-
PDF
- Split View
-
Views
-
Cite
Cite
Joseph Mccaffrey, Candace Galen, Between a Rock and a Hard Place: Impact of Nest Selection Behavior on the Altitudinal Range of an Alpine Ant, Formica neorufibarbis, Environmental Entomology, Volume 40, Issue 3, 1 June 2011, Pages 534–540, https://doi.org/10.1603/EN10304
- Share Icon Share
Abstract
Formica neorufibarbis Emery is a dominant alpine ant in North America. Above timberline, colonies nest under rocks, placing brood against the rock surface to warm during the day. We tested the hypothesis that nest rock preference represents a mechanism of behavioral thermoregulation and used a demographic model to explore its impact on the species' altitudinal range. Under sunny conditions rocks provide warm microclimates. Across a 400-m altitudinal gradient from below timberline to high alpine tundra, nest rocks warmed on average 5°C above ambient at mid-day, but cooled to near ambient before dawn. Soil was warmer at mid-day under rocks occupied by ants than under randomly chosen unoccupied rocks in the same area. Occupied nest rocks were painted white or black to manipulate temperature and test its impact on colony occupancy. In addition, black-painted and white-painted rocks of similar size were placed in the field to test for a temperature effect on nest site colonization. Likelihood of nest persistence and colonization decreased under cooler, white-painted rocks and depended strongly on rock warming capacity. Experimental results showed that nest site colonization and occupancy are favored in microsites maintaining mid-day temperature ≥22°C. Across the altitudinal gradient, 66-90% of nest microsites occupied by F. neorufibarbis met this temperature threshold. Conversely, none of the exposed microsites and only 15% of those microsites under randomly selected rocks warmed to 22°C at the high altitude site (3,900 m). Results support the conclusion that nest site preference contributes to the broad altitudinal distribution of F. neorufibarbis.
Insects exhibit a broad suite of behavioral adaptations for thermoregulation. In extremely cold climates insects depend on behavioral thermoregulation to maintain body temperature and hydration under dangerously arid and sub-freezing ambient conditions (Hodkinson 2003, Dillon et al. 2006). Some insects seek out solar tracking flowers to raise their own body temperatures (e.g., Kevan 1975), while others orient dark portions of their wings toward the sun's rays in an insect's version of solar tracking (Somme 2008). These behaviors potentially extend the species' niche beyond geographic or altitudinal boundaries dictated by ambient temperature. Though the importance of thermoregulation at high altitudes has long been known, most such work has focused on thermal limitations of individual foraging behavior rather than colony warming, and studies linking nest selection to altitudinal range have been few.
Many insect species engage in specialized nest construction to promote survival and reproduction over a broader range of environmental conditions than otherwise would be possible. Social insects are particularly adept at using nest architecture to maintain more stable temperature regimes than provided by ambient conditions (Jones and Oldroyd 2007). Orientation, color, and density of construction materials, shape, size, and other aspects of nest structure promote fitness via amelioration of cold and exposure. In ants and termites, nest site selection is shaped by two fundamental requirements: optimal conditions for brood development and shelter from biotic and abiotic risk factors (Brian 1973, Blüthgen and Feldhaar 2010). Mounds of the wood ant (Formica rufa L.) maintain stable nest temperatures across a broad latitudinal gradient, speeding up development and enhancing worker production in short growing seasons (Frouz and Finder 2007). Two species of termites build wedge shaped mounds oriented from north to south to allow for afternoon cooling (Jacklyn 1992). Ants also concentrate and exploit metabolic heat within nests to stabilize brood temperatures. Large (≈105-106 members) colonies of F. rufa raise temperatures nearly 10οC by aggregating at the center of the nest mound, while species with smaller colonies must rely on other modes of heat generation including decomposition of organic matter (Coenen-Stass and Schaarschmidt Band Lamprecht 1980, Rosengren et al. 1987).
Rock nesting is a common strategy for gaining shelter in exposed habitats (e.g., Van Pelt 1963). Here we examine nest rock selection in the alpine species, Formica neorufibarbis Emery (Hymenoptera: Formicidae). Though most ants are uncommon at high altitudes where cold temperatures severely limit brood development and food resources (Fisher 2010), F. neorufibarbis is abundant at up to 4,000 m above sea level (asl) in the Rocky Mountains of North America. Accordingly this species provides an excellent system for probing the role of nest site selection in persistence under cold environments.
