-
PDF
- Split View
-
Views
-
Cite
Cite
Ma. Guadalupe Mendoza, Yolanda Salinas-Moreno, Antonio Olivo-Martínez, Gerardo Zúñiga, Factors Influencing the Geographical Distribution of Dendroctonus rhizophagus (Coleoptera: Curculionidae: Scolytinae) in the Sierra Madre Occidental, México, Environmental Entomology, Volume 40, Issue 3, 1 June 2011, Pages 549–559, https://doi.org/10.1603/EN10059
- Share Icon Share
Abstract
The bark beetle, Dendroctonus rhizophagus Thomas & Bright, is endemic to the Sierra Madre Occidental (SMOC) in México. This bark beetle is a major pest of the seedlings and young saplings of several pine species that are of prime importance to the nation's forest industry. Despite the significance of this bark beetle as a pest, its biology, ecology, and distribution are poorly known. Three predictive modeling approaches were used as a first approximation to identify bioclimatic variables related to the presence of D. rhizophagus in the SMOC and to obtain maps of its potential distribution within the SMOC, which is a morphotectonic province. Our results suggest that the bark beetle could have an almost continuous distribution throughout the major mountain ranges of the SMOC. This beetle has a relatively narrow ecological niche with respect to some temperature and precipitation variables and inhabits areas with climatic conditions that are unique from those usually prevalent in the SMOC. However, the bark beetle has a broad ecological niche with respect to the number of hosts that it attacks. At the macro-scale level, the D. rhizophagus distribution occurs within the wider distribution of its main hosts. The limit of the geographical distribution of this bark beetle coincides with the maximum temperature isotherms. Our results imply a preference for temperate habitats, which leads to the hypothesis that even minor changes in climate may have significant effects on its distribution and abundance.
Both environment and population ecology processes limit the abundance and geographic distribution of species (Brown 1984, Thomas et al. 2001, Lomolino et al. 2006). Traditionally, distribution studies have used only basic information (location and elevation) associated with specimens from museums and scientific collections (MacDonald 2003). Integrating this basic information with additional data on climate, biology, and topography is essential to fully understand the distributional ranges of species, the potential for distributions to change, and the variables that limit or favor range expansion or contraction (Gaston 2003).
The use of geographic information systems (GIS) and the development of a broad spectrum of spatial modeling approaches, including bioclimatic analysis (BIOCLIM) (Nix 1986), Ecological Niche Factor Analysis (ENFA) (Hirzel et al. 2002), and Maximum Entropy (MaxEnt) (Phillips et al. 2004), have made possible the integration of diverse data on biology, climate, and topography. This has led to the development of more reliable distribution maps and the modeling of species responses to different past and future climatic conditions. BIOCLIM, in particular, has been used to predict the climate domain and potential species response to climate change at the meso-scale level (Mackey and Lindenmayer 2001, Ganeshaiah et al. 2003, Jiménez-Valverde et al. 2007). ENFA and MaxEnt have been used to characterize ecological niches and to determine suitable areas for species conservation and natural resource management (Braunisch and Suchant 2007, Sattler et al. 2007, Titeux et al. 2007, Kumar and Stohlgren 2009). Frequent use of these tools has shown their usefulness and reliability in analyzing the potential distribution of species that have a relatively wide distribution range and whose biology and ecology are poorly known (Pearson and Dawson 2003, Beaumont et al. 2005).
Bark and ambrosia beetles (Coleoptera: Curculionidae: Scolytinae) are important natural components of forest ecosystems. The infestation of weakened mature trees promotes the presence of different successional stages and demographic structures within the forest. Nevertheless, these insects are a worldwide problem for the forest industry and in public parks and natural reserves because of the extensive tree mortality they cause under outbreak conditions and as vectors of diseases that reduce tree vigor (Wood 1982). Moreover, some bark beetles are important invaders that represent a significant threat to the health of forests (Brockerhoff et al. 2006). Specifically, bark beetles of the genus Dendroctonus Erichson have a wide geographic distribution in North and Central America. They have caused significant economic losses and irreversible ecological changes in Mexican forests (Cibrián et al. 1995).
The development of Dendroctonus species usually takes place on mature trees (>15 yr) of the genera Larix Mill., Picea A. Dietrich, Pinus L., and Pseudotsuga Carr. (Wood 1982). The advanced age classes of these trees constitute an adequate resource for bark beetle nourishment and reproduction. The sole exception to this is Dendroctonus rhizophagus Thomas and Bright, a species that is endemic to the Sierra Madre Occidental (SMOC) mountain range in northwestern México. D. rhizophagus colonizes and kills the seedlings and young saplings (<10 yr) of various pine species, especially Apache pine (Pinus engelmannii Carr.), Durango pine (Pinus durangensis Martinez), Chihuahua pine (Pinus leiophylla Schlecht and Cham.), and Arizona pine (Pinus arizonica Engelm.) (Cibrián et al. 1995, Salinas-Moreno et al. 2004, Sánchez-Martínez and Wagner 2009). This unique bark beetle is a major threat to regeneration in pine forests throughout its distribution in México (Sánchez-Martínez and Wagner 2009).
