-
PDF
- Split View
-
Views
-
Cite
Cite
Eric H Clifton, Ann E Hajek, Nina E Jenkins, Richard T Roush, John P Rost, David J Biddinger, Applications of Beauveria bassiana (Hypocreales: Cordycipitaceae) to Control Populations of Spotted Lanternfly (Hemiptera: Fulgoridae), in Semi-Natural Landscapes and on Grapevines, Environmental Entomology, Volume 49, Issue 4, August 2020, Pages 854–864, https://doi.org/10.1093/ee/nvaa064
- Share Icon Share
Abstract
Spotted lanternfly, Lycorma delicatula (White), is an invasive Asian insect that was initially found in Berks County, Pennsylvania, in 2014. As of early 2020, this pest had been found in five more eastern states and it is expected to continue to expand its geographical range. Lycorma delicatula is highly polyphagous but seems to prefer tree-of-heaven, Ailanthus altissima. However, grape growers in Pennsylvania have reported significant damage and loss of vines caused by L. delicatula adults. In fall 2018, two fungal entomopathogens (Beauveria bassiana and Batkoa major) drove localized collapses in L. delicatula populations in Berks County, Pennsylvania. In 2019, we tested applications of a commercialized mycoinsecticide based on B. bassiana strain GHA on L. delicatula populations in a public park in southeastern Pennsylvania. A single application of B. bassiana reduced fourth instar nymphs by 48% after 14 d. Applications of B. bassiana to L. delicatula adults in the same park resulted in 43% mortality after 14 d. Beauveria bassiana spores remained viable on foliage for 5–7 d after spraying. We also conducted semi-field bioassays with B. bassiana GHA (formulated as BoteGHA and Aprehend) and another mycoinsecticide containing Isaria fumosorosea Apopka Strain 97 against L. delicatula adults feeding on potted grapes. All the mycoinsecticides killed ≥90% of adults after 9 d using direct applications. Aprehend killed 99% of adults after 9 d with exposure to residues on sprayed grapes. These data show that fungal entomopathogens can help to suppress populations of L. delicatula in agroecosystems and natural areas.
Spotted lanternfly, Lycorma delicatula (White), is an invasive Asian fulgorid that was first discovered in North America in southeastern Pennsylvania in 2014 (Dara et al. 2015). This large planthopper is native to China, Taiwan, and Vietnam (Liu and Mottern 2017). It spread to South Korea in 2004 (Han et al. 2008, Park et al. 2009) and was found in Japan in 2009 (Kim et al. 2013). Lycorma delicatula will feed on >73 different plant species in 32 families, including trees, vines, and other woody and herbaceous plants (Liu and Hartlieb 2019). This species is univoltine and the diversity of plant species fed upon is broader for nymphs earlier in the season and narrows as L. delicatula age. One of the most preferred hosts, especially prior to oviposition, is the invasive tree-of-heaven, Ailanthus altissima (Mill.) Swingle, which contains cytotoxic alkaloids (Dara et al. 2015). Lycorma delicatula feed through the plant epidermis or bark on the phloem tissues and this can cause oozing wounds and can kill branches when populations are dense (Liu 2019). In later instars, populations causing damage are often aggregated and very abundant (Urban 2020). In addition, the copious amounts of honeydew produced by L. delicatula feeding can attract vespid wasps and ants, and also leads to the growth of sooty mold (Liu and Mottern 2017). Lycorma delicatula has been reported causing economic damage in vineyards in South Korea and, similarly, in southeastern Pennsylvania, where it has caused economic damage by killing grapevines or reducing yields in vineyards (Leach et al. 2019).
From being present in a single county in Pennsylvania in 2014, L. delicatula has spread quickly so that by early 2020 it had been found in 10 eastern states (80 counties total), and populations were considered established and were quarantined in six of these states (Delaware, Maryland, New Jersey, Pennsylvania, Virginia, and West Virginia) (New York State IPM 2020). While adult L. delicatula can fly, it is assumed that much of this dispersal is due to incidental movement of eggs, nymphs, and adults by humans (Urban et al. 2020).
There have been reports of resident natural enemies attacking L. delicatula, including the egg-parasitic wasp Ooencyrtus kuvanae (Howard) (Hymenoptera: Encyrtidae), which was introduced from Asia to control gypsy moth (Lymantria dispar (L.)) (Lepidoptera: Erebidae) (Liu and Mottern 2017, Liu 2019), and generalist arthropod predators, i.e., predatory hemipterans including the native wheel bug (Arilus cristatus (L.)) (Hemiptera: Reduviidae) and predatory stink bug (Apoecilus cynicus (Say)) (Hemiptera: Pentatomidae) (Barringer and Smyers 2016), Chinese mantis (Tenodera sinensis (Saussure) (Mantodea: Mantidae) (H. Leach, personal communication), spiders (e.g., Argiope trifasciata (Forskål)) (Araneae: Araneidae), and vespid wasps (E. H. Clifton, personal observation), but so far there is little evidence to suggest that these natural enemies significantly reduce L. delicatula populations. In contrast, Clifton et al. (2019) described epizootics caused by two native entomopathogenic fungi, Batkoa major (Thaxt.) Humber and Beauveria bassiana (Bals.-Criv.) Vuill., in dense L. delicatula populations in Berks County, Pennsylvania, in October 2018. Lycorma delicatula adults that had been killed by B. major and B. bassiana were also found in 3 and 12 additional locations, respectively, throughout Berks County in 2018 (EHC and AEH, unpublished data). In any location where we found L. delicatula killed by B. major, we also found cadavers of L. delicatula killed by B. bassiana.
To date, management of the L. delicatula populations in North America involves detection, followed by use of various chemical insecticides. Since L. delicatula can be found in many diverse habitats on many different hosts, the registered insecticides and application methods differ for homeowners, vineyards, and park managers, as do economic or social thresholds for treatment. Optimization of control methods is in progress (Leach et al. 2019, Urban 2020) and environmentally friendly control approaches are needed. A study by Leach et al. (2019) evaluated insecticides for controlling L. delicatula on peaches and grapes, including some products listed by the Organic Materials Review Institute (OMRI). Unfortunately, the OMRI-listed products were not as effective as the conventional insecticides and, thus, organic producers have limited options for managing L. delicatula (Leach et al. 2019).
Entomopathogenic fungi are important pathogens infecting many hemipterans (Araújo and Hughes 2016), especially leafhoppers and planthoppers (Soper 1985). Some species of entomopathogenic fungi are mass-produced and are commercially available for use as mycoinsecticides. One of the fungi known to infect L. delicatula during 2018 epizootics, B. bassiana, is a well-studied entomopathogen (Feng et al. 1994, Chandler 2017) that is commercially available for use on crops and in forests in the U.S. Little is known about the second fungal pathogen, B. major, and it has not been developed as a mycoinsecticide.
In the current study, we applied B. bassiana to suppress fourth instar and adult populations of L. delicatula in the field. We report on the efficacy of these spray trials and persistence of the sprayed fungal spores, as well as the minimal non-target impacts. We also report the efficacy by contact and from residues of three commercial formulations of mycoinsecticides tested in semi-field studies on L. delicatula adults feeding on potted grapevines.
