-
PDF
- Split View
-
Views
-
Cite
Cite
Roger I. Vargas, John D. Stark, John Banks, Luc Leblanc, Nicholas C. Manoukis, Steven Peck, Spatial Dynamics of Two Oriental Fruit Fly (Diptera: Tephritidae) Parasitoids, Fopius arisanus and Diachasmimorpha longicaudata (Hymenoptera: Braconidae), in a Guava Orchard in Hawaii, Environmental Entomology, Volume 42, Issue 5, 1 October 2013, Pages 888–901, https://doi.org/10.1603/EN12274
- Share Icon Share
Abstract
We examined spatial patterns of both sexes of oriental fruit fly, Bactrocera dorsalis (Hendel), and its two most abundant parasitoids, Fopius arisanus (Sonan) and Diachasmimorpha longicaudata (Ashmead) in a commercial guava (Psidium guajava L.) orchard. Oriental fruit fly spatial patterns were initially random, but became highly aggregated with host fruit ripening and the subsequent colonization of, first, F. arisanus (egg-pupal parasitoid) and, second, D. longicaudata (larval-pupal parasitoid). There was a significant positive relationship between populations of oriental fruit fly and F. arisanus during each of the F. arisanus increases, a pattern not exhibited between oriental fruit fly and D. longicaudata. Generally, highest total numbers of males and females (oriental fruit fly, F. arisanus, and D. longicaudata) occurred on or about the same date. There was a significant positive correlation between male and female populations of all three species; we measured a lag of 2-4 wk between increases of female F. arisanus and conspecific males. There was a similar trend in one of the two years for the second most abundant species, D. longicaudata, but no sign of a time lag between the sexes for oriental fruit fly. Spatially, we found a significant positive relationship between numbers of F. arisanus in blocks and the average number in adjoining blocks. We did not find the same effect for oriental fruit fly and D. longicaudata, possibly a result of lower overall numbers of the latter two species or less movement of F. arisanus within the field.
Oriental fruit fly, Bactrocera dorsalis (Hendel), is among the five most damaging fruit flies in the world (Leblanc and Putoa 2000). It is distributed throughout Asia, from India to Taiwan (but not in Malaysia, Indonesia, or the Philippines) and as far north as southern China (White and Elson–Harris 1992, Clarke et al. 2005). Oriental fruit fly was accidentally introduced into the Commonwealth of the Northern Mariana Islands in 1935 (eradicated since 1965), Hawaii in 1945, Guam in 1948 (eradicated since 1965), Nauru in the 1980s (eradicated since 1999), and Tahiti in 1996 (Leblanc and Putoa 2000). Recently, two Bactrocera spp. have become established on two new continents: Bactrocera carambolae Drew and Hancock, the carambola fruit fly, in Suriname (South America), and Bactrocera invadens Drew, Tsuruta, and White in Kenya (Africa) (Drew et al. 2005, Rousse et al. 2005). With the recent spread of Bactrocera spp. into new areas of the world, there has been renewed interest in biological control for suppression of this important pest complex.
After the introduction of oriental fruit fly into Hawaii in 1945 (Fullaway 1947), the largest program of classical biological control (parasitoid releases) against fruit flies ever was undertaken to reduce serious damage occurring in fruits (Clausen et al. 1965). Thirty-two species of natural enemies were released between 1947 and 1952 (Bess et al. 1961). One of these was Diachasmimorpha longicaudata (Ashmead), which increased rapidly following its release in 1948, but suddenly lost its dominant position during the latter half of 1949 to Fopius vandenboschi (Fullaway), which itself was later superseded by Fopius arisanus (Sonan) (van den Bosch and Haramoto 1953, Ramadan et al. 1992). Since its establishment in Hawaii, F. arisanus has dramatically reduced infestation of fruit through a high level of oriental fruit fly parasitism (65–70%), and has remained the dominant parasitoid species (Haramoto and Bess 1970, Vargas et al. 1993). Given their success in Hawaii, F. arisanus and, to a lesser degree, D. longicaudata and F. vandenboschi have been primary candidates for biological control of the oriental fruit fly complex species in other parts of the world.
