-
PDF
- Split View
-
Views
-
Cite
Cite
Micky D. Eubanks, David E. Carr, John F. Murphy, Effects of Virus Infection of Mimulus guttatus (Phrymaceae) on Host Plant Quality for Meadow Spittlebugs, Philaenus spumarius (Hemiptera: Cercopidae), Environmental Entomology, Volume 34, Issue 4, 1 August 2005, Pages 891–898, https://doi.org/10.1603/0046-225X-34.4.891
- Share Icon Share
Abstract
Herbivorous insects often feed on pathogen-infected plants in nature, and it is likely that pathogen infection alters host plant quality. We documented the effects of plant infection by a widespread plant virus on host plant quality for a generalist insect herbivore and tested the hypothesis that these effects vary among plant genotypes. We found that infection by Cucumber mosaic virus (CMV) altered the host plant quality of Mimulus guttatus (Phrymaceae) for meadow spittlebugs, Philaenus spumarius (Hemiptera: Cercopidae). The effects of CMV infection on host plant quality, however, varied among full-sib M. guttatus families, suggesting that these effects vary among plant genotypes. In most full-sib families, CMV infection either had no effect on host plant quality or increased host plant quality as measured by spittlebug size and development time. In a few M. guttatus families, however, CMV infection decreased host plant quality. There was no relationship between the effect of CMV infection on plant growth and the subsequent effect of CMV infection on spittlebug performance, suggesting that broad changes in host plant performance (e.g., growth rate) were not responsible for the effects of CMV infection on host plant quality for spittlebugs. We suggest that future studies of the effects of pathogen infection of plants on insect herbivores consider variation among plant genotypes in the mechanisms and ecological consequences associated with these effects.
Plants are often simultaneously attacked by pathogens and insect herbivores, and pathogen infection of plants may have profound consequences for the insect herbivores that consume them (Fritz and Simms 1992, Felton et al. 1999, Gilbert 2002). Pathogen infection of plants may affect herbivorous insects by modifying the expression of resistance traits and/or by altering the nutritional quality of the plant. Some recent studies, for example, have shown that pathogen infection may alter expression of anti-herbivore traits through "cross-talk" or interference among signaling pathways. Interference among signaling pathways associated with systemic acquired resistance to pathogens, and the expression of induced resistance to insects can result in decreased resistance to insect herbivores (e.g., Doherty et al. 1988, Maleck and Dietrich 1999, Bostock et al. 2001, Dicke and Hilker 2003, Rostás et al. 2003). In tomato, for example, the expression of defensive proteins associated with resistance to herbivory by beet armyworm caterpillars can be suppressed by pathogen infection, and consequently, pathogen-infected tomato plants may suffer greater levels of herbivory and corresponding fruit loss (Stout et al. 1999, Thaler et al. 1999). Similar negative effects of pathogen infection on resistance against beet armyworm probably occur in Arabidopsis thaliana (Cipollini et al. 2004), and fungal infection in peanuts can reduce phenolic production and increase beet armyworm caterpillar survival and development (Cardoza et al. 2003). Other studies, however, suggest that pathogen infection may actually increase the expression of antiherbivore resistance traits and decrease host plant quality for insects (Thaler et al. 2002). Bacterial infection of A. thaliana, for example, can lead to enhanced resistance against cabbage looper caterpillars (Cui et al. 2002), and pathogen infection may lead to increased resistance against gall-inducing insects in willow (Ollerstam and Larsson 2003).
Likewise, pathogen infection of plants alters photosynthetic rates, translocation of water and nutrients, and cellular respiration (Ellis et al. 1981, Pennypacker et al. 1990, Culver et al. 1991, Agrios 1997), traits strongly associated with the nutritional quality of plants for herbivorous insects (Slansky and Rodriguez 1987, Brodbeck et al. 2004, Lempa et al. 2004). In silver birch, for example, infection by a fungal pathogen increases the concentration of free amino acids available for aphid consumption, resulting in more fecund aphids and larger aphid populations (Johnson et al. 2003). Similarly, fungus infection increases the concentration of soluble sugars that are positively correlated with herbivore performance in peanuts (Cardoza et al. 2003). In other cases, however, pathogen infection can decrease host plant quality for insect herbivores. Leaf beetles that feed on creeping thistle, for example, preferentially consume and have higher fitness when fed healthy thistle versus thistle infected with the fungus, Phoma destructiva (Krues 2002).