Behavioral mechanisms for thermoregulation in F. neorufibarbis may underlie its preference for nesting beneath rocks above timberline, largely explaining the species' atypical success at high altitudes (Gregg 1963). Solar radiation absorbed by nest rocks is thought to maintain favorable temperature and humidity regimes for brood development, though this idea has not been directly tested (Bernstein 1976). Because workers relocate brood from subterranean nest galleries to the rock surface on warm days, thermoregulatory advantages associated with nest rock selection seem plausible (Billick 2001).
In this study we address the hypothesis that behavioral thermoregulation via nest rock selection contributes to the broad altitudinal distribution of F. neorufibarbis. To link behavior to spatial distribution and niche breadth we ask the following questions: 1) Does rock nesting enhance warming across the altitudinal range of F. neorufibarbis?, 2) Do ant colonies select among potential nest rocks with respect to temperature?, 3) Are cold nest rocks vacated by F. neorufibarbis and avoided during colonization?, and 4) How do thermoregulatory advantages of nest rock selection contribute to the high altitudinal range of F. neorufibarbis?
METHODS
Study System.
F. neorufibarbis is a small (3- to 4-mm) ant found from subalpine meadows to high alpine tundra in the Rocky Mountains (Bernstein 1976, Billick 1999). Colonies of F. neorufibarbis typically consist of 150–300 workers with one or more (often multiple) queens. At high altitudes, colonies nest beneath large rocks with galleries extending 10–15 cm below the soil surface (Bernstein 1976). Individual colonies often exploit several nearby rocks. Brood and workers aggregate against the rock's lower surface on clear warm days, returning to underground chambers when temperatures drop in the late afternoon. Ants raise a single brood of young over the summer growing season (Billick 1999). According to Billick (1997, 2001) extreme temperatures and restricted growing season length represent the major challenges for colony productivity, with little evidence of food limitation or density dependent regulation. Floral nectar accounts for ≈85% of colony diet; dead insects and seeds make up the remainder (Bernstein 1976).
We observed F. neorufibarbis at altitudes from 3,474 to 3,886 m asl on southeast slopes of Pennsylvania Mountain (Park Co., CO). The underlying Dolomite rock formation in this area is light in color (Tweto 1974). Along the 400-m altitudinal gradient from tree-line to the high alpine tundra, surface dwelling organisms experience a 4°C decline in mid-day ambient temperature (see results, Fig. 1). We sampled populations at four sites: a subalpine meadow just below timberline at 3474 m (SA); in the tree-line ecotone between subalpine forest and alpine tundra at 3581 m (TL); a tundra slope at 3,799 m (TS); and a boulder-field at 3,886 m (BF). Observations were made from June to August in 2006 and 2008.
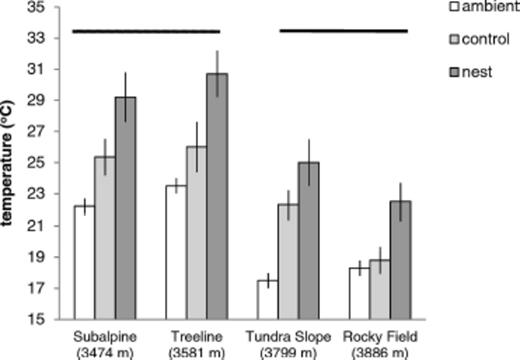
Ground surface temperature in the open (ambient), under randomly chosen unoccupied rocks (controls) and under rocks exploited for nests by F. neorufibarbis at four sites along an altitudinal gradient. Separate horizontal lines designate sites that differ significantly in ground temperature (P < 0.05). Regardless of altitude, the ground warmed to higher mid-day temperatures under nest rocks than randomly chosen controls (ANOVA, P < 0.0001; Table 1).
Characterization of the Altitudinal Temperature Gradient and Thermal Properties of Nest Rocks.