The U.S. Department of Agriculture Agricultural Research Service U.S. Pacific Basin Agricultural Research Center has been a world leader in promoting biological control of Bactrocera spp that includes classical, augmentative, conservation, and integrated pest management approaches (Vargas et al. 2012b). Hawaii has been a source of parasitoids for fruit fly control throughout the Pacific region including Australia, Pacific Island Nations, Central America, and South America, not only for Bactrocera spp. but also for Ceratitis and Anastrepha spp. (Vargas et al. 2012b). Recently in 2002, natural enemies were introduced into French Polynesia where oriental fruit fly had invaded in 1996 (Vargas et al. 2007). Scientists from Hawaii and French Polynesia developed a cost-effective sustainable biological program in French Polynesia with potential application over a land area of 4,167 km2 spread over 2,500,000 km2 of ocean by establishing fruit fly natural enemies to suppress oriental fruit fly and related species. A classical biological control program was initiated and the two parasitoids, F. arisanus and D. longicaudata, were shipped, reared, and established in Tahiti from Hawaii. Establishment of F. arisanus reduced not only oriental fruit fly but also two other fruit flies, Bactrocera tryoni (Froggatt), Queensland fruit fly, and Bactrocera kirki (Froggatt) by ≈90%, with parasitism rates ranging from 50 to 65%, and with a subsequent reduction in damage to tree fruits (Vargas et al. 2012a, b). French Polynesia is composed of 118 islands and atolls. From a small laboratory, the French Polynesians have been able to rear parasitoids and release them against oriental fruit fly, as it has not only spread throughout the Society Islands, but also to the Marquesas Islands, expanding the local program to an areawide or regional program. Establishment of F. arisanus in French Polynesia is the most successful example of classical biological control of fruit flies in the Pacific outside of Hawaii and provides important information for introduction into continental areas of South America and Africa where species of the oriental fruit fly complex have recently become established. Scientists are currently introducing F. arisanus into Senegal and Brazil. With the rapid expansion of airline travel and global trade in tropical fruits, there has been an alarming spread of Bactrocera spp. into new areas of the world (i.e., South America and Africa). With a scarcity of field data on spatial dynamics of fruit fly parasitoids and current interest in releases in new areas of the world for control of fruit flies attacking tropical fruit orchards, we felt that a critical evaluation of orchard spatial data to predict the impact of releases was warranted.
In the current study, we report on male and female oriental fruit fly, F. arisanus, and D. longicaudata spatial dynamics over time in addition to their resultant dispersion patterns in a commercial guava (Psidium guajava L.) orchard during two typical fruit production cycles. Stark et al. (1991) previously identified F. arisanus and D. longicaudata as the two most abundant parasitoid species in these orchards. Our findings are of importance to determination of the most economical and sustainable strategy of combating the current spread of oriental fruit fly and related Bactrocera pest species in various parts of the world through releases of biological control agents.
Materials and Methods
A study site (5.91 ha, 1,647 trees) was established within a 194-ha commercial P. guajava orchard (Guava Kai, Kilauea, Kauai, HI). The sampling area was in the center of the study site and contained 900 trees bordered on the east and west by two rows of P. guajava trees and on the north and south by 14 rows. Fruit ripening patterns were similar to those of wild P. guajava and their associated peaks of island-wide oriental fruit fly abundance (Vargas et al. 1989, 1990; Stark et al. 1991). Year-round fruiting was accomplished by an intensive pruning program. Most of the crop was produced between August and December.
The sampling grid (3 by 12 rows) consisted of 36 equal blocks (see blocks discussed in ‘Distribution of Male and Female Oriental Fruit Fly, F. arisanus, and D. longicaudata'); each with 25 trees (5 by 5 rows of trees within a block). For the hurdle model analysis, adjoining blocks were blocks with a common side. Center blocks would have four common sides, corner blocks two common sides, and border blocks three common sides. One tree was chosen at random within each block of 25 trees for a fogging treatment with an insecticide (Stark et al. 1991) on each sample date. Trees were sampled every 2 wk from July through November (a complete growing season for this block) in 1988. Sampling in 1989 ended a month earlier than in 1988 because of early pruning. The orchard (study site) was harvested during the study and fruit yield data (kilograms per hectare) were provided by Guava Kai.
For the fogging treatment, two 3.66 by 7.32-m plastic tarps (Z. W. International, Pomona, CA) were placed under each tree, one to either side of the trunk so that there was an overlap of ≈2 cm. A solution of pyrethrins (3 ml Pyrenone Crop Spray per 1.5 liter H2O, Fairfield American Corporation, Newark, NJ.) was applied to each tree at the rate of 180 mg ([AI]) per tree with a Solo Port-423 Mist Blower (Solo, Sindelfingen, Germany), calibrated to deliver 1.5 liter in 2 min. This amount of spray gave complete coverage of treated trees with a quick knockdown of insects present in the canopy. Four min after application of spray, each tree was vigorously shaken to dislodge any remaining insects. The two tarps were removed immediately, and flies and parasitoids were placed into labeled plastic bags and identified at a later date. Sampling was conducted from 0730 to 1400 hours. For further details on the canopy fogging technique used and information on the oriental fruit fly parasitoid guild, sex ratios, and correlations with ripe fruit abundance, see Stark et al. 1991, 1994.