Although D. rhizophagus infests a significant number of seedlings and young saplings every year, its biology, ecology, and geographic distribution are poorly known (Estrada-Murrieta 1983, Salinas-Moreno et al. 2004, Sánchez-Martínez and Wagner 2009). Thus, analyzing its geographic distribution is essential to identify the environmental variables associated with its presence in northwestern México and to evaluate its potential threats in other geographical areas of México. Furthermore, if we assume that ongoing climate change could affect the frequency and intensity of bark beetle outbreaks and the geographic distribution of these insects and their hosts (Logan and Powell 2001, Kurz et al. 2008, Waring et al. 2009), there is a possibility that D. rhizophagus could migrate north into forests of the southwestern United States and cause widespread tree mortality in these large contiguous forest. Thus, the purpose of this study is to model the potential distribution of D. rhizophagus in the Sierra Madre Occidental of northwestern México by using BIOCLIM, ENFA, and MaxEnt to describe the bioclimatic conditions where it occurs and to identify variables that determine its presence.
Materials and Methods
Biological Data.
Collection records (museum specimens) for D. rhizophagus were obtained from the major entomological collections in México and from technical reports of the Comisión Nacional Forestal (CONAFOR) and the Secretaría de Medio Ambiente y Recursos Naturales (SEMARNAT) for the states of Chihuahua, Durango, Sonora, and Sinaloa (Table 1). In addition, we included records gathered in the course of several field studies conducted from 2000 to 2008. Although some areas of the SMOC morphotectonic province have been explored little, these records represent a reliable sample of the geographical distribution of D. rhizophagus. A morphotectonic province is a zone having distinctive enough geomorphic and geologic or tectonic features to differentiate it from neighboring zones (Ferrusquía-Villafranca 1998).
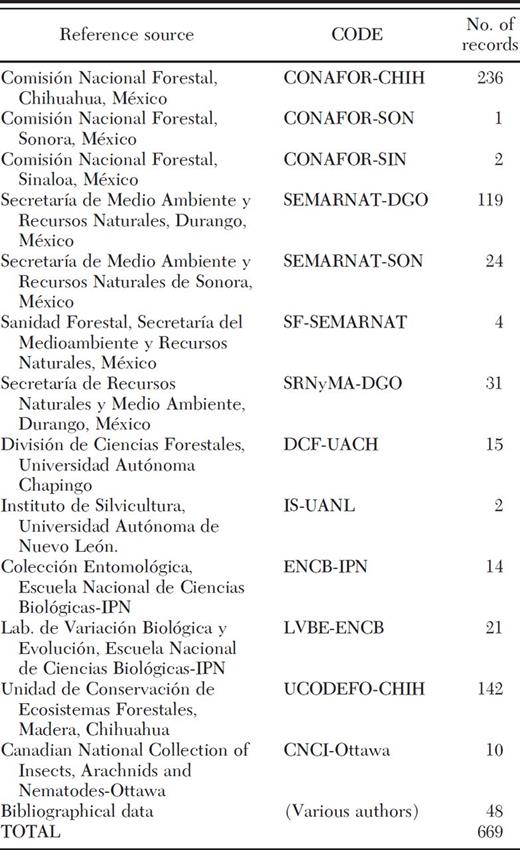
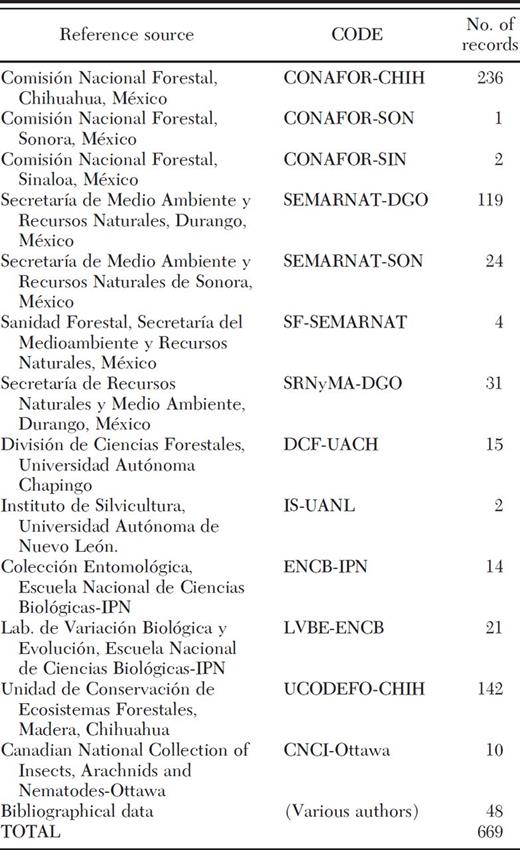
A preliminary database of 669 records was assembled to determine the altitudinal range where D. rhizophagus has been found most frequently and the frequency of its incidence (IP) on different hosts. The IP is a measure of the degree of occurrence of the bark beetle on its host plants (Salinas-Moreno et al. 2004). All location records were georeferenced on 1:50,000 topographic maps obtained from the INEGI. For the potential distribution analysis, 412 records were eliminated from the database, because they differed only minimally in geographic coordinates and elevation. Thus, the spatial modeling database was composed of 257 presence-only records.