Although this area of research is still rapidly developing, the results to date strongly suggest that the effect of pathogens on host plant quality is likely to vary within and among plant-pathogen systems and that work with many systems is needed before we fully understand this variation. In addition, most of the studies that have been conducted so far focus on the effects of pathogen infection on insect performance in one or a few varieties of agricultural crops (e.g., Thaler et al. 1999,2002). Consequently, we know very little about the effects of pathogen infection of naturally occurring plants on insect herbivores. Additionally, we know very little about the level of genetic variation that might exist within and among plant populations for these effects. It is possible that in naturally occurring plant populations the effect of pathogens on host plant quality for insect herbivores will be genotype specific (Fritz and Simms 1992). In this study, we used a greenhouse experiment to test the hypothesis that infection by a widespread plant virus alters the performance of an insect herbivore and that this effect varies among genotypes of the host plant. We focus on a widespread herbaceous plant of western North America, Mimulus guttatus (Phrymaceae, formerly Schrophulariaceae; Beardsley and Olmstead 2002), a highly polyphagous insect herbivore, Philaenus spumarius (Hemiptera: Cercopidae), and a widespread plant virus that infects >1,200 plant species (Cucumber mosaic virus). The specific objectives of this study were to (1) quantify the effects of CMV infection of M. guttatus on spittlebug performance (development time and adult biomass), (2) determine if there is genetic variation within and among M. guttatus populations for the effects of CMV infection on herbivore performance, and (3) determine if there is a relationship between the intensity of CMV infection and spittlebug performance when reared on CMV-infected plants. This is one of the first studies to document the effects of pathogen infection on host plant quality for insects in a naturally occurring plant species and one of the first studies to quantify genetic variation in plants for pathogen-induced changes in host plant quality.
Materials and Methods
Study Species.
The yellow monkeyflower, M. guttatus, ranges throughout western North America from Mexico to Alaska, occupying a variety of moist, open habitats and is locally abundant in all of the Pacific coast states (Munz and Keck 1973). M. guttatus plants can be either perennial or annual depending on soil moisture. Field-collected M. guttatus seeds for this experiment came from two annual populations: one (M5) in Santa Clara County, CA (37°17′ N, 122°09′ W) and the other (M13) in Napa County, CA (38°33′ N, 122°22′ W) (Carr and Eubanks 2002).
Meadow spittlebugs, P. spumarius, are perhaps the most polyphagous of all insect herbivores, with over 500 recorded host plant species (Weaver and King 1954, Hamilton 1982, Owen 1988). Meadow spittlebugs are univoltine. Females lay their eggs on plant tissue or in the soil in the fall, eggs overwinter, and first-instar nymphs hatch in the spring. In coastal California, meadow spittlebugs are the most abundant herbivore on M. guttatus. Infested plants typically host one to two spittlebug nymphs (M.D.E. and D.E.C., unpublished data). Meadow spittlebugs initially attack during the rosette stage in early spring, and nymphs develop on the plants throughout spring and into early summer. Once a feeding site is established, nymphs feed on xylem and generate the spittle for which they are named (Severin 1950, Wiegert 1964, Horsfield 1977). The spittle mass covers their bodies and primarily protects nymphs from desiccation (Weaver and King 1954, McEvoy 1986). Nymphs rarely move to a new plant once they settle and usually complete development (five nymphal instars) on a single plant. In a previous experiment, we found that herbivory by a single P. spumarius nymph can reduce the biomass and flower production of M. guttatus plants by 10-30% (Carr and Eubanks 2002).