Spearman's rank coefficients were used to examine correlations between populations (oriental fruit fly, F. arisanus, and D. longicaudata) on different dates and proc univariate statistics calculated by species and sex (SAS Institute 2004). Significance (P=0.05) of means (H0: mean=0) was assessed with a t-test. Dispersion of oriental fruit fly, B. arisanus, and D. longicaudata was evaluated by the index of dispersion (I) calculated as variance to mean ratios (VMR=0, not dispersed; 0 < VMR < 1, underdispersed; and VMR > 1, overdispersed) (Southwood 1978). Distribution maps for oriental fruit fly, B. arisanus, and D. longicaudata were generated graphically by using Sigma Plot and Excel. Cross-correlations were generated with the R statistical language (R Development Core Team 2011) for analysis of time lags in number of males and females of each species. Finally, spatial correlations for each species were estimated by using a hurdle count regression model (Mullahy 1986). Hurdle models are two-component models, with a first step for determining zero versus nonzero numbers (the “hurdle”) and a second component built only on the nonzero portion of the data. The model tests if the mean numbers of conspecifics in adjoining blocks (those directly to the N, S, E, and W when all were available; subsets were used for blocks on the edges of the study site) are significantly related to the numbers in the central block after controlling for the changes in overall numbers over time. Significance indicates some level of spatial correlation, that is, the numbers of individuals in adjoining blocks are related to the numbers in the current block. More information on hurdle models is available in the article by Cameron and Trivedi (1998). Calculations for the hurdle model were conducted via the “pscl” library in R (Jackman 2011).
Results
Correlations Between Populations of the Different Species.
Populations of all three insects were never significantly (P=0.05) correlated together within a specific time interval (Fig. 1). However, there was a significant positive relationship (P < 0.05) between populations of oriental fruit fly and F. arisanus during each of the F. arisanus increases, but not between oriental fruit fly and D. longicaudata during the same periods. Both populations of parasitoids were significantly correlated with each other in July and November of 1988 and September of 1989. Populations of D. longicaudata and oriental fruit fly were significantly correlated (P < 0.05) once in August 1989.
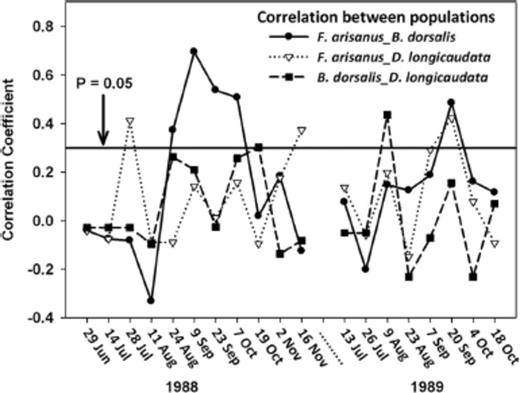
Correlation between populations of B. dorsalis, B. arisanus, and D. longicaudata in a commercial guava orchard during 1988 and 1989.
Population Dynamics of Male and Female Oriental Fruit Fly, F. arisanus, and D. longicaudata.
Population trends for the total number of oriental fruit fly, F. arisanus, and D. longicaudata of each sex collected during the two P. guajava seasons are shown in Fig. 2. During 1988, maximum fruit yield occurred on 23 September. Highest total numbers of oriental fruit fly (males and females), F. arisanus (males), and D. longicaudata (males and females) occurred 2 wk later on 7 October. During 1989, maximum fruit yield occurred on 20 September. Highest total numbers of oriental fruit fly (males and females), F. arisanus (males and females), and D. longicaudata (males and females) occurred on the same date.
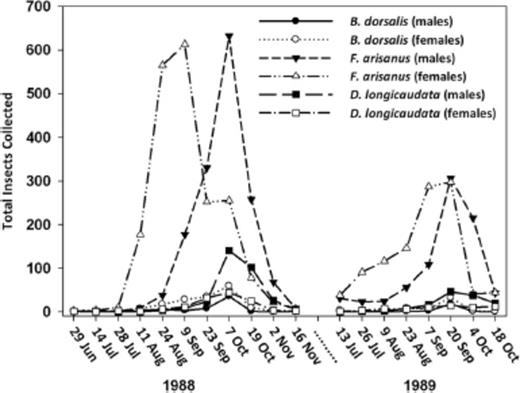
Total number of male and female B. dorsalis, B. arisanus, and D. longicaudata in a commercial guava orchard during 1988 and 1989.
