. Significance determined with ANOVA and Tukey post hoc analysis. Full statistical report in Supp Table 9 (online only).
We found strong evidence that IAPV titers were higher in female B. griseocollis at hive (+) sites than hive (-) sites, and moderate evidence that DWV quantities in B. griseocollis were higher at hive (+) sites (P < 0.01, P = 0.07 respectively, Figure 7A and B; Supp Table 10 [online only]). Male B. impatiens showed some evidence for higher DWV levels at hive (+) sites compared with hive (−) sites (P = 0.14, Fig. 7C, Supp Table 10 [online only]).
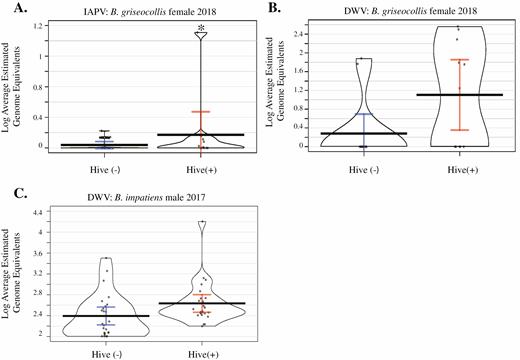
Viral levels of IAPV in Bombus griseocollis females (A), DWV in B. griseocollis females (B), and DWV in Bombus impatiens males (C) in bumble bees caught at hive (+) sites compared to those from hive (−) sites. IAPV and DWV quantities were higher in B. griseocollis at hive(+) sites than hive(−) sites (P < 0.001, P = 0.071, respectively, ANOVA), and DWV quantities were higher in male B. impatiens at hive(+) sites (P = 0.138, ANOVA). Full statistical report in Supp Table 10 (online only).
Discussion
This study presents data on the potential effects of honey bee hives on wild bees in the context of a restored native prairie habitat in the Midwestern United States. Many native plants found in prairies are highly attractive to both honey bees and wild bees (Tuell et al. 2008). Native prairies provide habitat for wild bees, including bumble bees, and wild bee abundance responds positively to availability of floral resources in prairie ecosystems (Hines and Hendrix 2005). In the state of Iowa, native prairie remnants have higher abundance and diversity of floral resources than ruderal grasslands and are an important wild bee habitat (Kwaiser and Hendrix 2008). Since both wild bees and managed honey bees utilize prairie habitats, there is potential for resource competition and the potential displacement of wild bees, as well as the potential for transmission of viruses between bee species.
We used an experimental approach to examine this question, by adding managed honey bee hives to multiple restored prairie sites in Iowa. We first verified that our experiment altered the amount of honey bees present, noting more honey bees caught in pan traps from hive (+) sites compared to hive (−) sites, especially after hives were delivered to the prairies (i.e., posthive move, Fig. 2). This suggests our treatment structure was effective in increasing honey bee abundance and out of hive flight activity at sites where hives were placed. However, we note that occasionally at hive (−) sites we collected honey bees in pan traps, particularly in the postmove period, suggesting that honey bee foraging/flight activity in the environment may increase during this part of the season. Indeed, honey bee hives experience a dearth of forage in central Iowa during this postmove period (Dolezal et al. 2019), and this has been previously associated with a spike in honey bees being captured in pan traps (St. Clair et al. 2020a). Several species of plants found in prairies bloom in the late summer and early fall, making this an attractive source of forage for honey bees (Zhang et al. in review). Alternatively, it is possible that the honey bees captured at sites without hives may have been foragers from our own hives kept at nearby sites for this project, or from other unidentified managed or feral hives in the surrounding landscape. Regardless, even though our hive (−) sites showed some presence of honey bee foraging, our hive treatment clearly increased honey bee presence at hive (+) sites.
Beekeeping has been shown to reduce wild pollinator diversity and alter plant-pollinator interactions in some pollination networks (Valido et al. 2019). For wild bee abundance and taxon richness, we predicted that stress caused by competition and pathogens from honey bees would correspond with overall lower abundance and richness of wild bees. We further predicted this would be most dramatic for closely related species in the family Apidae and less so for more taxonomically distant species like those in the family Halictidae. Contrary to our predictions, 2017 data revealed trends of more abundant and taxon-rich communities of wild bees in hive (+) sites (Fig. 3A and B), as well as more abundant and taxon-rich communities in the Apidae and Halictidae (Figs. 4 and 5A and B). However, we did not observe these trends in 2018 for the overall community nor family communities. We propose several possible explanations for these unexpected results.