Similarly, a database of the principal hosts of D. rhizophagus was assembled from specimens stored in the national herbariums of México and online records from the webpage of the Comisión Nacional para el Conocimiento y uso de la de Biodiversidad (CONABIO). The final database included 294 presence-only records of 11 pine species. These records were georeferenced on 1:50,000 INEGI topographic maps.
Study Area.
The morphotectonic province of the Sierra Madre Occidental (SMOC) is located between 20° 30′ and 31° 20′ N and between 102° 20′ and 109° 40′ W. It covers 289,000 km2 and runs northwest to southeast. Elevations in this mountain chain range from 200 m to slightly above 3,000 m. The climate is temperate, and the vegetation type is primarily pine forest and, to a lesser extent, oak and pine-oak forest. The mean annual precipitation ranges from 400 to 1,600 mm (Rzedowsky 1978, Ferrusquía-Villafranca 1998).
Potential Geographic Distribution of D. rhizophagus by using BIOCLIM.
A bioclimatic profile of D. rhizophagus was obtained from BIOCLIM in the DIVA-GIS v.5.2 software package (Hijmans et al. 2002) by using 19 default temperature and precipitation variables included in BIOCLIM (Table 2). This profile describes the environment where the bark beetle has been recorded, and it is used to identify other sites where the species may reside. The suitability limits of the species are characterized by the mean, standard deviation, minimum and maximum tolerance, and percentiles of each variable to a resolution of 2.5-arc min.
Bioclimatic profile of D. rhizophagus for each location (obtained using BIOCLIM)
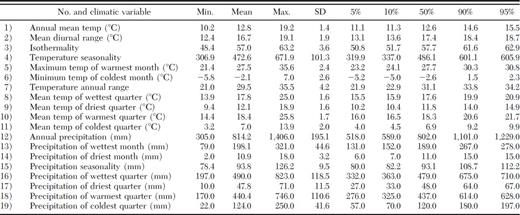
Bioclimatic profile of D. rhizophagus for each location (obtained using BIOCLIM)
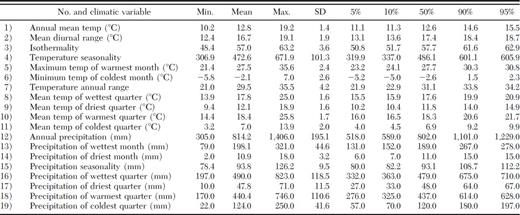
A principal component analysis (PCA) of these climatic variables was performed with STATISTICS v.7.0 to assess the relative contribution of each variable to the bioclimatic profile of the species. In addition, histograms were developed to determine the statistical distribution of these climatic variables. Variables that follow a normal distribution or a skewed distribution exert greater influences on the bioclimatic profile (Beaumont et al. 2005). Thus, those variables without a clearly defined distribution or a normal distribution with a truncated histogram were eliminated. Subsequently, grid cells that included the bioclimatic profile of D. rhizophagus were identified using BIOCLIM. Grid cells were grouped into four categories: null (areas outside the 0–100 percentile limits), low (areas within the 0–2.5 percentile limits), moderate to high (areas within 2.5–10 percentile limits), and very high to excellent (areas within the 10–100 percentile limits). The scatter of cells associated with these categories represents the potential distribution of the species.
Potential Geographic Distribution of D. rhizophagus by using ENFA.
Maps showing the major climatic variables (determined by PCA), minimum annual temperature, maximum annual temperature, and elevation were plotted with DIVA-GIS v.5.2. In addition, BIOCLIM was used to draw a map of the potential distribution of the hosts of D. rhizophagus to incorporate this variable into the analysis.
All maps were imported into ArcView v.3.2 (Environmental Systems Research Institute 1999) and were transformed to the Idrisi format. Subsequent analyses were conducted with the Ecological Niche Factor Analysis (ENFA) algorithm available in BIOMAPPER v.3.2 (Hirzel et al. 2006a). ENFA conducts a PCA of ecogeographic variables and generates a series of internally uncorrelated factors that are used to plot potential habitat or habitat suitability (HS) maps. Only significant factors were considered for the HS map. Biologically significant factors included marginality and tolerance. Marginality is defined as the difference between the optimum environment for the species and the global mean of environmental variables for the study area. Tolerance indicates the degree of specialization of the species in relation to the range of available environments (Hirzel et al. 2002, 2004). A habitat suitability score (0–100) was derived for each grid cell by comparing its marginality and specialization coefficients with the median of each factor estimated for the study area. Specialization coefficients are defined as the ratio of the ecological variance in mean habitat to that observed for the focal species (Hirzel et al. 2002). HS scores were normalized into four categories: null to low (0–25), moderate (26–50), high (51–75), and very high to excellent (76–100).