Materials and Methods
Norristown Farm Park Trials
Field Site
Replicated large-plot field evaluations of a commercial formulation of B. bassiana strain GHA known as BoteGHA ES (Certis USA, L.L.C., Columbia, MD; EPA registration No. 82074-1) were conducted at Norristown Farm Park located at 2500 Upper Farm Road, East Norriton, PA 19403 (Montgomery County, 40°08′43.8″N, 75°20′32.9″W). Four test sites with high populations of early instar L. delicatula and an abundance of preferred host plants, including A. altissima, black walnut (Juglans nigra L.), oriental bittersweet (Celastrus orbiculatus Thunb.), and wild grapes (Vitis spp.) were selected from the park’s 680 acres in late June 2019. An additional condition in selecting test sites was that they would contain host plants in 10 m wide fencerows bounded by non-host corn and soybean fields rather than larger blocks of wooded areas. These criteria attempted to minimize L. delicatula populations moving from untreated areas into the plots during the post-spray evaluation period. In addition, this plot width was chosen because the range of our application equipment was limited to only 10 m from the edge of the plots. To reduce population variation between the four replicate sites, we randomly paired each BoteGHA spray plot within the replicate site with a contiguous water-only sprayed control plot of equal size, in a split-plot design. The closest distance between any two replicate sites was 485 meters. All BoteGHA test plots and their adjacent control plots were each 15.2 m long by 9.1 m wide (Supp Fig. S1 [online only]).
The first spray application was made on 2 July 2019, mostly targeting fourth instar L. delicatula. On 14 August 2019, the efficacy of BoteGHA was tested on the more mobile adult L. delicatula. For this trial, we expanded the existing plots to 30.5 m long by 9.1 m wide (Supp Fig. S2 [online only]). One of the sites sprayed in July was not used in August because the expanded plot size could not fit between an adjacent parking lot and a bridge (near the field plots in the northwest corner on Supp Fig. S1 [online only]). A new site that also had dense populations of L. delicatula and could support the expanded plot size for the August spray trial was established near the previous site (northwest corner on Supp Fig. S2 [online only]).
Mycoinsecticide Applications
To dilute the mycoinsecticide for application, we mixed 6.9 ml of BoteGHA ES per L of water, creating a suspension with approximately 1.05 × 108 spores ml−1. From the roads adjacent to the plots, using a tractor with a three-point hitch to pull a power take-off (PTO) driven air blast orchard/vineyard sprayer (Model CDP14P100P, Durand Wayland, LaGrange, GA) with a GunJet handgun attachment (Model AA43HC, Spraying System Co., Wheaton, IL), the mycoinsecticide was applied to runoff (300 PSI at 19 liters per min) to a height of about 9.1 m and a depth of 9.1 m. Before spraying the mycoinsecticide, the control plots were sprayed first with water.
Analyzing B. bassiana Conidial Deposition and Persistence on Foliage
Leaves were sampled to quantify the number of viable conidia per cm2 of leaf area immediately after the BoteGHA spray applications. Leaves were also sampled 1, 6, 9, and 14 d after the 2 July trial, and daily for 7 d after application for the 14 August trial. On each of these sampling dates, three sets of 10 leaves per leaflets were collected from random locations within each treatment plot that had been sprayed with BoteGHA. Leaf samples were collected from control plots on the day of spray application only. Samples were taken from a variety of tree species within the plot, although most samples were from A. altissima. Each set of 10 leaves per leaflets was placed in a new, 4-L-zip-lock freezer bag (Walmart #564265379), labeled, and placed in a cooler on ice until processed.
To remove conidia from the surfaces of leaves, 100 ml of sterile 0.1% Tween 80 surfactant (CAS 9005-65-6, Acros Organics, The Hague, Netherlands) was added to each bag. The bags were vigorously agitated by hand for 30 s after adding 0.1% Tween, and then left to soak for 1 h. After soaking, the bag was shaken again vigorously for 15 s, before removing 1 ml of the resulting suspension using a sterile pipette tip. A dilution series using sterile deionized water, up to 10 E−2, was made for each sample. The surface of 2 replicate 9 cm Petri plates containing sterile selective agar media was inoculated with 0.1 ml from each dilution (0, −1, and −2) and spread with a sterile spreader. Selective medium was modified from Korosi et al. (2019) comprising: 3.9% powdered potato dextrose agar (Oxoid, Hampshire, United Kingdom), 0.016% Syllit (Ceres International, West Chester, PA), 0.01% chloramphenicol >98% (CAS 56-75-7, Sigma–Aldrich, Darmstadt, Germany) and Penicillin-Streptomycin (10,000 units and 10 mg, respectively; P4333, Sigma–Aldrich, Darmstadt, Germany). Penicillin-Streptomycin was added after autoclaving and when the agar had cooled but was still liquid, to avoid denaturation.
Plates were incubated at 25°C in the dark for 5 d for the fungus to form pure, white B. bassiana colonies that were easily distinguished from other microbial growth. Count data from the two replicate plates with the most appropriate dilution were logged and used to calculate the number of viable B. bassiana conidia cm−2 of leaf area.
The total leaf area for each set of 10 leaves was calculated using the phone app ‘Leafscan’ (http://www.leafscanapp.com/). Each leaf was removed from the zip-lock bag and placed on a white sheet of card within a 15 cm square demarked by four corner dots. The app uses the phone camera to photograph and calculate the area of the leaf. Each measurement was logged in the data manager within the app, and the cumulative leaf area from all 10 leaves from each sample bag was calculated.
Impact of Field Application on Densities of Fourth Instar Lycorma delicatula
For the fourth instar trial, we used timed visual counts to estimate the total number of L. delicatula on trees and other plants in five randomly chosen locations within each plot before spraying, and 12 and 14 d after spraying. Each timed count lasted for 2 min. Two counters estimated L. delicatula numbers in each plot on 1 July (1 d before spray) and again on 16 July (14 d after spray). Another counter estimated L. delicatula numbers in the plots on 14 July (12 d after spray) using the same method.
Impact of Field Application on Lycorma delicatula Adults and Non-targets
Rearing to Assess Adult Mortality
To assess the efficacy of BoteGHA applications to suppress L. delicatula adults, we randomly sampled adults from field plots after the August spray trial and reared them on potted A. altissima plants for daily monitoring. Ailanthus altissima plants were started from seeds and grown in a greenhouse (22.5°C, 16:10 (L:D) h) at Cornell University in 3.8-liter pots with soilless potting media (LM-111, Lambert, Rivière-Ouelle, Québec, Canada) with regular watering and fertilization. Before they were used in experiments, A. altissima plants were approximately 0.6–1.0 m tall with a minimum of six leaves on the main stem. On 9 August, the potted A. altissima plants were moved to a shade house at the Pfeiffer Turf and Ornamental Research Farm on the Penn State Berks campus in Reading, Pennsylvania, which is located within the L. delicatula quarantine. Plants were watered as needed daily in the shade house.