There is little information on naturally occurring viruses in M. guttatus and other noncrop plants. Cucumber mosaic virus was chosen for this study because it is ubiquitous in nature with one of the broadest host ranges among viruses that infect plants (>1,000 plant species), it is known to attack close relatives of M. guttatus (Tomlinson et al. 1970, Palukaitis et al. 1992), and M. guttatus plants often have symptoms consistent with CMV virus infection in the field (Carr et al. 2003). The widespread occurrence of cucumber mosaic virus in nature is due, in part, to its nonpersistent mode of aphid transmission, meaning that an aphid can acquire CMV from an infected epidermal cell during a brief probe of the plant and with no latent period transmit the virus to another plant (Kennedy et al. 1962, Tomlinson 1987). We have shown in previous studies that M. guttatus is fully susceptible to CMV infection (Carr et al. 2003), that there is genetic variation associated with resistance and tolerance to infection (Carr et al., unpublished data), and that CMV infection reduces biomass and flower production of M. guttatus plants by as much as 15% (Carr et al. 2003).
Crossing Design.
In the fall of 1998, field-collected seed from M. guttatus populations M5 and M13 were sown in a pollinator-free greenhouse at the Blandy Experimental Farm (BEF) in Boyce, VA. Population M5 was represented by 13 full-sib families, and population M13 was represented by 12 full-sib families. One individual grown from each of these families served as the maternal plant for the production of seed. All flowers were emasculated in bud before hand pollination. To produce seed, individuals from the same population were randomly paired and pollen was applied directly from the anthers of the pollen donor to the receptive stigma of the recipient. Seeds were harvested when fruits were mature and stored at room temperature.
Experimental Design.
Forty seeds per full-sib family were sown on 13 March 2001 into 72-mm square plastic pots filled with Pro Mix (Premier Peat, Riviére-du-Loup, Québec, Canada) growing medium in a greenhouse at Auburn University, Auburn, AL. On 27 March, 20 randomly selected seedlings per treatment per full-sib family were transplanted into individual 72-mm pots. The experiment was a randomized complete block design with population (M5 and M13), full-sib family (10 families from M5 and 13 families from M13), and virus (inoculated or not inoculated) replicated twice within each of five blocks (460 total plants and a total of 10 replicates).
Cucumber mosaic virus strain Fny was used throughout this project (kindly provided by P. Palukautis, Scottish Crop Research Institute, Invergowrie, Scotland). On 9 April 2001, M. guttatus plants were inoculated with CMV by rub-inoculation onto the oldest two to three leaves. Inoculum consisted of systemically infected Nicotiana tabacum cultivar Kentucky 14 tissue ground in 50 mM potassium phosphate buffer, pH 7.0, and 10 mM sodium sulfite (1 g tissue: 10 ml buffer). Control plants were mock inoculated with healthy Kentucky 14 tissue ground in buffer. M. guttatus plants were inoculated in blocks with fresh inoculum used for each block. A young Kentucky 14 plant was inoculated with each block to confirm inoculum infectivity. In each case, the Kentucky 14 plant developed typical systemic infection symptoms, confirming infectivity of the inoculum.
On 12-13 April 2001, second- and third-instar P. spumarius nymphs were collected from Point Reyes National Seashore, CA. Spittlebugs were packed in plastic bags and provided fresh plant material for transport to Auburn University. On 15 April, one randomly selected spittlebug (a typical number for infested plants) was applied to each of the plants. All plants were in a large rosette stage. Most nymphs immediately established feeding sites on the plants. On 16-18 April, nymphs that failed to establish a feeding site were replaced.
The experiment was monitored daily for adult spittlebug emergence and the experiment was halted after the last spittlebug molted into an adult. We retrieved 247 adult spittlebugs that were still within their spittle mass and could be definitively assigned to a given plant. To ensure that adult spittlebugs were properly matched with their respective host plants, we included in the study only newly molted adults that were still residing within their drying spittle mass. Because cages were not used in this study, adults found on the surface of plants outside of a spittle mass could have originated from another plant in the experiment. Spittlebugs were dried and weighed to the nearest 0.01 mg with a microbalance. Plants were harvested at ground level, wrapped in aluminum foil, dried to a constant weight at 50°C, and weighed to the nearest 0.01 g.