We conducted a survey to measure ground temperature variation along the altitudinal gradient and compare thermal properties of occupied nest rocks with those properties of randomly chosen unoccupied rocks and open ground. At each site, we located all nest rocks occupied by active ant colonies and measured the diameter of each to define size limits for rejecting physically unsuitable rocks (pebbles and boulders) from the pool of randomly selected unoccupied rocks chosen as controls. Based on the 95% confidence interval for nest rock size, we included rocks between 4.4 and 25.9 cm in diameter as controls in this study. Within each site, thermal regimes were characterized for a random sub-sample of 15 nest rocks and 15 nearby unoccupied (control) rocks of similar size. Mark–recapture surveys for F. neorufibarbis workers in tree-line and alpine tundra sites suggest that ants seldom move >1 m from a given nest rock (maximum observed travel distance averaged 73 ± 5 cm (SE), n=56; (C. G., unpublished data). Accordingly, all nest rocks and unoccupied rocks sampled for microclimate were separated by at least 1 m. Unoccupied rocks were chosen by locating the closest rock in the appropriate size range to a point designated by a random angle and distance (between 1 and 5 m) from each nest rock. Because ants and nest artifacts (tunnels or otherwise disturbed soil) were never observed beneath the unoccupied “controls” it is very unlikely that they were used as satellite nests for colonies whose nests we measured. Moreover, if one or more control rocks were incorrectly classified as unoccupied the error would be conservative, reducing our chance of detecting a difference in thermal regimes between nest rocks and controls.
Ground surface temperatures were measured three times at 30-s intervals under each nest rock and the nearest control rock and in the open by using a dual copper constantan thermocouple (Omega Inc., Stamford, CT). One temperature probe was placed on the ground ≈5 cm beyond the rock edge to measure ambient temperature and a second probe was placed directly under the center of the control rock or nest rock. Thermocouple placement temporarily disturbed colonies causing ants to disperse rapidly. Consequently, it is unlikely that any metabolic heat generated by ants affected measurements of ground temperature. All measurements were taken under clear skies from 13 to 20 August 2006, before dawn (400–600 h [MDT]) and at mid-day (1200–1400 hours [MDT]).
Excess temperature was calculated as the difference in temperature between the probe placed beneath the rock and the ambient (open) probe. We used one sample Student's t-tests to assess whether predawn and mid-day excess temperatures differed significantly from zero for nest rocks and controls. The three measurements made under each rock type per replicate were averaged before the analysis. Because excess temperature before dawn was biologically negligible (see results: “Thermal properties of nest rocks”) we focused on mid-day measurements in subsequent analyses. Mixed model analysis of variance (ANOVA) (ANOVA, PROC Mixed; SAS Vers. 9.2) was used to test for differences in ambient mid-day temperature among sites, with block comprising each nest rock and nearest control (within site) as a random effect. To test whether occupied rocks and control rocks differed in temperature across the altitudinal gradient, a similar ANOVA was run with temperature under rocks as the dependent variable, rock type (nest versus control), site and their interaction as fixed effects, and block as a random effect. A priori contrasts were used to test the hypothesis that colonies occupy rocks with enhanced heating capacity (control rocks versus nest rocks). We conducted an additional analysis of covariance (ANCOVA) to test whether rocks occupied by ants cool less sharply as temperature drops than unoccupied control rocks. Here, ground temperature beneath rocks was modeled as a linear function of ambient temperature with rock type as a categorical fixed effect. A significant interaction between rock type and ambient temperature would suggest that nest rocks and controls show different cooling responses as ambient temperature declines with altitude. Because this analysis directly compares the heating responses of nest rocks and controls to variance in ambient temperature, it pools effects from other unmeasured environmental differences among sites (e.g., in exposure or slope aspect) with the error variance.
Emigration and Colonization as Mechanisms of Thermoregulation.