Mean (±SD) numbers of oriental fruit fly, F. arisanus, and D. longicaudata by sex recovered from trees at ≈2-wk intervals at Guava Kai Orchards near Kilauea, HI, are given for 1988 and 1989 in Tables 1 and 2, respectively. Both parasitoid species colonized fields rapidly in response to ripening fruit and oriental fruit fly infestation. During June and July 1988 and July and August 1999, numbers of oriental fruit fly were low and very close to zero. Oriental fruit fly reached peaks of 1.00 ± 2.50 and 0.92 ± 1.13 males and 1.64 ± 2.50 and 0.50 ± 0.61 females during seasons 1988 and 1989, respectively. F. arisanus reached peaks of 17.56 ± 10.93 and 8.50 ± 11.46 males and 17.03 ± 14.65 and 8.25 ± 10.49 females during 1988 and 1989, respectively. D. longicaudata reached peaks of 3.89 ± 3.46 and 1.27 ± 1.73 males and 1.22 ± 1.17 and 0.39 ± 0.73 females during 1988 and 1989, respectively. Highest VMRs (indicating overdispersion) were obtained for F. arisanus, followed by D. longicaudata and oriental fruit fly.
Statistical tests on the occurrence (mean density) of B. dorsalis, F. arisanus, and D. longicaudata captured in sampling quadrats within an orchard on Kauai (June through November, 1988)
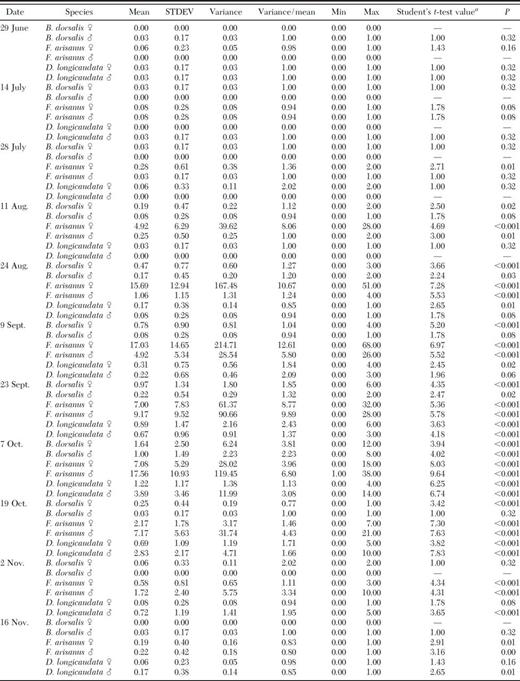
Significance of means (H0: mean = 0) was assessed with a Student's ttest at the P = 0.05 level (SAS 2004).
Statistical tests on the occurrence (mean density) of B. dorsalis, F. arisanus, and D. longicaudata captured in sampling quadrats within an orchard on Kauai (June through November, 1988)
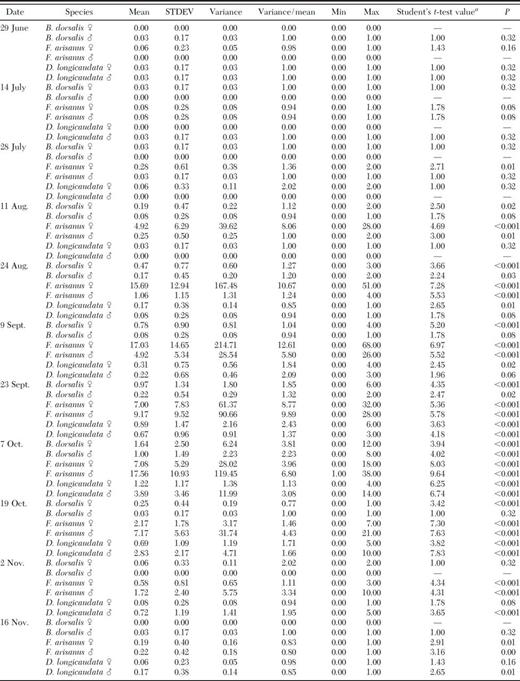
Significance of means (H0: mean = 0) was assessed with a Student's ttest at the P = 0.05 level (SAS 2004).
Statistical tests on the occurrence (mean density) of B. dorsalis, F. arisanus, and D. longicaudata captured in sampling quadrats within an orchard on Kauai (July through October, 1989)
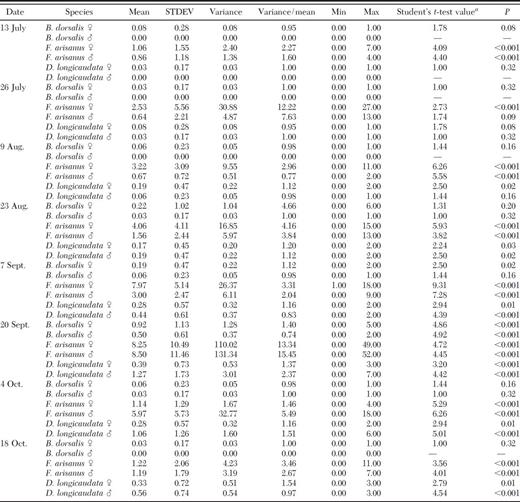
Significance of means (H0: mean = 0) was assessed with a Student's ttest at the P = 0.05 level (SAS 2004).