Statistical Analysis.
Adult spittlebug biomass and development time were both log-transformed to meet the assumptions of analysis of variance (ANOVA). The effect of block, population, family nested within population, CMV infection, and the interactions of CMV infection with family and population on the biomass and development time of adult spittlebugs were analyzed by ANOVA using SAS Proc GLM (version 8.2; SAS Institute 2001). Family was a random effect in the models. Means were compared using Bonferroni-adjusted mean separation tests. To determine if broad changes in plant development caused by cucumber mosaic virus infection (e.g., growth) were responsible for changes in host plant quality for spittlebugs, we regressed the effect of CMV infection on plant biomass (biomass of control plants minus biomass of infected plants) on the performance of spittlebugs reared on CMV-infected plants (biomass and development time, respectively). All calculations used family means. A positive relationship between the effect of CMV on plant biomass and spittlebug biomass would indicate that spittlebugs reared on M. guttatus plants that were negatively affected by CMV were larger, on average, than those reared on M. guttatus plants that tolerated CMV infection. Likewise, a negative relationship between the effect of CMV on plant biomass and spittlebug development time would indicate that spittlebugs that were reared on M. guttatus plants whose growth was reduced the most by CMV developed faster than those reared on plants that tolerated CMV infection.
Results
Cucumber mosaic virus infection of M. guttatus significantly affected the biomass of adult spittlebugs, but this effect varied among M. guttatus families (significant CMV × family [population] interaction; Table 1). In population M13, for example, spittlebugs reared on CMV-infected plants were larger than those reared on control plants in one-half of the families, but smaller in the other half (Fig. 1A). Cucumber mosaic virus infection significantly affected spittlebug biomass in two of these families (Bonferroni-adjusted mean separation tests, P < 0.05). Cucumber mosaic virus infection increased spittlebug biomass by ≈33% in family 2 and by almost 100% in family 17 (Fig. 1A). In population M5, the effects of CMV infection on spittlebug biomass were more consistent; spittlebugs reared on infected plants were larger than those reared on control plants in 11 of 13 families, and the increase in spittlebug biomass was significant in 5 of these families (families 17, 19, 21, 34, and 48; Fig. 1B). Differences in the way CMV infection affected spittlebug biomass in the two populations resulted in a significant CMV × population interaction (Table 1).
ANOVA of the effects of block, family nested within population, population, virus infection, and the interactions of virus infection with family and population on adult biomass and development time of spittlebugs
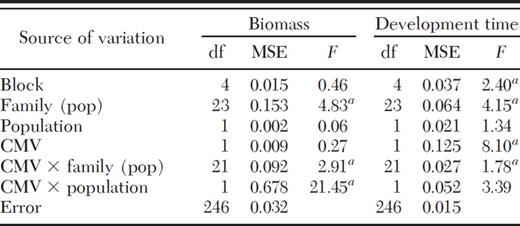
P < 0.05.
ANOVA of the effects of block, family nested within population, population, virus infection, and the interactions of virus infection with family and population on adult biomass and development time of spittlebugs
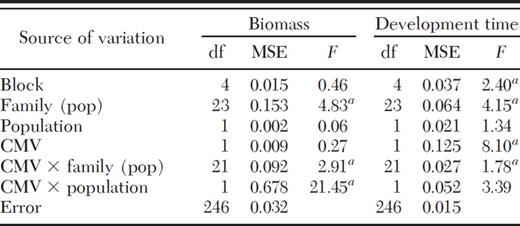
P < 0.05.