Because survey data are correlative in nature they cannot directly address the idea that ants preferentially choose rocks radiating more heat over cooler ones. To address this hypothesis we first tested whether colonies of F. neorufibarbis vacate rocks because of reductions in temperature. At the TL site, we located 30 nest rocks separated by ≥1 m to avoid nonindependence between treatments. Spatial blocks (15) contained two nearby rocks with similar worker density (estimated at mid-day by lifting rocks and counting all visible ants beneath them as they dispersed). These nearby rocks were randomly assigned between black and white painting treatments. We applied Krylon brand five ball interior and exterior glossy paint to the top of each rock on 20 July 2008. Nests beneath black-painted and white-painted rocks were similar in worker number (n) before painting (respectively, n=47 ± 7 (SE) and n=41 ± 7). Number of workers per colony and mid-day temperature beneath rocks were surveyed on 24 and 28 July 2008 and again on 28 June 2009. Although worker counts at mid-day likely underestimate nest size, we assume that this error is not confounded with painting treatment. We next tested whether ants preferentially colonize warm rocks by placing 50 pairs of similar-sized white-painted and black-painted rocks in the field at the TL site on 30 July 2008 and monitoring colonization status and worker density on 27 June 2009. Mid-day soil temperature measurements were taken at the 2009 census beneath a subset of rocks that included all rocks colonized by ants and additional randomly chosen rocks to increase sample size to a total of 15 rocks of each color.
To test whether emigration rate varied between paint treatments, we used a mixed model repeated measures ANOVA (rm-ANOVA) with paint color as a fixed effect, replicate as a random effect, and worker number at each of the three census dates as the dependent variable. Fisher-exact tests were used to test for a relationship between the frequency of nest persistence or nest colonization and rock color. We also conducted logistic regression analyses to test whether odds of nest persistence and colonization depended on temperature. For the analysis of nest persistence temperature was averaged across all survey dates in 2008. For nest colonization we used measurements from the single survey in 2009.
Modeling the Impact of Nest Site Selection on the Altitudinal Range of F. neorufibarbis.
To test whether nest rock preference provides F. neorufibarbis with favorable microsites for colonization and persistence at high altitudes, we used survey data (“Thermal Properties of Nest Rocks”) to calculate the proportion (p) of nest rocks, control rocks, and open microsites at each altitude with mid-day temperature ≥22°C, the lowest temperature at which odds of both colonization and persistence were bounded away from zero. ANCOVA (GLM Procedure, SAS Institute 2006) was used to test whether the rate of decrease in p with altitude depends on mode of nest site selection: open ground nesting, random rock nesting, or nest rock preference. To conform to normality, values of p were square root transformed before the analysis.
Results
Thermal Properties of Nest Rocks.
At mid-day, excess temperature averaged 6.3 ± 0.71°C (SE) under nest rocks and 3.0 ± 0.65°C under unoccupied controls (for Ho of |$\bar x$|=0, P < 0.001 for both). Conversely, excess temperature before dawn although above zero (P < 0.0001) likely had little biological importance, averaging 0.87 ± 0.18°C under nest rocks and 0.84 ± 0.17°C under controls. As expected, ambient ground temperature at mid-day varied significantly among sites (F3,299=35.31, P < 0.0001; Table 1) with warmer temperatures at lower altitudes (3,474–3,581 m) and cooler temperatures at the higher alpine sites (3,799–3,886 m; Fig. 1). Temperature at mid-day under unoccupied control rocks and nearby nest rocks followed similar trends (Fig. 1), varying among sites (F3,295=12.35, P < 0.0001; Table 1) and declining significantly from the lowest two sites to the upper two sites (planned comparisons, P < 0.05, Fig. 1). Temperature was significantly lower under randomly chosen controls than nest rocks, independent of elevation (F1,295=94.54, P < 0.0001; Fig. 1). Although temperature under both control rocks and occupied nest rocks increased with ambient temperature, the slope for this relationship differed significantly between rock types (ANCOVA, treatment by temperature interaction F1,296=6.80, P < 0.01; Table 2). Nest rocks warmed more sharply than controls with increases in ambient temperature (linear regression, slope (β=0.98 ± 0.22, t=4.40, P < 0.0001 and (β=0.62 ± 0.20, t=3.17, P < 0.0025, respectively; Fig. 2). Results suggest that ants choose warmer microhabitats for nesting than predicted at random.
