-
PDF
- Split View
-
Views
-
Cite
Cite
Félix Martínez, Martina Carrete, Guillermo Blanco, Spatial, social, and environmental factors influencing natal dispersal in the colonial griffon vulture, Current Zoology, Volume 71, Issue 2, April 2025, Pages 170–183, https://doi.org/10.1093/cz/zoae037
- Share Icon Share
Abstract
Natal dispersal is a critical trait for individual fitness and the viability, structure, and genetic identity of populations. However, there is a pronounced information gap for large and long-lived species due to the difficulty of monitoring individuals at appropriate spatio-temporal scales. Here we study how individual traits and social and environmental characteristics influence natal dispersal decisions of griffon vultures (Gyps fulvus) using long-term (30 years) monitoring of a large number of individuals marked as nestlings in Spain. Our results show a strong philopatry in both sexes, with some individuals recruiting as breeders on the same cliffs, and even the same nests, where they were born. This philopatric tendency was modulated by the effect of conspecific density on individual parameters and emphasized the importance of conspecific attraction and changes in food availability that may have influenced the increment in colony size and the colonization of new areas. Although further research is needed considering smaller colonies and more isolated population nuclei, our results highlight the importance of long-term studies on long-lived species to understand the factors that determine their population dynamics and their relationship with anthropogenic activities, whose effects should be predicted and managed using conservation criteria.
Natal philopatry (i.e., the tendency of an individual to return to its natal area to reproduce) and hence natal dispersal distance (i.e., the distance between an individual’s birthplace and that of its first reproductive attempt) are related traits that are critical for individual survival and reproduction and for population viability, structure, and genetic identity (Greenwood and Harvey 1982). Philopatry can confer benefits to individuals, derived from prior knowledge of the natal area, such as enhanced survival and reproductive success (Beletsky and Orians 1991; Forrester et al. 2015). However, multiple other individual, environmental, and social factors, which may differ between populations and geographic regions, can modulate philopatry, pushing some individuals to recruit in areas other than the natal area (Pusey 1987; Luna et al. 2020; Serrano et al. 2021). Knowledge of the spatial scale and timing of these traits, and the factors that modulate them, is paramount to understanding the processes governing wildlife populations and implementing appropriate conservation measures (Serrano 2018).
In birds, most species have a mating system based on the male defense of resources, so males tend to be more philopatric than females, reducing inbreeding (Perrin and Goudet 2001; Serrano et al. 2003). The cost of foraging in areas far from the natal area may also be higher for males, thus favoring females to find more distant breeding sites (Perrin and Goudet, 2001; Kingma et al. 2017). Previous studies have also shown that individual condition, measured as body mass/size ratio, physiological state, and other traits related to health status may affect natal philopatry–and thus dispersal distances–with apparently variable and even contradictory outcomes (Delgado et al. 2010). Some authors have found that some individuals may disperse longer distances without increasing mortality risk, while others have found that certain internal and environmental conditions force individuals to disperse longer distances due to high intraspecific competition (Clobert et al. 2009). Besides, dispersal decisions can be influenced by, among other things, food availability and phenology of individuals during development in the nest or after acquiring breeding status, and these conditions can, in turn, influence the condition and specific phenotypic features and fitness of offspring (Bonte et al. 2012; Azpillaga et al. 2018; Serrano 2018). However, philopatry ultimately depends on vacancies to be filled in the natal area, so population size and habitat saturation around nesting areas, or colonies in the case of social species, can influence dispersal decisions of individuals by triggering density-dependent processes that reduce reproductive or survival rates (Clobert et al. 2009). These processes are generally related to direct competition for resources, but also to other social interactions between individuals, both agonistic and mutualistic (Bonte et al. 2012; Serrano 2018). Thus, high conspecific densities at nesting areas can force first-time breeders, who are often young and inexperienced, to seek more distant resources or new breeding sites to reduce competition (Bonte et al. 2012; Cardador et al. 2012; Serrano 2018). Conversely, depending on intrinsic characteristics of individuals, such as age, status, sex, and genetic identity, or the social characteristics of the species, conspecific density may act as an attractant, favoring the settlement of more individuals in its vicinity and promoting philopatry (Sergio and Penteriani 2005; Nocera et al. 2006; Luna et al. 2020).
For many bird species, natal dispersal patterns and their association with individual, population, and environmental variables are unknown. This information gap is most pronounced for large and long-lived species, such as vultures and other large raptors with deferred maturity and delayed first breeding, due to the difficulty of monitoring individual vital parameters over representative time periods at geographic scales large enough to encompass their movements (Campbell 2015). This knowledge is fundamental for understanding the population dynamics and genetic structure of these species (Le Gouar et al. 2008; Blanco and Morinha 2021; Rousteau et al. 2022), thus contributing to their management and conservation.