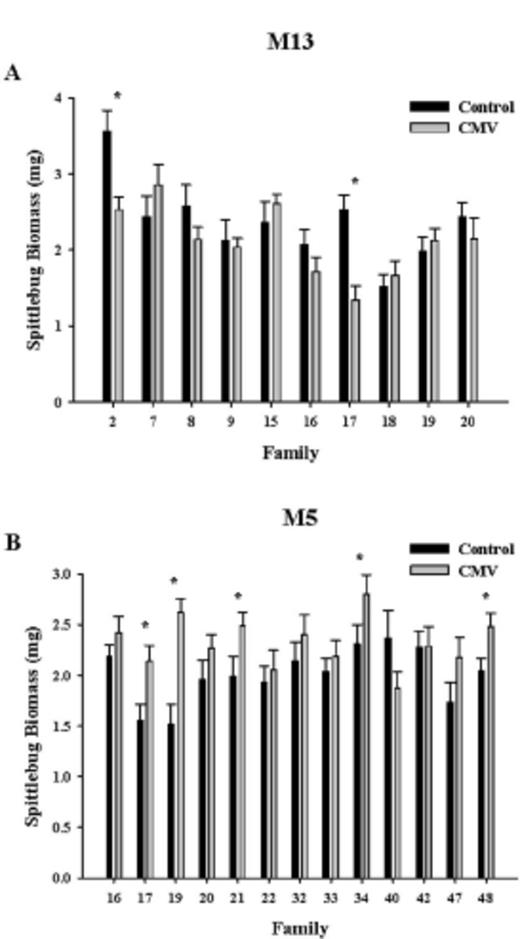
Biomass (mg; mean ± SE) of meadow spittlebugs reared on uninfected and Cucumber mosaic virus-infected M. guttatus plants from populations M13 (A) and M5 (B). * Significant differences in the weight of spittlebugs reared on control and infected plants from the same full-sib M. guttatus family.
Likewise, CMV infection altered the development time of spittlebugs, but this effect varied among M. guttatus families as well (significant CMV × family [population] interaction; Table 1). For example, CMV infection significantly increased development time for spittlebugs in family 2, but CMV infection significantly decreased development time in family 15 (Fig. 2A). In population M5, CMV infection increased spittlebug development time in three families, decreased development time in five families, and had no effect in two families (Fig. 2B). These effects were statistically significant in four families (significant decrease in development time in families 19, 32, 42, and 47).
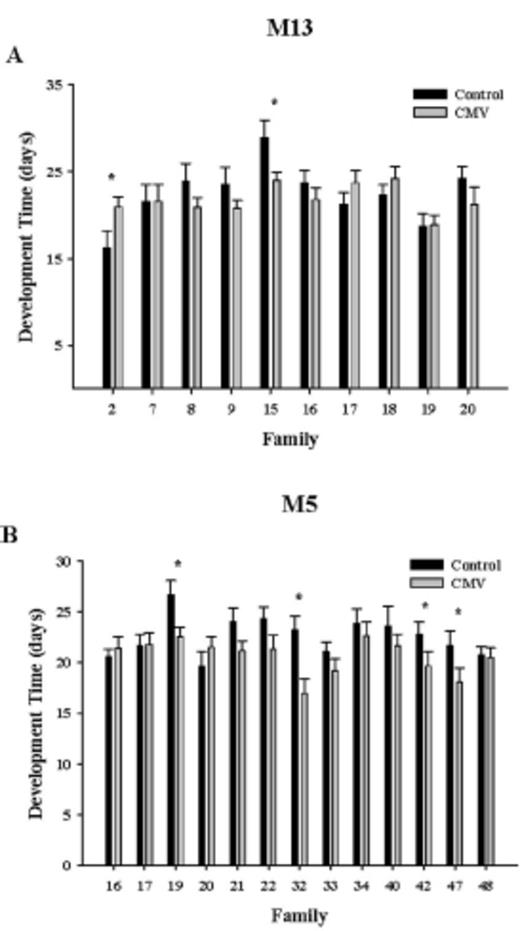
Development time (days; mean ± SE) of meadow spittlebugs reared on uninfected and Cucumber mosaic virus-infected M. guttatus plants from populations M13 (A) and M5 (B). * Significant differences in the development time of spittlebugs reared on control and infected plants of the same full-sib M. guttatus family.