On 15 August 2019, 1 d after plots were sprayed, live L. delicatula adults were randomly sampled from the plots at Norristown Farm Park. Approximately 50 L. delicatula adults were collected from each plot and taken to the shade house (approximately 71 km away) on the same day. The 50 L. delicatula adults from each plot were divided among five A. altissima plants, resulting in approximately 10 adults per plant. Bamboo poles (approximately 1.5 m tall) were used as stakes alongside plant stems to support plastic woven mesh nylon insect rearing bags (L 100 cm × W 66 cm) (BugDorm DC3170, MegaView Science Co., Ltd., Taiwan) enclosing the foliage. The nets were tied off at the bottom of the plant and the base of the bamboo pole to prevent L. delicatula escape.
The caged A. altissima plants and L. delicatula adults were checked daily for mortality from 16 to 25 August and on 29 August. During mortality checks, L. delicatula cadavers were removed from cages, transferred to sealed individual 30-ml cups containing a piece of moistened paper towel. Cadavers were checked 7–14 d later for fungal outgrowth by B. bassiana. Corrected mortality after 14 d was determined using Abbott’s correction (Abbott 1925). Two plants assigned for the control treatment were not included in the data analysis because they were noticeably smaller (<0.60 m tall), their leaves wilted just a few days after the experiment began, as they might have been damaged in transit to Pennsylvania.
Sampling Dead Adults and Non-targets From Groundcloths
On the same day of spraying plots (14 August), we set up four groundcloths in each plot around A. altissima or J. nigra trees that hosted populations of L. delicatula adults. These groundcloths were used for catching L. delicatula and non-targets that fell from trees. Groundcloths were 3.7 × 3.7 m, cut from woven white polypropylene fabric (WGC 12, DeWitt, Sikeston, MO). An incision was made from one end towards the center so that it could be wrapped around the tree trunk base. Groundcloths were attached to bases of tree trunks with a staple gun and the edges of each groundcloth were secured with multiple stakes.
We collected L. delicatula cadavers and any non-target arthropods from groundcloths on 18, 21, 24, and 29 August (= 4, 7, 10, and 15 d after spray application). After samples were brought into the lab, each cadaver was checked to verify whether the body was intact. Some of the bodies of these L. delicatula cadavers were partially eaten or hollowed out by scavengers, e.g., ants, yellowjackets, and slugs. These partially eaten cadavers and any molted exuviae were excluded from data analysis. To promote fungal outgrowth with large numbers of L. delicatula cadavers from each sampling date, L. delicatula cadavers from each plot were placed into humid containers. For each of these containers, a piece of plastic lighting louver was cut to fit at mid-height inside of a 13.2-liter food storage container (PFSH-6, Winco, Boise, ID). The bottom of the container was filled with water before dampened paper towels were laid on the piece of lighting louver. Lycorma delicatula cadavers were arranged on the paper towels with some space between different cadavers. The container’s lid was sealed to maintain 100% RH inside. Containers with these cadavers were kept at room temperature (20–22°C) for 7–14 d to allow for fungal outgrowth. Some cadavers already had B. bassiana outgrowth when they were collected from groundcloths. For each plot and sampling date, we recorded the total numbers of L. delicatula cadavers, with or without B. bassiana outgrowth, and the final percentage of those cadavers that had fungal outgrowth from B. bassiana infections.
Non-target arthropods collected on groundcloths were stored at 4°C until they were identified to species, when possible, or to family. After identification, the non-targets were put in 30-ml cups containing a piece of moistened paper towel and sealed with a lid for 7–14 d to allow for fungal outgrowth.
On 4 September, the four trees in each plot with attached groundcloths were treated with injections of dinotefuran to kill surviving L. delicatula adults and in order to estimate the density of the remaining L. delicatula populations in the plots. We injected 0.79 ml Dinocide (12% dinotefuran, J. J. Mauget Company, Arcadia, CA), per cm of trunk diameter at breast height (DBH). Lycorma delicatula feeding from trees injected with dinotefuran would die within 48 h (Urban 2020, B. Walsh, personal communication). Lycorma delicatula cadavers were collected from the groundcloths on 5–6 September and stored at 4°C before counting. The counts were adjusted for tree size by dividing the total numbers by tree DBH.
Verifying Mortality From BoteGHA or Native Beauveria With Molecular Tools
For any L. delicatula cadaver that was recovered from groundcloths in control plots and showed signs of Beauveria fungal outgrowth, before or after it was brought into the lab, we attempted cultures on selective media before DNA extractions and polymerase chain reaction (PCR). Isolates that were hypothesized as Beauveria spp. were cultured from L. delicatula or non-target cadavers by transferring the fungus to selective agar medium, following Goettel and Inglis (1997). A small surface area containing conidia on the insect cuticle was swabbed with a sterile cotton-tip applicator and conidia that had been collected were transferred to 6-cm Petri dishes containing selective media. The medium, selective for Beauveria cultures, was adapted from Chase et al. (1986), with 30 g wheat germ liter−1, autoclaved and then filtered through cheesecloth, before adding 0.25 g liter−1 chloramphenicol, 0.20 g dodine liter−1, 0.01 g crystal violet liter−1, and 15 g agar liter−1 before autoclaving again. Selective media plates were sealed with Parafilm and placed in an incubator (20°C, 0:24 (L:D) h). After 14 d, cultures of Beauveria isolates were stored at 4°C until DNA extractions were completed. For positive controls, to verify infection by B. bassiana GHA, we diluted a suspension of BoteGHA ES with deionized water and spread it on plates with the same selective media to obtain cultures and extract DNA. We were able to culture Beauveria and extract DNA from 6 of the 32 cadavers with fungal outgrowth that were collected from groundcloths in the control plots during 21–29 August. Because there were 250 L. delicatula cadavers from BoteGHA plots with Beauveria outgrowth and under the assumption that most of them were killed by B. bassiana GHA we only created cultures and extracted DNA on a random subset of 26 L. delicatula cadavers from these plots; these cadavers were collected from groundcloths between 21 and 29 August.
For an isolate presumed to be a species of Beauveria, a small agar plug (<1 mm2) from the culture plate was transferred to a 6-cm Petri dish containing ~6 ml of sterile potato dextrose broth (Code No. 7585, Neogen, Lansing, MI), sealed with Parafilm, and then placed in an incubator (20°C, 0:24 (L:D) h). After 72–96 h, a piece of mycelium (approximately 10 mg) was collected with sterile forceps and placed into a 1.5 ml bead beater tube. After bead-beating the sample for 60 s at 48 krpm in a Mini-BeadBeater (BioSpec Products, Inc.), fungal genomic DNA was extracted using a DNeasy Plant Mini Kit (Qiagen).
For any isolate that was presumed to be a Beauveria sp., bidirectional nucleotide sequences were determined for the B locus (Bloc) nuclear intergenic region. A region of Bloc was amplified and sequenced using the primer pair B22-deg × B3.3R, following PCR conditions described by Rehner et al. (2011). The SAP/EXO protocol was used to clean up reaction mixtures prior to sequencing. Sequencing was done by Cornell University Institute of Biotechnology on an ABI 3730x1. Chromatograms and sequences were edited/trimmed, assembled, and aligned using Geneious Prime software (2019.0.4; Biomatters Ltd.), resulting in contigs with 959–969 base pairs for the Bloc region. We then used ClustalW multiple alignment in Geneious, including B. bassiana GHA as a positive control, to verify whether the non-targets and the L. delicatula adults collected from groundcloths were killed by B. bassiana GHA (in BoteGHA) or from different B. bassiana strains that naturally occurred in the field. Sequence data were checked with the National Center for Biotechnology Information (NCBI) nucleotide database (https://blast.ncbi.nlm.nih.gov/Blast.cgi).