Statistical tests on the occurrence (mean density) of B. dorsalis, F. arisanus, and D. longicaudata captured in sampling quadrats within an orchard on Kauai (July through October, 1989)
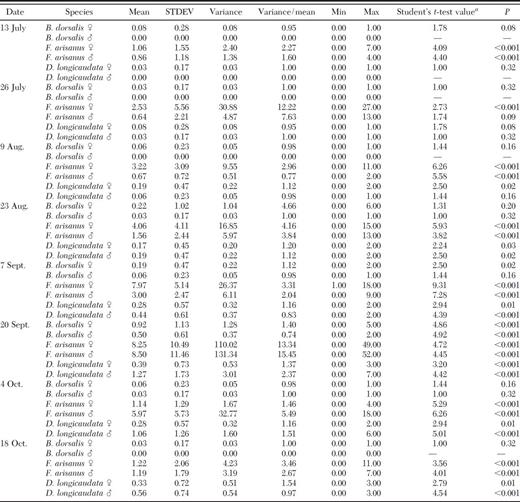
Significance of means (H0: mean = 0) was assessed with a Student's ttest at the P = 0.05 level (SAS 2004).
Correlations between Sexes of the Different Species.
Cross-correlation analysis (Fig. 3) suggested significant correlation between the number of oriental fruit fly males and females only when the lag between them was zero, so for this species, numbers of both sexes varied together in time. For F. arisanus in 1988, the highest significant cross-correlation occurred at a lag of +2 for the males, indicating that changes in the number of males were lagging changes occurring in the number of females by about 1 mo. A similar pattern was observed for F. arisanus in 1989, with the highest significant cross-correlation at a lag of +1 (=2 wk). There was some indication of a similar pattern in 1988 for D. longicaudata, but cross-correlation at a lag of +1 was similar to that at a lag of zero. These results are concordant with the abundance curves shown in Fig. 2.
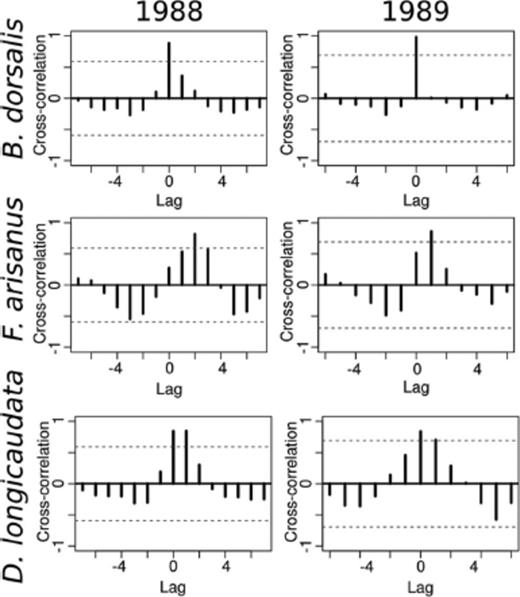
Cross-correlation of total number of male and female B. dorsalis, F. arisanus, and D. longicaudata for 1988 and 1989. Rows are species and columns are years, as labeled. The number of males over time is lagged relative to the number of females over the same period to find significant correlations that are temporally shifted. Broken lines indicate significance at P=0.05.
Distribution of Male and Female Oriental Fruit Fly, F. arisanus, and D. longicaudata.
Spatial distributions with time for oriental fruit fly, F. arisanus, and D. longicaudata by sex during 1988 and 1989 throughout a 36-block grid are summarized in Figs. 4F5F6F7F8 – 9. Oriental fruit fly females became highly aggregated (VMR > 1) from 24 August to 7 October during 1988 and from 23 August to 20 September during 1989. F. arisanus females became overdispersed sooner than D. longicaudata during both years. In addition, max VMRs [12.61 (9 September) versus 2.43 (23 September) in 1988; 13.34 (20 September) versus 1.54 (18 October) in 1989] were higher for F. arisanus females than those for D. longicaudata females. Similarly, VMRs [9.89 (23 September) versus 3.08 (2 October) in 1988; 15.45 (20 September) versus 2.37 (20 September) in 1989] were higher for F. arisanus males than those for D. longicaudata males.
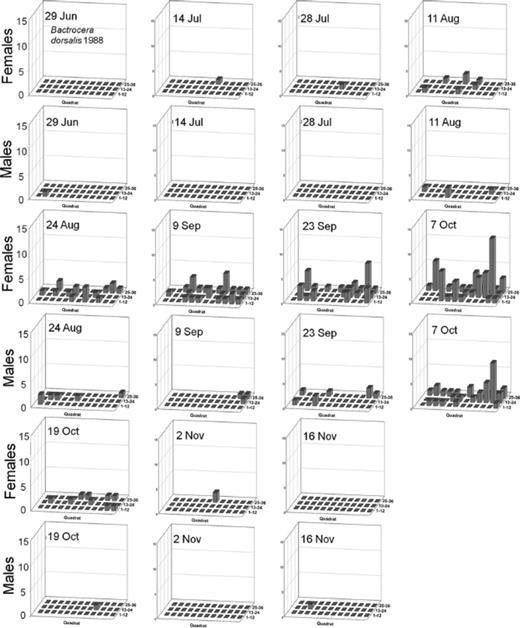
Spatial distribution patterns for female and male B. dorsalis in a commercial guava orchard during 1988.