There was no relationship between the effect of CMV infection in M. guttatus plants (the change in plant biomass caused by CMV infection) and the effect of CMV infection on subsequent biomass production of spittlebugs (Fig. 3A; y = 3.36x + 2.11, R2 = 0.06, P = 0.25). The relationship between the effect of CMV infection on plant growth and spittlebug development time was also not significant (Fig. 3B; y = 12.57x + 21, R2 = 0.03, P = 0.43). These results suggest that changes in M. guttatus quality for spittlebugs caused by CMV infection of M. guttatus were not caused by impacts that change plant growth.
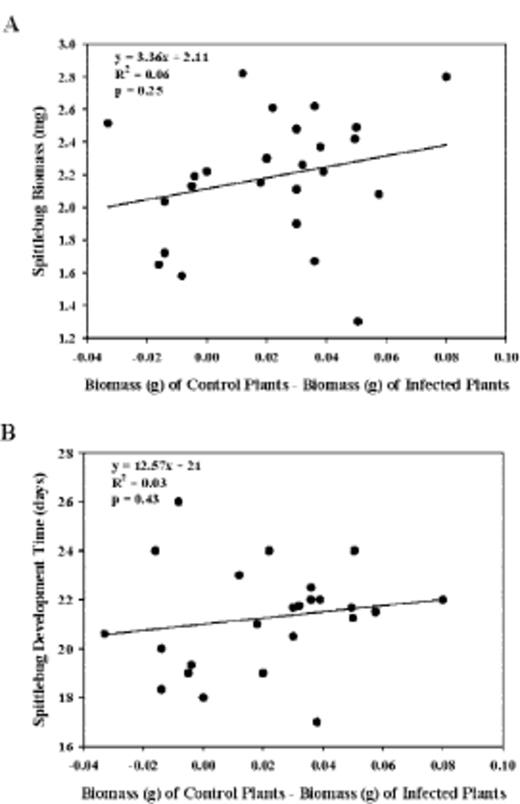
(A) The relationship between the effect of CMV infection on plant biomass production (g) and the biomass of adult spittlebugs (mg) reared on CMV-infected plants. (B) The relationship between the effect of CMV infection on plant biomass production (g) and the development time of spittlebugs (days) reared on CMV-infected plants.
Discussion
Infection of M. guttatus plants by CMV-induced changes in host plant quality for meadow spittlebugs, but this effect varied among and within M. guttatus populations. Cucumber mosaic virus infection decreased host plant quality in some families, increased host plant quality in some families, and had no effect on spittlebug performance in other families. These results suggest that the mechanisms underlying the effects of CMV on host plant quality for spittlebugs vary among M. guttatus genotypes. Our regression analysis of the effect of CMV infection on plant biomass versus spittlebug performance suggest that changes in host plant quality caused by CMV infection are not caused by broad changes in plant growth or plant vigor, but are probably caused by more specific CMV-induced changes in plant defensive traits or in traits that affect the nutritional quality of the plant. In ribwort plantain (Plantago lanceolata), for example, fungal pathogens can induce the production of iridoid glycosides that deter attack by generalist insect herbivores, and the magnitude of this effect varies among P. lanceolata genotypes (Marak et al. 2002). We have not studied the physiological changes that occur in CMV-infected M. guttatus plants yet, but it is likely that CMV infection alters both kinds of traits. For example, CMV stimulates the salicylic acid pathway that results in systemic acquired resistance in many plants (Murphy et al. 2001) and is likely to do so in M. guttatus plants. Induction of this pathway may suppress the production of anti-herbivore compounds as it does in many plants, including iridoid glycosides that are thought to be important defenses against generalist herbivores in plants of this family (Bowers et al. 1992, Marak et al. 2002). Cucumber mosaic virus infection may even alter the expression of morphological defenses that affect spittlebug performance. Spittlebugs feed on xylem, a plant tissue that is thought to be largely devoid of alleleochemicals (Wiegert 1964, Horsfield 1977, Crews et al. 1998, Malone et al. 1999,2002, Ponder et al. 2002). Consequently, defensive chemistry of host plants is thought to be an unimportant component of spittlebug resistance in plants. Morphological traits such as trichome size and density, stem width and hardness, and axil angle have been shown to provide plants with resistance against spittlebugs (Hoffman and McEvoy 1985; McEvoy 1986), and a previous study of M. guttatus suggests that trichome density is negatively correlated with spittlebug development (J. D. Tindle, D. E. Carr, and M. D. Eubanks, unpublished data). Recent studies have shown that trichomes are induced by herbivore feeding in many plant species (Traw 2002, Traw and Dawson 2002, Dalin and Bjorkman 2003) and that pathogen induction of the salycilic acid pathway and systemic acquired resistance can dramatically interfere with trichome production (Traw and Bergelson 2003). Thus, it is possible that infection of M. guttatus could suppress the production of trichomes resulting in an increase in spittlebug performance when reared on infected plants. This is an intriguing hypothesis that we hope to test in the near future.