The robustness and predictive ability of the HS model were evaluated in BIOMAPPER by a jackknife-type cross-validation by using k=4 partitions of the records of the species based on Huberty's rule, which is a heuristic method (“rule of thumb”) that determines the ratio of calibration and validation points (Fielding and Bell 1997). Three of the generated partitions were used to calibrate the HS map, and the fourth was used to evaluate the outcome. The process was replicated four times, and each group was used to validate the model for each replicate by using Boyce's continuous index. This index measures the relationship between the expected and observed values for different HS scores (Hirzel et al. 2006b). A value near one indicates congruence between the expected and observed values.
Potential Geographic Distribution of D. rhizophagus by using MaxEnt.
Maps of the major climatic variables obtained from DIVA-GIS v.5.2, determined with the aid of PCA and histograms, plus maps of minimum annual temperature, maximum annual temperature, elevation and host distribution, were analyzed with MaxEnt v.3.3.1 (Phillips et al. 2004). The MaxEnt algorithm was performed using the default parameters (500 iterations with a convergence threshold of 10−5). During model development, 75% of the localities were used for model training, whereas 25% of the localities were held back to test the model. Suitable regularization values were included to reduce over-fitting and were selected automatically by the program. The output format was the logistic default option (Phillips and Dudík 2008). The model was evaluated by means of the area under the receiver operating characteristic curve (AUC). AUCs near 0.5 are similar to random predictions, which indicate an inaccurate model; in contrast, values above 0.9 indicate a highly accurate model (Swets 1988, Phillips and Dudík 2008). The resultant map showed the probability (0–1) of species presence. Probability values were normalized into four categories: null to low (0–0.22), moderate (0.23–0.44), high (0.45–0.67), and very high to excellent (0.68–1.0).
Results
The preferential altitudinal range for D. rhizophagus varied between 2,000 and 2,600 m. The percentage of incidence showed a high frequency of attacks by this beetle on P. arizonica, P. durangensis, P. engelmannii, and P. leiophylla (Table 3).
Incidence (%) of D rhizophagus on Pinus species in the Sierra Madre Occidental, México

Incidence (%) of D rhizophagus on Pinus species in the Sierra Madre Occidental, México

Bioclimatic Profile of D. rhizophagus.
The profile based on 257 locations (Table 2) suggests that this bark beetle may be present in sites supporting the following temperatures: annual mean temperatures of 10–19°C, maximum temperatures of the warmest month of 21–36°C, mean temperatures of the driest quarter of 9–19°C, and mean temperatures of the coldest quarter of 3–14°C. In terms of precipitation, suitable sites are characterized by annual rainfalls of 305–1,406 mm, wettest-month rainfalls of 79–321 mm, driest-month rainfalls of 2–18 mm, wettest-quarter rainfalls of 197–823 mm, and warmest-quarter rainfalls of 170–746 mm.
PCA showed that the first three principal components accounted for 86.79% of the total variance (Table 4). Of the 19 variables that were analyzed, 13 had high eigenvalues. However, of these 13 variables, only precipitation variables 12, 13, 14, 16, and 18 and temperature variables 1, 5, 9, and 11 were normally distributed. Temperature variables 2 and 7 had normal but truncated distributions, whereas 3 and 4 did not fit any distribution. These last four variables were not used to generate potential distribution models with BIOCLIM, ENFA, or MaxEnt.
Principal component analysis of BIOCLIM climatic variables in relation to the occurrence of D. rhizophagus
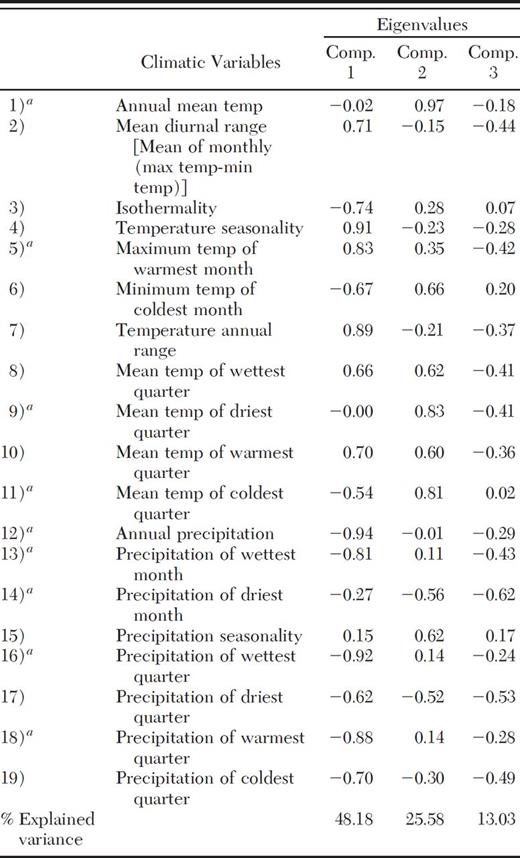
Most relevant variables according to histograms analysis.