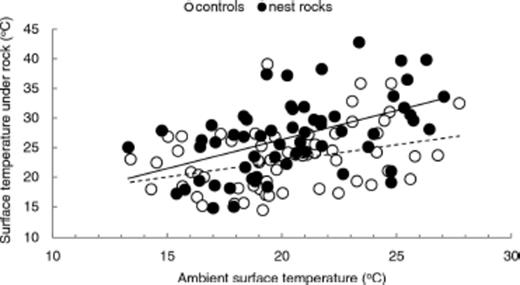
Mid-day surface temperature under randomly chosen unoccupied rocks (open circles, dashed line) and occupied nest rocks (filled circles, solid line). Although temperature under both random rocks and occupied nest rocks increased with ambient temperature, nest rocks warmed more with ambient temperature than unoccupied rocks (ANCOVA, P < 0.01, Table 2).
Mixed model analysis of variance in ground surface temperature at mid-day in the open and under nest rocks and randomly chosen controls (15 locations per site) at four altitudes. Separate models were run for temperature of open ground vs measurements under rocks
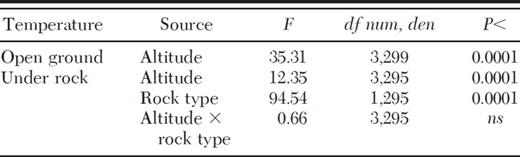
Mixed model analysis of variance in ground surface temperature at mid-day in the open and under nest rocks and randomly chosen controls (15 locations per site) at four altitudes. Separate models were run for temperature of open ground vs measurements under rocks
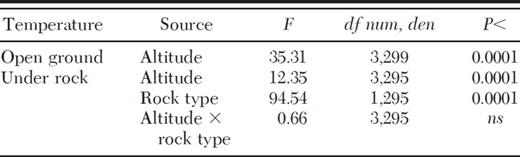
Analysis of covariance between surface temperature under nest rocks and randomly chosen controls and ambient temperature of nearby open ground. Measurements were taken at mid-day in four sites along a 400 m altitudinal gradient with 15 spatial replicates per site. For this analysis, sites were pooled to assess responses to the full range of mid-day temperature. experienced by F. neorufibarbis
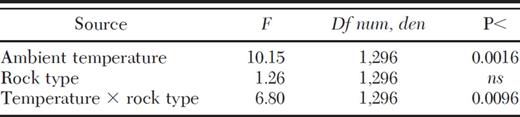
Analysis of covariance between surface temperature under nest rocks and randomly chosen controls and ambient temperature of nearby open ground. Measurements were taken at mid-day in four sites along a 400 m altitudinal gradient with 15 spatial replicates per site. For this analysis, sites were pooled to assess responses to the full range of mid-day temperature. experienced by F. neorufibarbis
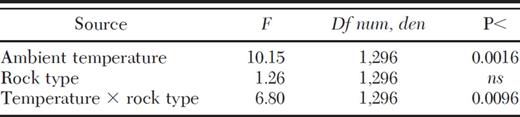
Emigration and Colonization as Mechanisms of Thermoregulation.
Rock color had a strong effect on surface temperature. At mid-day, temperature in 2008 (nest occupancy experiment) averaged 21.9 ± 0.55°C under black-painted rocks and 18.6 ± 0.35°C under white ones. In 2009, ambient temperatures were warmer and mid-day temperature averaged 29.9 ± 1.0°C under black-painted rocks and 22.5 ± 0.71°C under white ones. Variation in ant density over time depended strongly on nest rock color (for the time by color interaction, F3,75=3.38, P < 0.0227; Table 3 ; Fig. 3). Counts of workers per nest changed little under black-painted rocks, but dropped sharply under white ones (Fig. 3). Declining worker density under the cooler white rocks was accompanied by nest abandonment. After 8 d ants remained in all nests under black-painted rocks, but vacated 13% of nests under white ones (Fisher-exact test, P < 0.013). This trend continued the following summer, with persistence rates of 93% for nests under black-painted rocks and 80% for nests under white ones in 2009 (Fisher-exact test, P < 0.045). Nest rock colonization was rare: 94% of experimentally added nest rocks remained vacant in 2009. Nonetheless, black-painted rocks tended to receive more colonists than white ones (12% versus 2%, Fisher-exact test, P < 0.088). Mid-day temperature beneath painted rocks was a strong predictor of nest persistence in 2008 and nest colonization in 2009 (logistic regression, respectively, χ2=5.33, df=1, P < 0.021, and χ2=5.46, df=1, P < 0.0194; Fig. 4) supporting a role for these behaviors as mechanisms of thermoregulation. Mid-day temperature in 2008 averaged 16.6 ± 0.8°C under vacated nest rocks and 20.5 ± 0.3°C under occupied ones. In 2009 mid-day temperature averaged 24.9 ± 0.9°C under vacant nest rocks and 31.5 ± 1.8°C under colonized ones. Logistic regression models suggest that nest persistence is possible even at relatively cool temperatures (Fig. 4 a) while new nest colonization is restricted to warmer ones (Fig. 4 b). The regression model identified 22°C as the lowest temperature at which the 95% confidence interval for colonization was bounded away from zero (Fig. 4).