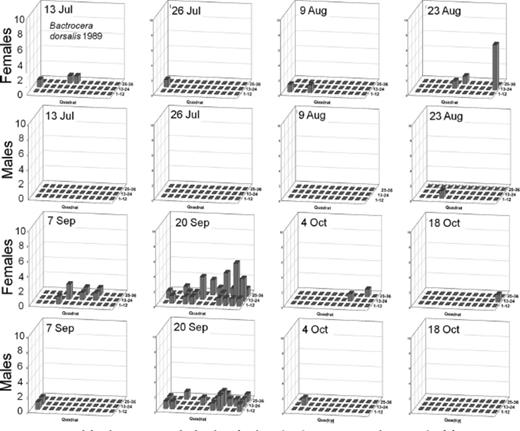
Spatial distribution patterns for female and male B. dorsalis in a commercial guava orchard during 1989.
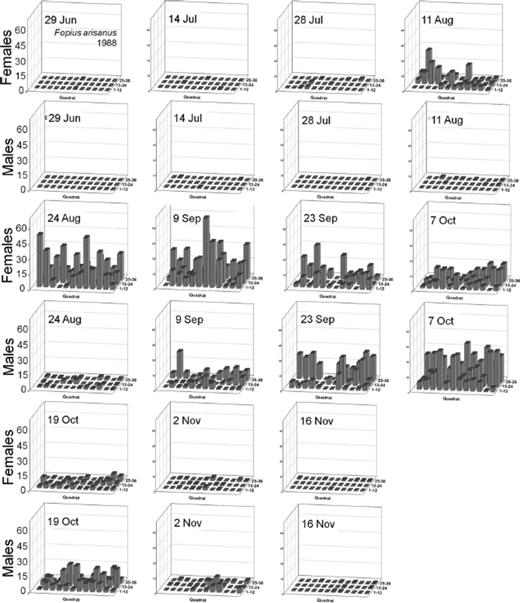
Spatial distribution patterns for female and male B. arisanus in a commercial guava orchard during 1988.
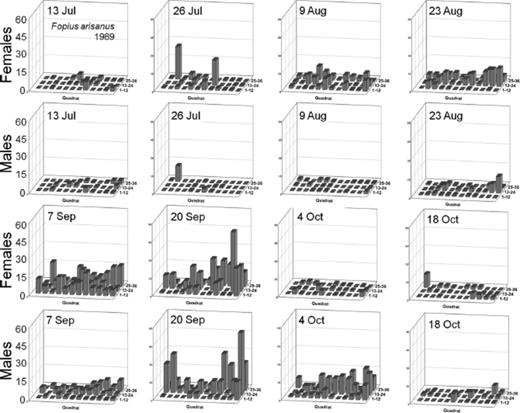
Spatial distribution patterns for female and male B. arisanus in a commercial guava orchard during 1989.
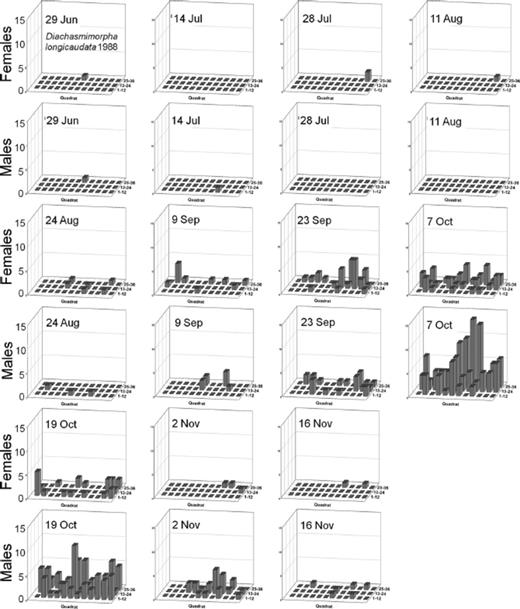
Spatial distribution patterns for female and male D. longicaudata in a commercial guava orchard during 1988.