Mycoinsecticide Trials for Adult Lycorma delicatula as Direct Sprays and Residuals on Potted Grapevines
We evaluated the direct contact efficacy of B. bassiana GHA formulated as BoteGHA ES at two label-recommended field application rates (2.4 and 4.8 liters concentrate ha−1) and Aprehend (ConidioTec LLC, Centre Hall, PA), which also uses B. bassiana strain GHA in a pre-mixed, oil-based formulation (Table 1). Additionally, we evaluated PFR-97 WDG (Certis USA), which uses the fungus Isaria fumosorosea Wize, Apopka Strain 97, at label-recommended rates of 1.12 and 2.24 kg ha−1 (Table 1). Further, we evaluated the residual efficacy of the higher rate of BoteGHA and Aprehend after application to grape plants. Trials were conducted at the Penn State Berks campus in Reading, PA. We used Riesling grapes (Vitus vinifera L. var. Chardonnay) that were grafted on SO4 and 3309 rootstocks in March 2019. Grapes were grown in 11-liter pots in greenhouses at the Fruit Research and Extension Center (FREC; Biglerville, PA) with regular watering and fertilization. The potted grapes were brought to the Pfeiffer Turf and Ornamental Research Farm on the Penn State Berks campus in May 2019. Fungicides, including Captan, Dithane (mancozeb), and Pristine (pyraclostrobin and boscalid), were applied to grapes at both FREC and at Penn State Berks until June 2019 to allow fungicide residues to dissipate and prevent interference with bioassays.
Mycoinsecticide product dilutions and application rates applied to containers (11.7 cm diameter × 10.5 cm height) with 10 adult Lycorma delicatula per container
Product and label field application rate . | Conidia or CFU in undiluted product . | Dilution of concentrate . | Volume of diluted product applied (ml) . | Total conidia or CFU applied per replicate . |
---|---|---|---|---|
BoteGHA low rate (2.34 liter ha−1) | 2.1 × 1010 conidia ml−1 | 5 ml liter−1 water | 2.5 | 2.6 × 108 conidia |
BoteGHA high rate (4.68 liter ha−1) | 2.1 × 1010 conidia ml−1 | 10 ml liter−1 water | 2.5 | 5.2 × 108 conidia |
PFR-97 low rate (1.12 kg ha−1) | 1 × 109 CFU g−1 | 2.4 g liter−1 water | 2.5 | 6 × 106 CFU |
PFR-97 high rate (2.24 kg ha−1) | 1 × 109 CFU g−1 | 4.8 g liter−1 water | 2.5 | 4.8 × 107 CFU |
Aprehend (Ready to use) | 2.2 × 109 conidia ml−1 | No dilution | 0.2 | 4.4 × 108 conidia |
Product and label field application rate . | Conidia or CFU in undiluted product . | Dilution of concentrate . | Volume of diluted product applied (ml) . | Total conidia or CFU applied per replicate . |
---|---|---|---|---|
BoteGHA low rate (2.34 liter ha−1) | 2.1 × 1010 conidia ml−1 | 5 ml liter−1 water | 2.5 | 2.6 × 108 conidia |
BoteGHA high rate (4.68 liter ha−1) | 2.1 × 1010 conidia ml−1 | 10 ml liter−1 water | 2.5 | 5.2 × 108 conidia |
PFR-97 low rate (1.12 kg ha−1) | 1 × 109 CFU g−1 | 2.4 g liter−1 water | 2.5 | 6 × 106 CFU |
PFR-97 high rate (2.24 kg ha−1) | 1 × 109 CFU g−1 | 4.8 g liter−1 water | 2.5 | 4.8 × 107 CFU |
Aprehend (Ready to use) | 2.2 × 109 conidia ml−1 | No dilution | 0.2 | 4.4 × 108 conidia |
Mycoinsecticide product dilutions and application rates applied to containers (11.7 cm diameter × 10.5 cm height) with 10 adult Lycorma delicatula per container
Product and label field application rate . | Conidia or CFU in undiluted product . | Dilution of concentrate . | Volume of diluted product applied (ml) . | Total conidia or CFU applied per replicate . |
---|---|---|---|---|
BoteGHA low rate (2.34 liter ha−1) | 2.1 × 1010 conidia ml−1 | 5 ml liter−1 water | 2.5 | 2.6 × 108 conidia |
BoteGHA high rate (4.68 liter ha−1) | 2.1 × 1010 conidia ml−1 | 10 ml liter−1 water | 2.5 | 5.2 × 108 conidia |
PFR-97 low rate (1.12 kg ha−1) | 1 × 109 CFU g−1 | 2.4 g liter−1 water | 2.5 | 6 × 106 CFU |
PFR-97 high rate (2.24 kg ha−1) | 1 × 109 CFU g−1 | 4.8 g liter−1 water | 2.5 | 4.8 × 107 CFU |
Aprehend (Ready to use) | 2.2 × 109 conidia ml−1 | No dilution | 0.2 | 4.4 × 108 conidia |
Product and label field application rate . | Conidia or CFU in undiluted product . | Dilution of concentrate . | Volume of diluted product applied (ml) . | Total conidia or CFU applied per replicate . |
---|---|---|---|---|
BoteGHA low rate (2.34 liter ha−1) | 2.1 × 1010 conidia ml−1 | 5 ml liter−1 water | 2.5 | 2.6 × 108 conidia |
BoteGHA high rate (4.68 liter ha−1) | 2.1 × 1010 conidia ml−1 | 10 ml liter−1 water | 2.5 | 5.2 × 108 conidia |
PFR-97 low rate (1.12 kg ha−1) | 1 × 109 CFU g−1 | 2.4 g liter−1 water | 2.5 | 6 × 106 CFU |
PFR-97 high rate (2.24 kg ha−1) | 1 × 109 CFU g−1 | 4.8 g liter−1 water | 2.5 | 4.8 × 107 CFU |
Aprehend (Ready to use) | 2.2 × 109 conidia ml−1 | No dilution | 0.2 | 4.4 × 108 conidia |
One day prior to mycoinsecticide trials, L. delicatula adults were collected from field sites near the Penn State Berks campus and they were kept overnight in the shade house feeding on potted grape plants inside of rearing cages (Cat. No. 1462W, BioQuip, Rancho Dominguez, CA). Both types of assays on potted grapes were begun on 21 August 2019. Contact activity was conducted by directly spraying L. delicatula adults in groups of 10, contained in 940 ml Kraft Brown Cardboard Deli/Soup Bar containers (diameter: 11.7 cm, height: 13.5 cm) with the test solutions. Mycoinsecticide applications were done in the early morning, to keep L. delicatula adults out of direct sunlight during the process of transporting, counting and using them for mycoinsecticide trials. Aqueous-based formulations were applied using Grainger spray bottles (BoteGHA, PFR-97, and water control), and the ready to use, oil-based product Aprehend was applied using the ULV spray applicator supplied by the manufacturer (SKU 30101–50, ConidioTec LLC, Centre Hall, PA). The total amount of spray mixture used for direct contact sprays was 2.5 ml for BoteGHA, and PFR-97 and water control formulations, and 0.225 ml for Aprehend, per container. The volume and application rates were different according to formulation type and the resulting infective units (CFU or conidia) applied per container also differed (Table 1). Ten L. delicatula adults were treated per container and 10 replicate applications were conducted for each treatment (n = 100 per treatment). To avoid suffocation due to excess spray solution at the bottom of the cups, the cups were placed upside down with polyester netting covering the bottom so that excess solution would drain out. After allowing cups to dry for 10 min, the exposed L. delicatula adults were evaluated for mortality. Live individuals were transferred to unsprayed potted grapes covered with nylon mesh insect rearing sleeves (same model used for adults on potted A. altissima). Potted grapes were kept outside in a turf area on groundcloth to prevent grass and weed growth in the testing area. The potted grapes were watered when necessary. Adult mortality was assessed at the transfer from the cups to the potted grapes, and 5 days after treatment (DAT) (26 August) and 9 DAT (30 August).