1) Pan traps have limitations as a tool to understand the abundance of native bee populations (Portman et al. 2020, St Clair et al. 2020b). These traps are effective at catching family Halictidae (Roulston et al. 2007, Grundel et al. 2011) but may be less effective at catching members of the Apidae, like bumble bees and honey bees (Toler et al. 2005, Grundel et al. 2011), at least in some regions, with the Midwest United States included (Gill and O’Neal 2015, Wheelock and O’Neal 2016, St. Clair et al. 2020b). Pan traps are estimated to capture only about half of all the species found in an area and are suggested to be paired with netting (Grundel et al. 2011) or reconsidered for their use in bee population studies (Portman et al. 2020). The relative abundance of different species caught in pan traps is not a completely accurate picture of the bee community. Pan traps measure activity-density of bees in an area (St. Clair et al. 2020a and b), not the full range of abundance and taxon richness present.
2) Increased Apidae abundance in pan traps could signify a dearth of resources in the surrounding habitat (St. Clair et al. 2020b). Pan traps are presumed to be attractive because of their appearance as a potential floral resource. If the surrounding prairie did not have adequate resources, or resources were utilized by the abundant managed honey bees, foraging wild bees might be more likely to be attracted to pan traps, being unsuccessful in finding real flowers to forage upon. This may be especially pronounced for bumble bees, as they are large-bodied and some of the least likely bees to be caught in pan traps, making their relative abundance in pan traps more surprising. Several researchers predict a possible inverse relationship between effectiveness of pan traps and flower abundance (Roulston et al. 2007, Baum et al. 2011).
3) Additionally, it is also possible that, due to unintended random variation in site quality, the sites with hives present had better floral resources than the sites without hives, leading to more abundant bees at those sites in 2017 collections. Variations in floral resources across sites and years may also help to explain why these trends were present in 2017 and absent in 2018.
4) One potential explanation for the lack of evidence of a competitive effect in our study could be that resources were not limiting in the landscape. We did not measure nectar and pollen availability at our sites, and therefore cannot discuss if there were limiting resources in the environment. Additionally, we do not have direct observations of any honey bees competing with wild bees. Such data would be useful in future studies that address wild and managed bee competition.
5) Last, the impact of honey bees on a wild bee community may not be seen in the first 2 yr of study, and more years of sampling may be needed to see the effects. Further years would be especially interesting, as we collected far fewer wild bees in 2018 compared with 2017 and do not yet know if that will be an ongoing trend, or just an anomaly. Since bees obtain nutrition as larvae and overwinter as pupae, the provisions available in one summer, when the female bees are foraging, determine the nutrition of the next-generation of bees emerging. Increased competition and pressure on wild bees could result in less provisioning for the next generation, resulting in effects on their populations not seen until future years.
Although our wild bee data did not provide evidence of a negative impact of honey bees on wild bee communities, it is still possible for honey bees to transmit viral pathogens to wild bees without having immediate negative effects on the community. To address the possibility of viral transmission from managed honey bee hives to wild bees, we used bumble bees as a focal genus to delve deeper into the possible impact of honey bees on wild bees. Flowering plants in a prairie are a potential location for the transfer of parasites and pathogens, including viruses. Viruses could be transferred through shared floral resources when bumble bees and honey bees coexist in an ecosystem (Singh et al. 2010). Flowers have been highlighted as a potential medium for pollinator pathogen transfer by previous research (Durrer and Schmid-Hempel 1994, Graystock 2015). Experimental studies have also demonstrated potential for bumble bee and honey bee parasites to be transferred onto flowers by bee host species, then spread between flowers by other pollinator species (Graystock et al. 2015).
Virus transmission is shaped by many factors, not all of which were explored in this study. Plant-pollinator networks can shape pathogen dynamics in a community: for example, landscape simplification can reduce bee pathogen loads in a common bumble bee species due to dietary shifts (Figueroa et al. 2020). Bee parasite loads on flowers and in bees are also known to fluctuate across a season depending on bee diversity and bee and flower abundance (Graystock et al. 2020), presenting an interesting potential future direction for bee pathogen work. Our data corroborate the evidence that co-visitations of flowers in native habitat could help vector viruses amongst bee species, but we do not have direct transmission evidence from flowers. Research has demonstrated that BQCV and DWV are more prevalent in bumble bees caught in sites with honey bee hives present, compared to sites further from hives (Alger et al. 2019). Our results show that, in some instances, bumble bees at sites where hives were placed had higher viral quantities than when no hives were present (Fig. 7). However, viral levels in bumble bees were very low overall, and many were below the previously established limit of detection. The levels we detected in these individuals may thus only represent exposure to honey bee viruses, not necessarily sickness or infection (overt virus infection symptoms were not apparent in any collected bees; ZAP, personal observation). It is still unclear if and how the bumble bee population would be impacted by these low viral levels. More research in this area is needed to determine whether viral exposure may be a concern for wild bee conservation when placing honey bee hives in native habitat.