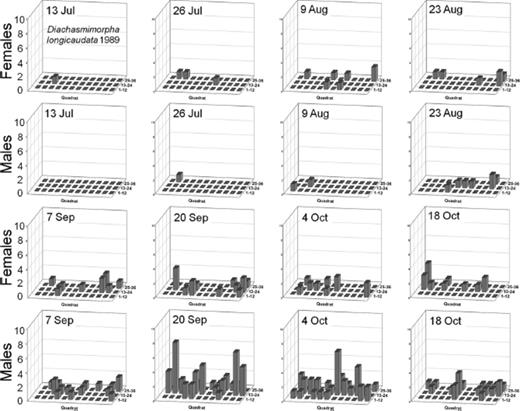
Spatial distribution patterns for female and male D. longicaudata in a commercial guava orchard during 1989.
To determine if there was evidence of spatial correlation in the number of each species, we fit a hurdle count regression model for each species separately. Results for F. arisanus are shown in Table 3; this was the only species that showed a significant correlation between the number of insects recovered in a given block and the average number of the same species in adjoining blocks after accounting for the total number of insects of that species in the whole study site (“mean number per block”). The relationship between the number in each block and the average number in the adjoining blocks for F. arisanus is shown graphically for both years (1988 and 1989) in Fig. 10.
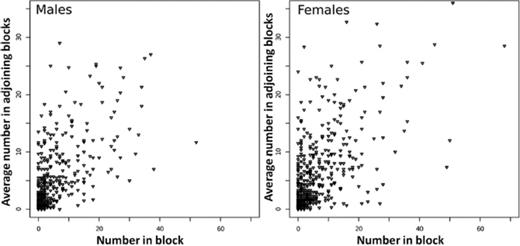
Number of F. arisanus per block plotted against the mean number in adjoining blocks for each sex.
Hurdle model of the number of F. arisanus per block as predicted by the mean number in adjoining blocks and the overall mean number per block (see text for further details)
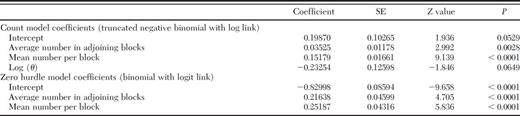
Hurdle model of the number of F. arisanus per block as predicted by the mean number in adjoining blocks and the overall mean number per block (see text for further details)
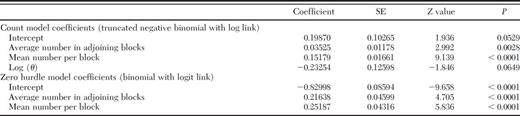
Discussion
Results of this study provide novel insights into the temporal and spatial dynamics of the two sexes of oriental fruit fly and two of its parasitoids as well as into the relationship between parasitoid and host. Such analytical detail for field data has not been previously reported, and it should help inform decision-makers choosing between various options when seeking the best strategy to combat the spread of oriental fruit fly and related pest species in various parts of the world through biological control releases.
Particularly noteworthy was the rapid response by F. arisanus to population changes by oriental fruit fly (Fig. 1). The increases by D. longicaudata, at a later time in the season, were not unexpected and most likely were driven by large amounts of ground fruit with mature larvae just before harvest. Changes in total numbers of male and female oriental fruit fly and D. longicaudata were synchronous, but for F. arisanus, we found a significant lag in the density curves, with females leading males by 2–4 wk. One possible explanation is that because of the high correlation between oriental fruit fly and F. arisanus increases, F. arisanus females were tracking oriental fruit fly females ovipositing in guava fruits. Subsequently, F. arisanus males were attracted to the presence of large numbers of females in trees.
Of the three species studied, only F. arisanus showed a significant positive relationship between the number found in a given block and the mean number in adjoining blocks in terms of both presence (P < 0.0001) and number (P=0.0028) after correcting for changes in the total number of this species (Table 3). This suggests a measurable degree of spatial correlation and thus within-study site spatial structure (correlations in the number of individuals over space). This structure may be the result of less movement throughout the field by F. arisanus compared with the other two species. One other reason we may not have detected spatial structure in oriental fruit fly and D. longicaudata populations may be that their numbers were overall lower than those of F. arisanus, so we may have lacked statistical power to find spatial structure.
Dispersion patterns of oriental fruit fly and its two most abundant parasitoids, F. arisanus and D. longicaudata, in the commercial orchard were initially random, but became highly dispersed with the ripening of P. guajava (for oriental fruit fly) and the subsequent presence of, first, large number of host eggs (for F. arisanus) and, second, host larvae (for D. longicaudata) in P. guajava fruits.
The large change in the numbers of fruit flies and parasitoids over each of the two study years is a feature previously observed and described. In the case of fruit flies, Vargas et al. (1990) documented a reciprocal relationship between host ecology and spatial distribution of fruit flies throughout a large agricultural area, whereby oriental fruit fly abundance increased in agricultural areas with the fruiting of wild strawberry guava, Psidium cattleianum Sabine, and P. guajava (common guava) in surrounding areas. Regarding parasitoids, Stark et al. (1991) showed a similar pattern to the current study area not only with canopy fogging but also with fruit collections, with the highest numbers in the orchard coinciding with highest numbers in surrounding areas. Thus, the rapid increase in colonization of cultivated guava trees would suggest that flies and parasitoids moved from wild areas into cultivated areas.