Additionally, the residual efficacy of the higher rates of BoteGHA (4.68 liter ha−1) and Aprehend (pre-mix ULV; 2.2 × 109 CFUs ml−1) was tested by spraying the grapes to wet using approximately 25 ml of BoteGHA solution (5.25 × 109 conidia plant−1) and 2.25–2.5 ml Aprehend (4.95 × 109 conidia plant−1). Plants dried for 30 min before 10 naive L. delicatula adults were added to each plant. Ten replicate plants were used per treatment (n = 100 per treatment).
Data Analysis
All data analyses were performed in SAS 9.4 (2019). For conidial deposition and persistence on foliage, we compared B. bassiana CFUs per cm2 foliage for different spray trials (2 July vs 14 August) using a one-way analysis of variance (ANOVA) with Fisher’s least-significant-difference test, using the PROC ANOVA statement. We performed separate comparisons of CFUs at different time points (0, 1, and 6 DAT). The same model was used to compare B. bassiana CFUs in each replicate site (plot #) on day 0 and 1 DAT in the 2 July trial, after observing some differences in conidial deposition. We used the log10 transformation on the numbers of B. bassiana CFUs per cm2 foliage before data analysis.
For the visual counts in the July field trial, we used paired t-tests with the PROC TTEST statement to compare the total numbers of L. delicatula nymphs in each control plot with the numbers in the adjacent treatment plots. We used the natural-log transformation on total counts to normalize residuals, and analyses were conducted for each sampling date.
For comparisons of adult L. delicatula survival times on potted A. altissima plants during the August trial, a Cox proportional hazards model was performed using the PROC PHREG statement.
For the L. delicatula cadavers collected from groundcloths, we used a mixed-model ANOVA with the PROC MIXED statement. Treatment (control vs BoteGHA), sampling date (18, 21, 24, 29 August), and their interaction were fixed effects. We included plot and the interaction of block and treatment as random effects. We analyzed the cumulative numbers of L. delicatula cadavers as the dependent variable and applied a natural-log transformation to normalize residuals.
For the L. delicatula adults treated with mycoinsecticides and reared on potted grapes, multiple comparisons of numbers of dead adults were done at each time point using a one-way ANOVA with Fisher’s least-significant-difference test, using the PROC ANOVA statement.
Results
Norristown Farm Park Trials
Conidial Deposition and Persistence on Leaves
Very few contaminating microbes were present on the plates for all samples from the first trial (2 July). However, increasing levels of contamination were present in plates from the second trial (14 August), likely due to the accumulation of honeydew from L. delicatula activity and resulting sooty mold and other fungi. Regardless, it was still easy to distinguish and count Beauveria colonies on these plates as no inhibition from competing fungi or microbes was observed. There were no quantifiable B. bassiana colony forming units (CFUs) on foliage in the control plots immediately after spraying (day 0) for both trials.
On day 0 in the plots sprayed with BoteGHA, B. bassiana CFUs on foliage were 2.5 × 104 and 3.0 × 104 CFUs per cm2 leaf surface for the July and August trials, respectively (Fig. 1A). The B. bassiana CFUs on day 0 were not significantly different between the July and August trials (F1,22 = 1.19, P = 0.2877). On day 0 in the July trial, B. bassiana CFUs in replicate site #4 were significantly lower than the CFUs for sites #1 and #3 (F3,8 = 4.42, P = 0.0412), but not significantly different than site #2 (Fig. 1B). However, there were no significant differences between replicates sites in the August trial on day 0 (Fig. 1C) (F3,8 = 0.78, P = 0.5386).
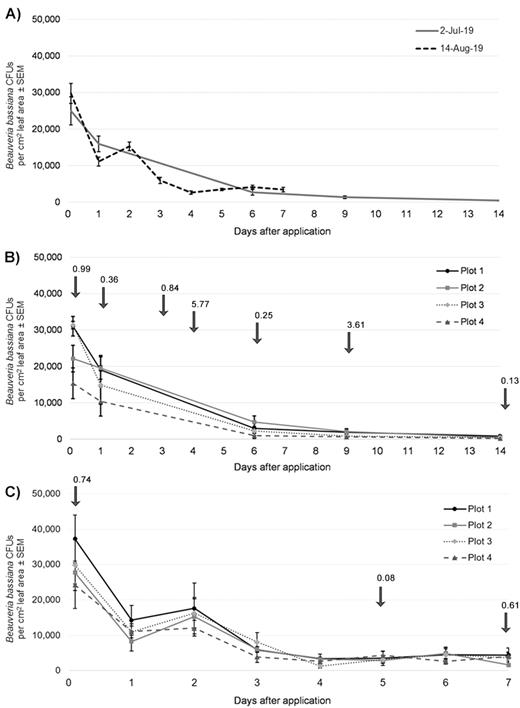
Persistence of Beauveria bassiana colony-forming units on leaves in the field plots at Norristown Farm Park. Error bars represent standard errors of means. (A) Means across replicate sites (plots) comparing the persistence of B. bassiana colony-forming units on leaves for the two trials. (B) Leaf samples were taken immediately after application (day 0), and 1, 6, 9, and 14 d after spraying BoteGHA against Lycorma delicatula nymphs on 2 July 2019. (C) Leaf samples were taken immediately after application (day 0), and then daily for up to 7 d after application against L. delicatula adults on 14 August 2019. Arrows and data labels (aligned above days on x-axis) in (B) and (C) represent total rainfall (cm) for the Philadelphia area (26 km from Norristown Farm Park).
One DAT, B. bassiana CFUs were 1.6 × 104 and 2.1 × 104 CFUs per cm2 leaf surface during the July and August trials, respectively (Fig. 1A). The counts of B. bassiana CFUs 1 DAT were not significantly different between the July and August trials (F1,22 = 2.19, P = 0.1527). Furthermore, for 1 DAT there were no significant differences between replicate sites in both the July (F3,8 = 1.24, P = 0.3587) and August trials (F3,8 = 0.64, P = 0.6076) (Fig. 1B and C).