This study did not sequence the qPCR products of the virus amplified from wild and managed bees. Such sequencing data can reveal if the variants of viruses are shared among cohabitants. For example, evidence of viral communities being shared between managed and wild bees was observed by tracking DWV variants in populations of Bombus terrestris and managed honey bees (Fürst et al. 2014). As the number of viruses tracked in wild bee populations continues to increase, future work tracking variants in DWV and other pathogens will be useful for determining virus community sharing and directional pathogen transfer.
Honey bee viruses are often associated with mites, but research indicates that oral transmission is also possible. For example, bumble bees have been observed with the characteristic crumpled wings which are indicative of a DWV infection but showed no presence of the V. destructor mites, suggesting possible oral transmission of DWV (Genersch et al. 2006). This also suggests that DWV infection causes crumpled wings in bumble bees as well as honey bees. In our study, none of our collected bumble bees were observed to have crumpled wings, even though 100% of the 2017 bumble bees collected and 41.02% of those in 2018 had levels greater than zero of the virus present. It is possible that bees with crumpled wings are less likely to be caught by researchers because they may be too malformed to fly from the nest and would therefore create a bias in collecting more healthy bees. The fact that we observed very low-level viral quantities, and a lack of obvious symptoms such as crumpled wings, could indicate that bees came into contact with the virus as adults (as deformed wings stem from infection during development), which would align with the hive placement late in the season. It would be valuable to track viral infections and symptoms over a longer time scale; even though viruses were detected at low levels in our samples, legacy effects are certainly possible in future seasons or generations. However, we lack information on how virus levels relate to symptoms and health in bumble bees, and this area deserves further study (but see Wang et al. 2018).
While this study adds to our understanding of the interaction between wild bees and honey bees, it also raises many new questions that deserve future research. Our data suggest that bumble bee exposure to honey bee viruses is likely to be higher for some viruses (like DWV) in sites with honey bee hives present. However, this was not true for all viruses and many questions remain. Why are there different patterns of exposure for different viruses? What is the nature of interactions between wild and managed bees, and when and how are viruses spread in the bee community? If male bumble bees are infected, like the male B. impatiens sampled in 2017, could they transmit viruses to the queen during mating? Could queens transfer viruses to the next year’s brood, and how might that impact the colony health? These differences between viral prevalence have been observed in past studies and could signal different susceptibilities for some wild bee species (Singh et al. 2010, McMahon et al. 2015, Dolezal et al. 2016). It is also uncertain if our study fully represented the virus levels present in the bee community. There is the possibility that the bumble bees most affected by honey bee viruses were not caught in our sampling because they were unable to fly and not seen by samplers. Future studies should aim to study bumble bees with confirmed, high level and symptomatic infections in order to better understand the range and effects of the level of virus present. New technology will also be key in future studies; Galbraith et al. (2018), for example, utilized metagenomics to screen honey bees and other bee species for many viruses simultaneously.
Understanding how managed honey bees interact with wild bees, like bumble bees, is important to the study of pollinator health, as there is concern that shared diseases could be contributing to native bee declines. If keeping honey bees on prairies leads to higher disease levels in wild bees, land managers interested in bee conservation might choose other locations for raising honey bee hives. The use of native flower plantings and habitat have been proposed as a strategy to provide forage for both wild and managed pollinators (Vilsack et al. 2016, Dolezal et al. 2019), but given shared flower usage, it is unclear whether doing so in areas in which pollinators are actively managed will also increase pathogen pressure on native species. This study provides an important early step in understanding managed and wild bee pathogen interactions in native prairie habitat; however, additional studies are still needed for a broader understanding of pollinator health in the Great Plains in order to best inform land management decisions that can impact bee health and conservation.
Acknowledgments
This work was supported by USDA NIFA 2017-68004-26326 (to ALT, AGD, MEO) and the Iowa State University Honors Program Grant (to ZAP). We would like to thank Cindy Hildebrand, and Doug Sheeley, Natural Resources Supervisor at Chichaqua Bottoms Greenbelt, for access to sites. Maria Cline helped with collection of bumble bees. Amy Geffre assisted in the qPCR process. Fernando Miguez provided statistical guidance, and Jing Zhang and Greta Linse provided statistical guidance and code for viral analysis in RStudio. Statistical methods and analysis on viral data reported in this publication were suggested and reviewed by Statistical Consulting and Research Services at Montana State University that is supported by Institutional Development Awards (IDeA) from the National Institute of General Medical Sciences of the National Institutes of Health under Awards P20GM103474, U54GM115371, and 5P20GM104417. The content is solely the responsibility of the authors and does not necessarily represent the official views of the National Institutes of Health.