Principal component analysis of BIOCLIM climatic variables in relation to the occurrence of D. rhizophagus
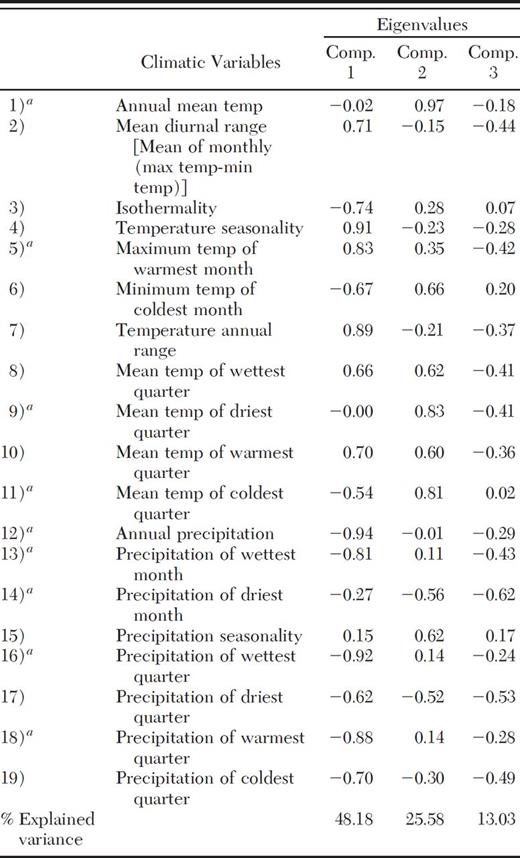
Most relevant variables according to histograms analysis.
Potential Geographic Distribution of D. rhizophagus by using BIOCLIM, ENFA, and MaxEnt.
The potential distribution map obtained from BIOCLIM showed a continuous area of moderate to high climate suitability that encompassed the entire length of the SMOC morphotectonic province, as well as a narrower, continuous area with very high to excellent values (Fig. 1). The ENFA map showed a potential distribution that was similar to the distribution derived by BIOCLIM but it had better definition (Fig. 2). The high and very high to excellent HS areas on the ENFA map appeared as small, discontinuous patches scattered along the entire length of the SMOC morphotectonic province. Areas of moderate suitability occurred in the gaps between these patches. The map generated with MaxEnt (Fig. 3) showed two separate, large areas of very high to excellent probability of occurrence in the northern part of the SMOC and some small, discontinuous areas in its central and southern regions. Moderate and high probability areas were found in almost all the SMOC.
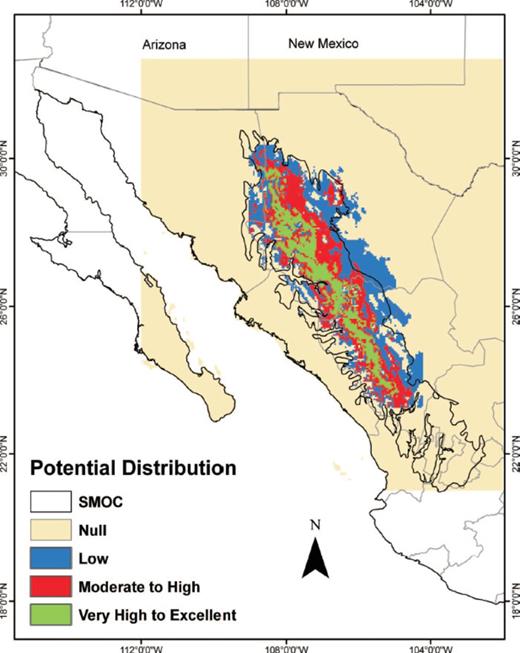
Potential distribution of D. rhizophagus in the Sierra Madre Occidental, México modeled with BIOCLIM.
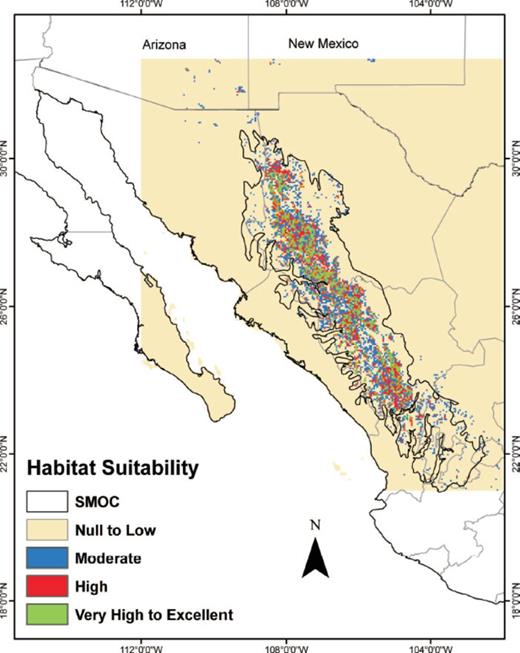
Habitat suitability (HS) for D. rhizophagus in the Sierra Madre Occidental, México modeled with ENFA.