Six DAT, the densities of B. bassiana CFUs on foliage were 2.7 × 103 and 4.1 × 103 CFUs per cm2 leaf surface during the July and August trials, respectively (Fig. 1A). Still, the B. bassiana CFUs 6 DAT were not significantly different between the two trials (F1,22 = 2.53, P = 0.1262). These slight differences in CFU persistence could probably be attributed to the fact that there was 8.20 cm of rainfall around the field site between 2 and 8 July (0–6 DAT), but only 0.81 cm of rainfall between 14 and 20 August (Fig. 1B and C) (Supp File S3 [online only]). Regardless of varying weather and rainfall events, the densities of B. bassiana CFUs at 0, 1, and 6 DAT were not significantly different and conidial persistence was similar between trials.
Changes in Lycorma delicatula Nymph Population Density
Based on 10 min visual counts of L. delicatula nymphs, the average total counts on 1 July (1 d before spray) were 358 ± 95 nymphs in control plots and 327 ± 100 in adjacent BoteGHA plots and these counts were not significantly different (t-test = 3.11, df = 3, P = 0.0530). For the population densities on 14 July (12 d after spray), the average total 10 min counts were 266 ± 65 in control plots and 142 ± 30 in adjacent BoteGHA plots, and these counts were significantly different (t-test = 6.63, df = 3, P = 0.0070) (Fig. 2). For population densities on 16 July (14 d after spray), the average total counts were 251 ± 118 in control plots and 130 ± 67 in adjacent BoteGHA plots, and these average counts were significantly different (t-test = 4.82, df = 3, P = 0.0170) (Fig. 2). Based on these 16 July counts, the density of L. delicatula nymphs in BoteGHA plots was 48% lower than those in the adjacent control plots. The average ratio of population densities in control versus BoteGHA plots was 1.13 ± 0.04 on 1 July, but then the ratio increased to 2.14 ± 0.31 on 16 July. In other words, population densities in the field plots were similar before spraying, but then approximately twice as many L. delicatula were found in the control plots compared to adjacent BoteGHA plots 14 d after spraying.

Visual counts of Lycorma delicatula population densities in field plots at Norristown Farm Park. Counts were made 1 d before the 2 July application and again on 14 July (12 d later) and 16 July (14 d later). Box plots show the median (solid line), interquartile range, and range of population densities. Asterisks denote significant differences between treatments based on paired t-tests.
Adult Lycorma delicatula Field Trial and Subsequent Bioassays
Survival of Field Collected Lycorma delicatula Adults on Potted A. altissima
After 14 d on potted plants, 51% of the adults sampled from the BoteGHA plots were dead, compared to 14% mortality for the adults from control plots (Fig. 3). Using Abbott-corrected survival, we estimated that the BoteGHA application resulted in 43% mortality for L. delicatula adults after 14 d. The survival times of these adults were significantly different between treatments, based on a Cox proportional hazards model (Log-rank χ 2 = 50.63; df = 1; P < 0.0001). Among the dead L. delicatula adults collected and reared from BoteGHA plots, 83% had fungal outgrowth with B. bassiana, whereas only 9% of those from control plots had B. bassiana outgrowth.

Survival of adult Lycorma delicatula on potted Ailanthus altissima plants kept in a shade house. Adults were sampled from field plots in Norristown Farm Park on 15 August (1 d after spraying). Lines represent the fungal applications versus controls. Letters represent significant differences between treatments for survival curves based on Cox proportional hazards.
Dead Lycorma delicatula Adults Collected From Groundcloths
The fixed effects of treatments (F1,3 = 30.59; P = 0.0116) and sampling date (F3,18 = 106.47; P < 0.0001) were significant, with significantly more L. delicatula cadavers collected from BoteGHA plots over the sampling period compared to control plots (Fig. 4). The interaction of treatment and sampling date was not significant (F3,18 = 1.18; P = 0.3435). By 29 August, we collected a cumulative total of 627 and 1,240 L. delicatula cadavers from groundcloths in the control and BoteGHA plots, respectively. Among these dead L. delicatula adults, 32 from control plots (5.1% of the total) and 250 from BoteGHA plots (20.2% of the total) had fungal outgrowth from B. bassiana. For the final collections of cadavers on 5–6 September, after dinotefuran injections, there was no significant difference between treatments, based on the numbers of adults adjusted for tree size (Supp Fig. S4 [online only]).

The mean numbers (± standard error) of cumulative Lycorma delicatula cadavers recovered from groundcloths in field plots after spray applications on 14 August. The first sampling date was 4 d after application (18 August); the final sampling date was 15 d after application (29 August). Lines represent the fungal applications versus controls. Letters represent significant differences between treatments based on mixed-model analysis of variance.
Confirmation of Lycorma delicatula Mortality From BoteGHA
All six L. delicatula cadavers from control plots with successful Beauveria cultures and DNA extractions were killed by B. bassiana GHA (Supp Table S5 [online only]). We aligned 959 base pairs from the Bloc region and these samples had a 100% match to the positive controls for B. bassiana GHA. Twenty-four out of 26 cadavers from BoteGHA plots were killed by B. bassiana GHA (100% match); however, the other 2 L. delicatula adults were killed by native strains of B. bassiana (Supp Table S5 [online only]). One of these isolates (L. delicatula #50; GenBank MT122124) had a 99.9% match to isolate LHY10A (GenBank JN849669) and four other isolates in the same study, all collected from emerald ash borers, Agrilus planipennis Fairmaire (Coleoptera: Buprestidae) (Johny et al. 2012). The other isolate (L. delicatula #61; GenBank MT122133) had a 99.8% match to isolate L28A (GenBank JN849673) and six other isolates from the same study, which were also collected from emerald ash borers (Johny et al. 2012). This result confirms that there was some level of naturally occurring infection caused by native strains of B. bassiana at Norristown Farm Park in August 2019.
Non-targets From Groundcloths
Among 1,867 L. delicatula cadavers that were collected from groundcloths between 18 and 29 August 2019, we found only 59 non-target insects, and only 9 of those were confirmed to be killed by B. bassiana strain GHA (Supp Table S6 [online only]). One non-target (#46; GenBank MT122109), a tiphiid wasp, was killed by a native B. bassiana with 98.2% similarity to isolate CHE-CNRCB 35 (GenBank MH203427) that was isolated from a scarab larva (Serna-Domínguez et al. 2019). Some of the non-targets killed by B. bassiana GHA included planthoppers: three individuals were Flatormenis proxima (Walker) (Hemiptera: Flatidae) and one was Acanalonia conica (Say) (Hemiptera: Acanaloniidae). Some of these native planthoppers have been observed on the same host trees as L. delicatula in southeastern Pennsylvania (Fig. 5). Our list of the dead non-target individuals (Supp Table S6 [online only]) collected from field plots includes 22 planthoppers with unknown causes of mortality, 12 of which were Ormenoides venusta (Melichar) (Hemiptera: Flatidae). Some of the non-targets were killed by other fungal entomopathogens, including a dog-day cicada (Neotibicen canicularis (Harris)) infected by a Metarhizium species (Hypocreales: Clavicipitaceae).