Alternatively, plant viruses often increase the concentration of amino acids, carbohydrates, or both, in plants (Orlob and Arny 1961, Fereres et al. 1990, Shalitin and Wolf 2000) and the increased availability of these nutrients can result in enhanced herbivore performance on virus-infected plants (Fereres et al. 1988,1989, Fiebig et al. 2004). Most of these examples, however, involve increased nutrient availability in the phloem and subsequent increases in host plant quality for phloem-feeding insects (e.g., Johnson et al. 2003). Virus transport through xylem tissues has been reported for several virus species (Kahn et al. 1994, Urban et al. 1989, Opalka et al. 2000, Verchot et al. 2001, Moreno et al. 2004), but CMV was shown to move through plants in the phloem with no evidence for xylem transport (Moreno et al. 2004). While CMV may not translocate through the xylem and therefore not directly affect spittlebugs, the virus may affect xylem flow through changes in transpiration, resulting from disease symptoms in leaves. Infection of Chinese cabbage plants by Turnip yellow mosaic virus, for example, lowered the responsiveness of stomata to light intensity causing a reduction in transpiration (Bedbrook and Matthews 1973).
Genetic variation among M. guttatus plants in the effects of CMV infection on host plant quality for spittlebugs could make it difficult to predict the effect of this pathogen on spittlebug population dynamics in nature. In some M. guttatus populations, pathogen infection may have little effect on spittlebug performance and subsequent spittlebug population growth. This may be the case in population M13 where CMV infection altered host plant quality in <20% of the M. guttatus families we studied. In other populations, however, pathogen infection may have pronounced effects on spittlebug performance and population dynamics. This may be the case in population M5 where CMV infection of M. guttatus plants increased spittlebug performance in almost one-half the families we studied. Our work suggests that detailed studies of individual populations may be needed to accurately predict the ecological and evolutionary consequences of pathogen infection for herbivorous insects in naturally occurring plant species (Gilbert 2002).
In conclusion, this is one of the first studies to show that the effect of pathogen infection on host plant quality for herbivorous insects varies among plant genotypes. The results of this study suggest that future work on the mechanisms and consequences of pathogen infection of plants for insect herbivores is likely to vary within and among plant populations and this variation should be explored in other plant systems.
We thank S. Blackwell, B. Clark, Z. DeLamar, J. Eubanks, C. Ivey, C. Harvey, H. Hull-Sanders, I. Kaplan, and M. Patrick for assistance in the field, greenhouse, and/or laboratory. Point Reyes National Seashore, the Wantrup Wildlife Sanctuary, and the Midpeninsula Open Space Preserve kindly provided field sites and accommodations for collecting in California. This work was supported by National Science Foundation Grants DEB-0075225 to M.D.E., DEB-0074556 to D.E.C., REU supplements to both grants, the Department of Entomology and Plant Pathology at Auburn University, and the University of Virginia's Blandy Experimental Farm.
References