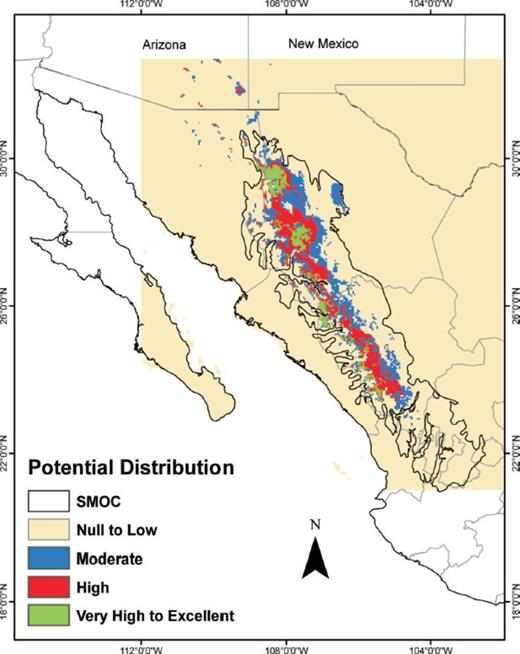
Potential distribution of D. rhizophagus in the Sierra Madre Occidental, México modeled with MaxEnt.
The global marginality factor was 2.76, suggesting that D. rhizophagus is present in areas supporting bioclimatic conditions that differ from the average conditions in the SMOC. Global tolerance was 0.17, indicating that this species has a relatively narrow ecological niche. The first three factors explained 93.4% of the total variation (Table 5). The marginality factor (number 1) explained only 4.7%, and the first two tolerance factors (numbers 2 and 3) explained 81.4% and 7.3%, respectively.
Percentage of variation explained by ecogeographic variables for D. rhizophagus by using ENFA
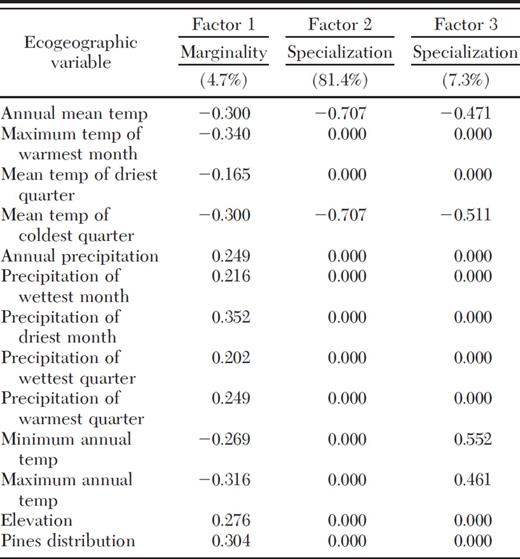
Percentage of variation explained by ecogeographic variables for D. rhizophagus by using ENFA
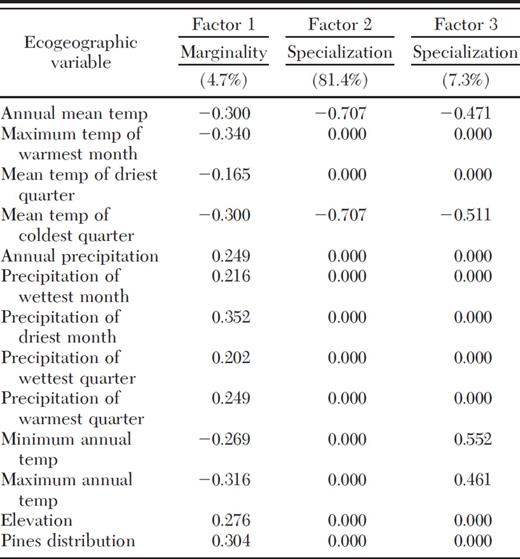
In terms of the marginality factor (Table 5), D. rhizophagus is essentially linked to the precipitation of the driest month (0.352) and the following temperature variables: annual maximum temperature (−0.316), maximum temperature of the warmest month (−0.340), mean temperature of the coldest quarter (−0.300), annual mean temperature (−0.300), and annual minimum temperature (0.269). In addition, D. rhizophagus is linked to host distribution (0.304) and elevation (0.276). Tolerance factors indicate that D. rhizophagus is associated with annual mean temperature (−0.707), mean temperature of the coldest quarter (−0.707), annual minimum temperature (0.552), and annual maximum temperature (0.461). Jackknife-type cross-validation and evaluation by using Boyce's continuous index yielded a mean of 0.67 and a standard deviation of 0.32, both of which indicated the model is adequately robust.