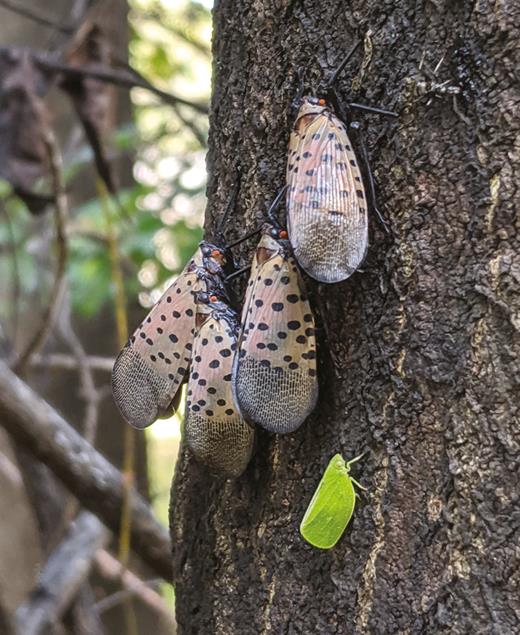
A flatid planthopper, Acanalonia conica, observed near Lycorma delicatula adults on Ailanthus altissima. Photo taken on 1 October 2019 in a public park in Reading, Pennsylvania. Photographer: Eric H. Clifton.
Semi-Field Mycoinsecticide Trials With Lycorma delicatula Adults on Grapes
All direct contact treatments had significantly greater mortality than controls at 5 DAT (F7,72 = 34.74, P < 0.0001) and 9 DAT (Fig. 6B and C) (F7,72 = 53.91, P < 0.0001). At 9 DAT, direct contact applications killed 90–100% of L. delicatula adults and all treatments had significantly greater mortality than controls (Fig. 6C). Direct contact applications of the high rate and low rate of BoteGHA killed 63% and 79% of L. delicatula adults 5 DAT (Fig. 6B), and 99 and 100% 9 DAT, respectively (Fig. 6B). The lower rate of BoteGHA had significantly greater efficacy than the higher rate of BoteGHA at 5 DAT (Fig. 6B) and had greater efficacy than the lower rate of PFR-97. PFR-97 killed 58 and 65% of adults 5 DAT (at low and high rates, respectively; Fig. 6B), and 90 and 92% at 9 DAT (at high and low rates, respectively; Fig. 6C). By contrast, 100% of adult L. delicatula exposed to Aprehend by direct spray application died within 10 min after exposure (Fig. 6A).

Mycoinsecticide trials with adult Lycorma delicatula on potted grapes. Bar shading indicates treatment. Letters above bars represent significant differences between treatments on a day of sampling, based on multiple comparisons with Fisher’s least-significant-difference test. (A) 10 min after treatment (contact). (B) Mean mortality 5 DAT (contact). (C) Mean mortality 9 DAT (contact). (D) Mean mortality 5 DAT (residual). (E) Mean mortality 9 DAT (residual).
Exposure of L. delicatula adults to BoteGHA applied as a residual treatment on grape plants killed 27 and 57% of adults at 5 and 9 DAT, respectively (Fig. 6D and E), whereas adults exposed to plants treated with Aprehend resulted in 80 and 99% mortality at 5 and 9 DAT, respectively (Fig. 6D and E). At both 5 and 9 DAT, residual exposure to Aprehend had significantly higher mortality than BoteGHA.
Discussion
Fourteen days after spraying at Norristown Farm Park, densities of fourth instar L. delicatula were 48% lower in BoteGHA-treated plots compared to control plots, based on visual counts. One major challenge in determining the efficacy of B. bassiana applications for our study was that researchers are still developing methods for estimation of L. delicatula population densities in the variety of landscapes that L. delicatula occupies (Urban 2020). Detection lag, population estimates, and development of control strategies are challenges for all invasive species (Mehta et al. 2007). Additionally, movement of L. delicatula into the plots may have reduced the apparent impact of BoteGHA in this study because at least some nymphs from the edges of the plots and from the controls probably moved into the treated plots after the residues of BoteGHA diminished, inflating estimates of survival. After the establishment of our trial, recent studies have found that fourth instar nymphs can move >50 m in natural landscapes over the course of 1 wk (K. Hoover, personal communication).
In the August trial, we quantified higher numbers of L. delicatula cadavers (= more mortality) in BoteGHA plots compared with controls (Fig. 4). However, by the time the surviving adults that remained on trees were killed using dinotefuran injections on 4 September, there were no significant differences in the numbers of L. delicatula adults (Supp Fig. S4 [online only]). Late August to early September precedes a period of increasing aggregation and mating by L. delicatula adults in southeastern Pennsylvania (J. Urban and H. Leach, personal communication). One study reported that L. delicatula adults can fly ~40 m during one flight bout after launching from trees (Baker et al. 2019). Based on these recent studies and the fact that the dinotefuran injections were made 3 wk after the August application, it is very likely that some of the L. delicatula populations moved in and out of our field plots between the dates of spraying and sampling cadavers of dinotefuran-killed L. delicatula. Future studies will hopefully benefit from increasing knowledge about instar-specific dispersal of L. delicatula and improved methods for quantifying L. delicatula densities by instar, especially because L. delicatula behavior can vary among different instars (Urban 2020).
Lycorma delicatula adults were sampled from plots and reared on A. altissima plants as another method to measure the efficacy of BoteGHA applications during the August spray trial. We had greater confidence in this method because the adults collected just 1 d after spraying would represent the population that had direct and/or 1 d of residual exposure to B. bassiana and had limited time to disperse. The experiment with potted A. altissima plants showed that 43% of the adults from BoteGHA plots had died by 14 d, after adjusting for the 14% mortality in control plots (Fig. 3). It should be mentioned that there was a heat wave in Reading, PA (where the shade house used for rearing was located) on 18–22 August (3–7 d after sampling; Fig. 3), with high temperatures of 31.5°C or more on five consecutive days (Supp File S3 [online only]). High temperatures like these can be fungistatic to B. bassiana, even after the infective propagules have invaded hosts (Zimmermann 2007). More L. delicatula adults died from infections when temperatures cooled down following 22 August (8 d after spraying). The weather records for Philadelphia (25 km from Norristown Farm Park, which was closer than Reading) show that the high temperatures were 32°C or higher on 18–22 August (Supp File S3 [online only]), so the environmental conditions at the application site (Norristown Farm Park) were consistent with the rearing site (Reading).
The observed persistence of B. bassiana CFUs on foliage (Fig. 1) corresponds with BoteGHA’s label recommendations to reapply when needed every 5 to 7 d. Mycoinsecticides that use fungal entomopathogens like B. bassiana perform best when there is direct contact with target pests (Jaronski 2010). The formulation of a mycoinsecticide also plays an important role in its efficacy and residual activity (Jenkins and Thomas 1996, Inglis et al. 2000). Also, environmental conditions such as UV radiation, extreme temperatures, and rainfall can all affect the rate of degradation for B. bassiana CFUs on plant surfaces (Inglis et al. 2000, Jaronski 2010).