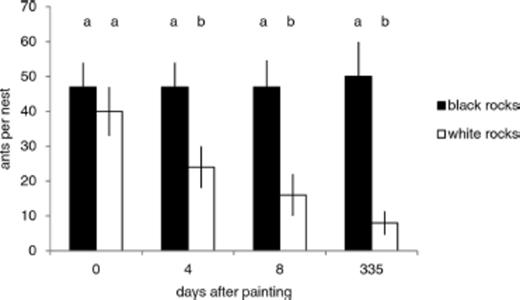
Number of ants counted under black-painted and white painted nest rocks. Bars show mean values and error brackets denote SE. Unique letters above bars show dates on which density of ants differed significantly between color treatments.
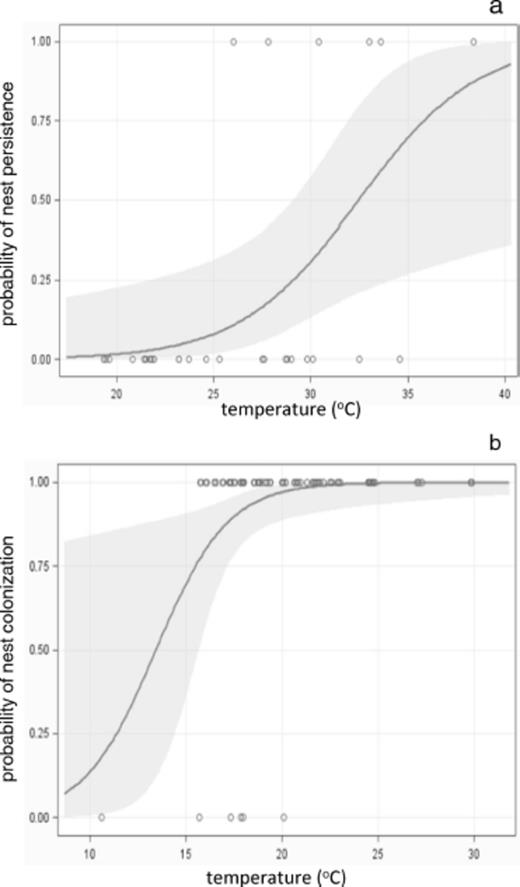
Logistic regression of (a) nest persistence and (b) nest rock colonization as functions of mid-day surface temperature under painted rocks. Curves show (a) probability of nest persistence and (b) probability of new nest colonization as functions of temperature. Open circles denote surface temperature in occupied and empty nests. Shaded envelopes indicate 95% confidence intervals for probability functions. At both demographic stages, P < 0.021 for the impact of temperature on nest fate.
Repeated measures analysis of variance to test for effect of nest rock temperature on rate of nest abandonment. Nest rocks were either painted white (to reduce solar warming) or black (control) and density of ants per nest sampled before painting, twice afterward during the same season, and once more a year later

Repeated measures analysis of variance to test for effect of nest rock temperature on rate of nest abandonment. Nest rocks were either painted white (to reduce solar warming) or black (control) and density of ants per nest sampled before painting, twice afterward during the same season, and once more a year later

Impact of Nest Selection on Altitudinal Range.