MaxEnt analysis showed that the presence of D. rhizophagus is determined mainly by annual mean temperature (30.1%), elevation (13.4%), maximum annual temperature (12.8%), precipitation of the warmest quarter (10.5%), precipitation of the driest month (9.8%), maximum temperature of the warmest month (8.1%), and precipitation of the wettest month (5.1%) (Table 6). The AUC of the training data was 0.976 (±0.006), and the AUC of test points was 0.970 (±0.006), indicating the model is robust.
Percentage of estimated contribution for ecogeographic variables by using MaxEnt
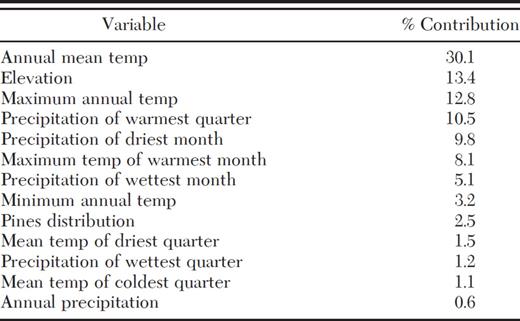
Percentage of estimated contribution for ecogeographic variables by using MaxEnt
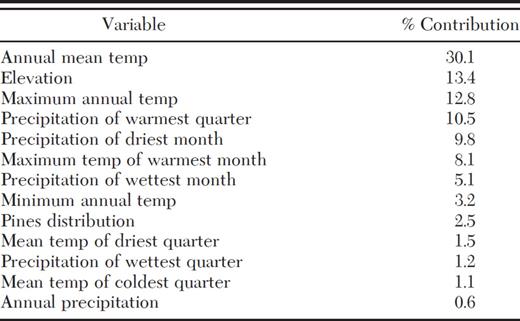
Discussion
The spatial modeling of species distributions combining occurrence data with biotic, climatic, and topographic variables can be used to determine the bioclimatic profile of a species and, as a consequence, the potential ranges in which it may reside (Anderson et al. 2003, Beaumont et al. 2005). This information provides an initial explanation for the presence of a species in one area but not in another (Lindenmayer et al. 1991). For herbivorous insects, the host is an extremely important limiting factor in terms of geographical distribution, as insects cannot continue to occur in those places where their host plants are not present (Spellerberg and Sawyer 1999, Lomolino et al. 2006).
Although various studies suggest that the geographic distribution of bark beetles at the macro-scale level is determined by variables such as host availability, temperature, and elevation (Amman 1973, Lekander et al. 1977, Régnière and Logan 1996), few studies have examined the relative importance of these variables (Ungerer et al. 1999, Carroll et al. 2004). With respect to the host, D. rhizophagus has a narrow diet breadth, because it uses a small number (≤40%) of the available pine hosts across its distribution (Kelley and Farrell 1998). However, the results of our study indicate that D. rhizophagus attacks almost all (>80%) species of pine present in its known distribution which is limited to specific areas within the SMOC, especially P. arizonica, P. engelmannii, P. leiophylla, and P. durangensis (Wood 1982, Salinas-Moreno et al. 2010). The major incidence percentages (IPs) on these four pine species do not appear to be related to the abundance of them in the SMOC, as all of the hosts used by this bark beetle are similarly abundant elements in the pine forests of this morphotectonic province (Rzedowsky 1978, Perry 1991, García Arevalo and González Elizondo 2003, Ortega-Rosas et al. 2008). However, the observed IP values could be related to the ecologically heterogeneous or patch distribution that these species have in the SMOC morphotectonic province. For example, while Pinus herrerae and P. leiophylla inhabit slopes and ravines, P. arizonica and P. durangensis grow on plateaus and mesas.
However, our study also suggests that the distribution of D. rhizophagus may not be limited by the geographical distributions of its hosts (Table 6), as there are areas in the SMOC morphotectonic province where hosts are present but where there are no records of the beetles presence. In addition, the geographical distributions of the majority of them (≥90%) extend over much of the range of this bark beetle; for example, P. cembroides Zucc., P. herrerae Martínez, P. leiophylla, P. lumholtzii Rob. & Fernald, P. oocarpa Schiede, and P. teocote Schiede ex Schltdl. & Cham. are present in other morphotectonic provinces in México, and others such as P. cembroides, P. engelmannii, P. leiophylla, P. ponderosa Dougl., and P. strobiformis Engelm. are located beyond the Mexican border and occur in the southwestern United States (Farjon and Styles 1997). Differences in the geographic distributions of D. rhizophagus and its hosts might be the result of their natural history, where the specific biotic interactions and particular ecological requirements of each of them have played important roles.