Based on the molecular data that found some different strains of B. bassiana infecting L. delicatula, some naturally occurring mortality caused by native B. bassiana occurred in Norristown Farm Park. A 2019 survey of L. delicatula adults at one field site in Berks County, Pennsylvania (neighboring Montgomery County, the site of Norristown Farm Park) found that as many as 22% of adults died from naturally occurring B. bassiana infections during mid-October (E. H. Clifton, unpublished data), but the prevalence of these infections during July-August has not been studied. We used molecular identification tools on a subsample of B. bassiana isolates among the 282 L. delicatula cadavers that were collected from groundcloths and had fungal outgrowth. It should be noted that not every insect killed by B. bassiana would show fungal outgrowth, especially for cadavers that laid on groundcloths for multiple days before sampling. Cadavers with multiple days of exposure to scavengers and saprophytes in the environment, UV radiation, and other abiotic factors could fail to show sporulation (Lacey and Solter 2012). This helps to explain why many of the cadavers from treatment plots did not have fungal outgrowth. Our collections from groundcloths probably underestimated the level of mortality caused by applications of BoteGHA.
Two out of 26 L. delicatula adults (~8%) from plots that were treated with BoteGHA were killed by native strains of B. bassiana during August 2019. It is not known where the treated adults killed by native B. bassiana became infected. Although we had a limited sample size for identifying the fungi on cadavers from the control plots, 6 out of 6 (100%) were killed by B. bassiana GHA. This finding may reflect some flaws in the field plot design and our methodology of collecting cadavers from groundcloths over a period of 2 wk. Two weeks would allow time for exposed L. delicatula to move from treated plots to adjacent control plots. The maximum size of field plots was somewhat limited because the trials were conducted in a public park with a mixed landscape consisting of pathways, forests, and agricultural production.
We did not observe a great deal of non-target mortality after spraying plots with BoteGHA. Previous studies have shown that B. bassiana strain GHA and other strains had minimal impacts on beneficial insects, including parasitoids and generalist predators (Zhu and Kim 2012, Herrick and Cloyd 2017, Martínez-Barrera et al. 2019, Mingotti Dias et al. 2019). Some of the dead insects we found on groundcloths were planthoppers in the families Flatidae and Acanaloniidae (Supp Table S6 [online only]). For many of these non-targets there was no fungal outgrowth. Without fungal outgrowth from B. bassiana, we cannot determine whether BoteGHA killed these insects or if they died from other causes. Searches for ‘Beauveria’ and either ‘Flatormenis proxima,’ ‘Ormenoides venusta,’ or ‘Acanalonia conica’ on Web of Science (https://www.webofknowledge.com) and Google Scholar (https://scholar.google.com/) yielded no results. To our knowledge, there have been no studies exploring the susceptibility of these planthoppers to Beauveria spp., but there have been reports of fungal pathogens infecting other planthoppers (Soper 1985). Alternatively, there is little knowledge about the life histories of these native planthoppers and they could have died for other reasons (e.g., the end of the season, as it was late August).
The immediate mortality from direct applications of Aprehend was caused by its oil-based formulation and not from B. bassiana infection (Fig. 6A). While it is not likely that large scale field applications would result in direct contact with the entire population, the rapid mortality of those individuals hit directly by spray droplets would be advantageous. Additionally, the enhanced residual efficacy of the Aprehend formulation on plant surfaces after application (Fig. 6D and E) also indicates that Aprehend could play a useful role in L. delicatula control operations. Direct applications of BoteGHA and PFR-97 to L. delicatula adults did not result in immediate mortality, but rather occurred at later time points after fungal infections progressed (Fig. 6A–C). The PFR-97 product, containing I. fumosorosea, showed similar efficacy to BoteGHA, with 90–92% mortality at 9 DAT. Of note is that the concentrations of infective propagules (CFUs) in the PFR-97 direct spray treatments (6 × 106 CFU and 1.2 × 107 CFU) were one or two orders of magnitude lower than the high and low application rates of BoteGHA (2.6 × 108 conidia and 5.2 × 108 conidia) and Aprehend (4.4 × 108 conidia).
Repeated applications of any of these mycoinsecticides that were tested may be necessary to manage large populations of L. delicatula adults, especially if they repeatedly enter vineyards from surrounding landscapes. Researchers are still developing economic injury levels (Pedigo et al. 1986) for L. delicatula feeding on grapes and other crops. One challenge for grape growers is that L. delicatula adults will disperse from the surrounding landscape to enter vineyards, and edge effects have, therefore, been observed (H. Leach, personal communication). There are limited options to stop the adults from repeatedly entering vineyards, but exclusion netting and other tools will be explored in the future. Mycoinsecticides with good residual efficacy, as observed with Aprehend on potted grapes, could be helpful for managing these active L. delicatula adults. Targeting applications of fungal entomopathogens to L. delicatula adults during periods of aggregation and mating should be investigated further. Besides direct contact with sporulated cadavers, insects exposed to fungal entomopathogens can horizontally transfer infective spores during close contact and mating events (Kreutz et al. 2004, Barbarin et al. 2012, Ugine et al. 2014).
Lycorma delicatula is a particularly difficult pest to manage because of its varying host plant preferences and the many habitats that it can occupy. Previous studies have shown that applications of B. bassiana can suppress hemipteran pests on crops and ornamentals (Pogetto et al. 2011, Gouli et al. 2012, Gutierrez-Coarite et al. 2018, Portilla et al. 2019). Other studies have explored the suitability of Beauveria spp., including B. bassiana strain GHA, for managing forest pests like the emerald ash borer (Liu & Bauer 2008; Castrillo et al. 2010, 2011; Zhang et al. 2011; Mayerhofer et al. 2015). Given the relative efficacy of the PFR-97 and Aprehend treatments in the grapes trial, in spite of the lower CFU concentrations, future trials will also include these products. Interestingly, we have not yet observed L. delicatula infected by Isaria spp. in the field. Further research is needed to determine the susceptibility of L. delicatula to different genera and species of fungal entomopathogens. Future studies will consider different formulations and application techniques for B. bassiana and I. fumosorosea, targeting applications for earlier L. delicatula instars, i.e., applications after peak egg hatch, and repeated sprays based on the duration of residual activity. The current study highlights the potential of using B. bassiana and I. fumosorosea for biological control of high-density L. delicatula populations in vineyards and semi-natural landscapes with minimal risks to non-targets.
Acknowledgments
This project was supported by the New York State Department of Agriculture and Markets—Contract T00128GG to A.E.H., and the Pennsylvania Department of Agriculture—Agricultural Resource Center, Grant C940000081 to D.J.B., N.E.J., and J.M.U., and Contract 44188504 to A.E.H. We thank Brad Fritz and Michael Dimock (Certis USA) for providing BoteGHA and PFR-97 products for trials. Kenneth Shellenberger II and other park rangers at Montgomery County Norristown Farm Park for cooperation on setting up field plots. John Deere and Company for their support and contributions of equipment. Dr. David Sanford for sharing lab space at Penn State Berks. David Harris, Sarah Stefanik, Dennis Calvin, Julie Urban, Heather Leach, Brian Walsh, Mitchell Hornberger, Jon Elmquist, Caryn Michel, Simona Principato, Liz Deecher, Kathy Wholaver, and Lolita Miller for assistance with field work and data collection. Charles Bartlett for help with identifying non-target planthoppers. Cindy Huebner and Melody Keena for providing Ailanthus altissima seeds and advice on growing these plants in greenhouses. Louela Castrillo for advice on DNA extractions and molecular identification. Stefan Jaronski for advice on experiments and references for the manuscript. Françoise Vermeylen of the Cornell Statistical Consulting Unit for advice on data analyses. Sana Gardescu for reviewing an earlier draft of the manuscript.