Survey data on thermal properties of potential nest sites (Fig. 1) allowed us to estimate p the proportion of microsites having temperatures above the 22°C mid-day temperature threshold (Fig. 4). Plotting p against altitude shows that under all nest selection scenarios, likelihood of colonization by F. neorufibarbis decreases in high alpine sites (Fig. 5). However, the ANCOVA results suggest that the rate at which p declines with altitude depends on mode of nest site selection (nest microsite type by altitude interaction, F2,6=6.92, P < 0.0276). Only for occupied microsites is colonization favored across the species entire altitudinal range: (range, of p from 0.66 to 0.95). On open ground and under unoccupied control rocks, p declines to zero and 0.15, respectively at 3,886 m asl.
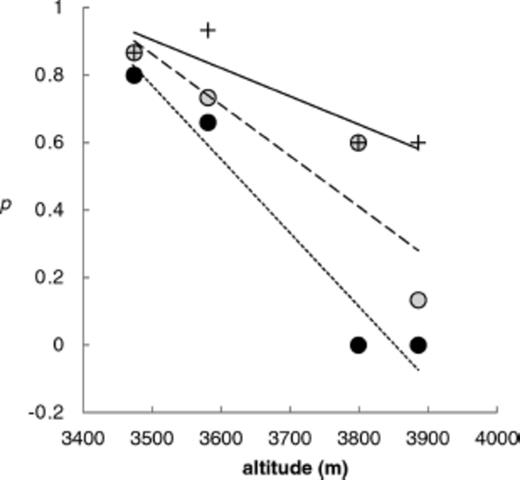
Proportion (p) of potential Formica neorufibarbis nesting microsites at four altitudes with mid-day temperatures permissive of nest colonization and persistence. Open ground nesting is indicated by filled circles, and small dashed line, random rock nesting by open circles and large dashed line, and nest rock preference by plus symbols and solid line. Lines differ significantly in slope at P < 0.0276.
Discussion
Thermal Benefits of Rock Nesting.
The results of this study show that F. neorufibarbis engages in nest rock preference as a form of behavioral thermoregulation in alpine environments. Nest rocks were on average 3.3°C warmer than nearby unoccupied controls. Several abiotic factors may contribute to this difference in microsite warming. The angle of nest rocks may enhance solar exposure: mound-building ants in thermally stressful environments orient the slope of their mounds to capture morning sunlight (Romey 2002). Physical characteristics of rocks, such as surface area, volume, or density should also affect their thermal properties (Robinson 2008). As our painting manipulation demonstrated, rock color may also influence thermal capacity (Tweto 1974).
Another possibility is that the temperature difference between nest rocks and unoccupied controls is mostly because of biotic factors. The metabolic activity of the ants (Rosengren et al. 1987) and the microbial decomposition of organic materials in the nest soil (Romey 2002) contribute to excess warming of nests in other ant species. In the current study, it is unclear why white painted rocks were abandoned if ant metabolism is responsible for nest warming. Moreover, ants of F. neorufibarbis descend into subterranean galleries to avoid cold conditions at night, suggesting limited capacity to actively raise nest temperature. Future experiments, measuring thermal properties of nests from which ants are removed with barriers installed to prevent recolonization, could help resolve this question. Regardless of the specific mechanisms of nest warming, it seems that rocks provide a range of thermal conditions for potential colonization by F. neorufibarbis. This raises the question: why are nests restricted to warmer sites?
Nest thermoregulation is an important adaptation of insects to climates with variable temperature and moisture regimes (Hodkinson 2003). Social insects perform numerous thermoregulatory behaviors that enable them to thrive in environments that would be intolerable for their nonsocial counterparts (Jones and Oldroyd 2007). For ants, which use many of these behaviors, temperature is critical for both the developmental rates of juveniles and the daily activity level of adults (Seeley and Heinrich 1980). Rock nesting facilitates two modes of thermoregulation for F. neorufibarbis: brood translocation from lower galleries to the rock surface on warm days (Billick 2001) and selection of warmer microhabitats during colonization (this study). These mechanisms likely work in tandem, with nest rock preference enabling workers to reach a daily metabolic threshold for activity more quickly and enhancing the advantages of brood translocation. In alpine habitats with cold summer temperatures and short growing seasons, higher insect metabolism provides significant benefits for colony productivity (Billick 1997).