Based on these results and those derived with ENFA and MaxEnt (Tables 5, 6), which suggest that the host distribution has little weight as a predictor variable, we hypothesize that at the macro-scale level the potential distribution of D. rhizophagus is limited more by environmental variables such as precipitation and temperature than by the availability or distribution of hosts (Lekander et al.1977). In this context, the tolerance and marginality coefficients derived from ENFA suggest that this beetle has a relatively narrow ecological niche with respect to some temperature and precipitation variables, inhabiting areas with climatic conditions that differ from those generally prevalent in the SMOC morphotectonic province. Areas of higher suitability may therefore be smaller and may appear in patches along the SMOC, as predicted by ENFA, compared with those predicted by BIOCLIM.
The influence of elevation on the distribution of this beetle is not clear: although MaxEnt (Table 6) suggests that this factor is important, ENFA (Table 5) suggests the contrary. The relationship between temperature and elevation could be masking the effect of this factor on the altitudinal distribution of D. rhizophagus. The broad elevation range of this species (1,000–2,800 m), matches with the altitudinal range in which pine and pine-oak communities are found in the SMOC. In addition, the preferential elevation range (2,000–2,600 m) of D. rhizophagus is correlated with the preferential altitudinal range of its main hosts (Farjon and Styles 1997, Lammertink et al. 1997) and represents areas identified by BIOCLIM, ENFA, and MaxEnt as high to excellent habitats for this bark beetle.
The actual distribution limits of D. rhizophagus into the north and south of the SMOC coincides with the isotherms for maximum temperature occurring between 30 and 23° N of its distribution range. Specifically, these isotherms are annual temperatures of 20–25°C and temperatures of the warmest month of 23–30°C. Other bark beetle studies conducted at the macro-scale level report similar associations with climatic variables. For example, the distribution of the mountain pine beetle, Dendroctonu s ponderosae Hopkins, in western Canada is limited by minimum temperatures, rather than by the availability of its preferred hosts (ponderosa pine, P. ponderosa), despite their wide geographic distribution (Logan and Powell 2001). Therefore, its distribution is limited in areas further north (Yukon and Northwest Territories) and east (Alberta) in Canada (Carroll et al. 2004). Ungerer et al. (1999) reported that the isotherm for minimum annual temperature (−16°C) limits the dispersal of D. frontalis Zimm. into more northerly areas of the United States.
Our study suggests that D. rhizophagus could potentially be distributed over a greater extent of the SMOC morphotectonic province. This will occur as a result of both the use of a wide range of pine hosts present in this province and the presence of numerous areas in the SMOC where optimal conditions of elevation, temperature, and humidity exist. Moreover, our results suggest that limits of the D. rhizophagus distribution at the macro-scale level could be related to the maximum temperature isotherms and that optimal temperature ranges where the bark beetle occurs are related to temperate habitats. Finally, its relatively narrow ecological niche with respect to some temperature and precipitation variables and the preference for temperate habitats, lead us to hypothesize that even minor changes in climate may have significant effects on the distribution and abundance of this bark beetle. The apparent absence of this species from potentially suitable habitats within the SMOC morphotectonic province and the wide distribution of its hosts outside this province suggest that variations in temperature and humidity, such as those predicted to take place as a result of climate change (Logan and Powell 2001, Bale et al. 2002), may significantly affect its geographic distribution. A shift in the maximum temperature isotherms may favor D. rhizophagus dispersal into temperate areas or beyond its current high altitudinal limit. More detailed modeling studies are required to determine the effects that climate change may have on the distribution of D. rhizophagus. It also would be important to explore how temperature and humidity variables directly affect the development of the life cycle and population dynamics of this bark beetle, given that our study suggests these variables could also have strong influences at the meso- and micro-scale levels, as has been demonstrated in other Dendroctonus species (Logan and Amman 1986, Bentz et al. 1991, Turchin et al. 1991).
We are grateful to José Luis Benito Rosas Ortiz (SEMARNAT-Sonora, México); Ricardo Adán Peralta Durán and Pedro Hernández Díaz (SEMARNAT-Durango, México); Sergio Quiñonez Barraza (CONAFOR Durango-Sinaloa, México); Oscar de León Lara (CONAFOR-Sonora, México); Marcos Daniel Trujano Thomé and José Luis Aguilar Vitela (Secretaría de Recursos Naturales y Medio Ambiente, Durango, México); and Guillermo Sánchez-Martínez (INIFAP-Aguascalientes, México) for providing us with access to their collection records and for their logistic support, time, and availability for consultations. We thank Jorge E. Macías Sámano (Colegio de la Frontera Sur, Chiapas, México); Jane L. Hayes (Pacific Northwest Research Station, USDA Forest Service, LaGrande, OR); and three anonymous reviewers for their critical review of the manuscript. The project was funded by Comisión Nacional Forestal (CONAFOR, 69539) and Secretaría de Investigación y Posgrado-IPN (SIP-20090576). This work was part of M.G.M.s Ph.D. dissertation. She was a Consejo Nacional de Ciencia y Tecnología (207124) and Programa Institucional de Formación de Investigadores del Instituto Politécnico Nacional (PIFI-IPN) fellow.
References