To understand how the results from this study might be useful in planning future biological control releases, it is helpful to revisit efforts to control oriental fruit fly by using F. arisanus in French Polynesia over the past decade. Oriental fruit fly invaded French Polynesia in 1996 (Leblanc and Putoa 2000). Data from the present spatial study and related ecological studies (Stark et al. 1991, 1994; Vargas et al. 1993) on parasitoid establishment in Hawaii were critically examined before biological control releases were attempted in French Polynesia. Key strategies included releasing F. arisanus in large numbers near host trees around fruiting time, especially targeting stands of P. guajava. Releases began in 2002, and continued until 10 parasitoid shipments from Hawaii, >5,00,000 insects, were dispersed in 2 major locations and 10 minor locations around Tahiti Island. F. arisanus was established throughout all 21 communities within 3 yr on Tahiti Island and nearby Moorea Island (Vargas et al. 2007). Furthermore, F. arisanus became so abundant in P. guajava fruits that recovery of F. arisanus from wild P. guajava fruits was used to establish it on the Society Islands of Huahine, Tahaa, and Raiatea, where oriental fruit fly had spread (Vargas et al. 2007). By 2009, mean (±SD) F. arisanus parasitism for fruit flies infesting P. guajava, Inocarpus fagifer (Parkinson) Fosberg (Polynesian chestnut), and Terminalia catappa L. (tropical almond) fruits on Tahiti Island was 64.8 ± 2.0% (Vargas et al. 2012a, b). Noteworthy was that F. arisanus was most effective in hosts that were present throughout the year (P. guajava, I. fagifer, and T. catappa) and not as effective in hosts with fruits present for only a few months (i.e., mango, Mangifera indica L.) Subsequently, five shipments of D. longicaudata (of ≈5,000 each for 10,000 surviving wasps) were made between September 2007 and 2008 on Tahiti. Although it became equally widespread, parasitism rates have not been higher than 10% (Vargas et al. 2012a, b), in agreement with observations in the current study. From 2002 to 2009, based on numbers of oriental fruit fly, B. tryoni (Froggatt), Queensland fruit fly, and B. kirki (Froggatt) emerging (per kilograms of fruit), there has been a decline in fruit flies emerging from P. guajava of 92.3, 96.8, and 99.6%, respectively (Vargas et al. 2012a). F. arisanus was reared from Queensland fruit fly, but the exact impact on B. kirki is unknown. Nonetheless, establishment of F. arisanus in French Polynesia is the most successful example of classical biological control of fruit flies in the Pacific outside of Hawaii and can be introduced if oriental fruit fly spreads to other French Polynesian islands.
Recent experience in French Polynesia and spatial data presented here support F. arisanus as a prime biological control candidate for introduction into South America and Africa, where B. carambolae and B. invadens, respectively, have become established. Releasing F. arisanus in a continental setting brings some new challenges, especially in making certain that sufficient numbers of wasps are released so the population becomes self-sustaining. Based on the results described here, if there is already an established Bactrocera population, parasitoid releases could be initiated before host fruit ripen completely, increasing the amount of time available for large numbers to be reared, moved to the field, and released. We would also expect a steady increase in the size of the area parasitized by F. arisanus between years, as observed in Tahiti and other islands in French Polynesia. For future research, we note that although the biological control system observed in the commercial orchard on Kauai is a sustainable system, it may be important to test if augmentative releases during certain times of the year when parasitoids are not abundant could improve levels of field parasitism and significantly decrease damage to fruits.
We are grateful to Neil Miller, Steven Souder, and Brian Fujita (United States Department of Agriculture [USDA], Agricultural Research Service [ARS], United States Pacific Basin Agricultural Research [USPBARC], Hilo, HI) for their assistance with this research and manuscript. We also appreciate the technical assistance in French Polynesia of Rudolph Putoa, Axelle Maitere, Berthe Neagle, Djeeen Cheou, Jules Wohler, and Jean-Marc Tinirau, Service du Développement Rural, Département de la Protection des Végétaux, Papeete, Tahiti. Special thanks to Danny Seo, Michael McKenney, Thomas Mangine, and Keith Shigeteni for their work in the insectary, essential to this work. We thank anonymous reviewers for reviewing earlier drafts of this manuscript. Finally, without USDA, Foreign Agricultural Service and USDA, ARS, Area-Wide Pest Management funds, this work could not have been accomplished.
References
Author notes
This article reports the results of research only. Mention of proprietary product does not constitute an endorsement or recommendation by the U.S. Department of Agriculture (USDA).