Emigration and Colonization as Mechanisms of Nest Site Selection.
When nest rocks were painted white or black to manipulate temperature, F. neorufibarbis colonies persisted under black rocks with temperatures similar to unpainted controls, but vacated white (cooled) rocks. Furthermore, vacated nests were not recolonized the following summer. Similarly, ants tended to colonize cooler white-painted rocks at a low rate compared with warmer black-painted ones. Other studies (e.g., Kuriachan and Vinson 2000, Miyata et al. 2003) also demonstrate that ants migrate their nests based on temperature and seasonal cues. Our results are in accord with these findings and show that temperature is crucially important for colony persistence and microsite colonization in F. neorufibarbis. Outcomes reinforce the idea that rock nesting confers thermal benefits on F. neorufibarbis colonies. Observed rates of emigration for cooled nests suggest that F. neorufibarbis colonies have a higher temperature threshold for moving currently occupied nests than colonizing new ones (Fig. 4 a versus 4b). This reluctance suggests that vacating extant nests exacts a greater energy cost than colony expansion via new nest colonization (Blüthgen and Feldhaar 2010).
Ecological and Evolutionary Significance of Nest Rock Preference.
Our results show that F. neorufibarbis uses nest rock preference as a form of behavioral thermoregulation. By selecting warmer microsites, F. neorufibarbis may alter its niche in a manner conforming to a niche construction scenario. While most accounts of niche construction focus on physical alterations of the environment by organisms (e.g., beaver dams or ant mounds), habitat selection is also a form of niche construction because organisms change the environments they experience (their realized niche) by relocating (Laland and Sterelny 2006). Considering habitat selection as niche construction is useful because it highlights how behaviors for selecting microhabitats might influence the subsequent evolution of behaviors within those microhabitats. There may be interesting feedback relationships in F. neorufibarbis, for example, between the evolution of behaviors for brood translocation and nest rock preference.
Our model for range extension over the altitudinal temperature gradient on Pennsylvania Mountain (Fig. 5) supports the view that the success of F. neorufibarbis in colonizing high alpine environments is enhanced primarily by nest rock preference (Gregg 1963). The model suggests that random rock nesting behavior would not permit range expansion to the highest altitudes surveyed in this study. The capacity to choose warmer microsites likely underlies the unusual tolerance of F. neorufibarbis for the cold and arid conditions of high alpine tundra in the Rocky Mountains. Selection of warmer nest rocks during colonization and rejection of cooler ones by emigration should influence the distribution of F. neorufibarbis on two distinct spatial scales. Locally, the behavior alters the distribution of nests, which will be shaped by the availability of warm microsites. Geographically, nest rock preference should extend the species' altitudinal range to a greater extent than rock nesting alone, assuming that the temperature gradient on Pennsylvania Mountain is similar to other altitudinal clines in temperature for the Rocky Mountains (Fig. 5). This assumption seems reasonable, because lapse rate is nearly constant in July across Rocky Mountain latitudes (Moore 1956). If temperature is a limiting factor for this species, then rock nesting, brood translocation, and nest site selection are all behaviors that should extend its altitudinal range.
In conclusion, results suggest that nest rock preference is a mechanism for behavioral thermoregulation in F. neorufibarbis—nest temperatures are higher than temperatures under unoccupied rocks, temperature plays an important role in colony persistence, and warmer rocks are more likely to be colonized. Our results imply that nest rock preference is an important driver of niche breadth in response to limiting environmental factors. The selection of warmer nest sites during colonization and emigration may enable F. neorufibarbis to extend its niche to higher altitudes than otherwise would be possible. As with so many ant species, F. neorufibarbis provides a rich system for probing the complex relationship between habitat selection, niche breadth, and how the feedback between organism and environment influences the evolution of behavior.
We thank R. Holdo, I. Billick, J. Geib, A. Michels; K. M. Becklin and S. Kelso for helpful conversations and comments on the manuscript; and J. J. Rabenold and Adam J. McKinley for assistance in the field. Funding was provided by NSF grant DEB0316110 to C. Galen.
References