Here, we studied natal dispersal patterns of griffon vultures (Gyps fulvus) through long-term monitoring (i.e., 30 years) of a large number of individuals marked as nestlings in Spain. Published information on vulture natal dispersal patterns is scarce and insufficient to assess their role in conservation, despite this group being one of the most endangered worldwide (Campbell 2015). The griffon vulture is the most abundant obligate avian scavenger in the western Palaearctic and is therefore key to the functioning of its ecosystems (Cortés-Avizanda et al. 2015). In Western Europe, griffon vulture populations have grown in recent decades, especially in Spain, where they have increased tenfold in the last 40 years. This increase is due to both an increase in the number of breeding pairs in or near existing colonies and the formation of new colonies in previously unoccupied areas (del Moral and Molina 2018; Almaraz et al. 2022). Despite its importance for population dynamics and geographic expansion, there is little information on the individual, social, and environmental factors that influence natal dispersal in this species. Our main hypothesis is that due to the high social behavior of the species, individuals may prefer to settle in their natal areas, especially when the number of conspecifics is high (conspecific attraction) and when vacant nesting sites are available. Therefore, density may be an important attractor for recruitment if colonies of different densities and nesting site availability exist within the population. However, the specific traits of individuals, birth date corresponding to early or late fledglings, and the environmental and social conditions of their natal colony could influence their competitive abilities and thus the decision to try to settle in the natal colony or disperse to other colonies of different sizes or even to vacant localities corresponding to the former range of the species, or to areas of new colonization. Griffon vultures are very long-lived birds that begin to breed at variable ages (usually≥4 years-old). Older or more experienced individuals, that is, those with greater competitive ability, could be more likely to recruit in the natal area, displacing younger and less experienced individuals to more distant colonies. Given that both sexes contribute similarly to parental tasks (Xirouchakis and Mylonas 2007; Yaniv-Feller et al. 2018; but see Martínez et al. 2024a), we do not expect sexual differences in philopatry. Finally, we examined the effect of food availability on natal dispersal patterns. Vertebrate scavengers are highly dependent on anthropogenic subsidies from livestock production (Cortés-Avizanda et al. 2016), and are therefore affected by changes associated with this human activity. In the early 2000s, the Bovine Spongiform Encephalopathy disease (hereafter referred to as the mad cow crisis) led to a drastic reduction in scavenger feeding in Europe due to health regulations that limited the abandonment of cattle carcasses (Donázar et al. 2009; Blanco, 2014). These restrictions have had profound effects on the population dynamics of griffon vultures and other scavengers (Almaraz et al. 2022), although the effect of these resource changes on natal dispersal is unknown. We expected that the reduction in food availability associated with the mad cow crisis would affect the dispersal pattern of vultures both at birth, through a reduction in individual quality, and during recruitment, favoring the exploration of larger areas and increasing the probability of settlement at more distant locations.
Materials and Methods
Study species and area
The griffon vulture is a large (8–10 kg) monomorphic obligate scavenger that breeds colonially on rocky cliffs (Chakarov and Blanco 2021). Vultures can live up to 30 years in the wild and begin breeding at 4–6 years of age, although some individuals can show breeding behavior as early as 3 years, while others delay their first breeding attempt to more than 10 years (Sarrazin et al., 1996; Chantepie et al. 2016; Fargallo et al. 2018). In general, individuals do not lay eggs during their first breeding attempts, but only mate and occupy nesting sites (Blanco and Martínez 1996; 1997). The breeding season begins in December with nest building, copulations, and pair formation. Eggs are laid between late December and mid-March, although some pairs may lay replacement clutches until early May (Martínez et al. 1997, 1998; Almaraz et al. 2022). Nestlings hatch between mid-February and early May, and the fledging period lasts from mid-June to early September. Young vultures begin to leave the breeding colonies in September, a phenomenon that becomes widespread in October and November, with very few juveniles remaining at breeding sites during the winter months and at the beginning of the following year’s breeding season (December to March).
Juveniles travel long distances during their first years of life, many of them migrating to Africa through the Strait of Gibraltar, where the number of birds crossing has increased in recent decades in line with population growth (Onrubia 2021). The spring migration through the Strait of Gibraltar occurs between February and June, with a more limited number of individuals, mostly (67.6%) in their second year (Ramírez et al. 2019). These vultures tend to concentrate in large numbers in areas that are sometimes far from their birthplaces or with very few breeding colonies nearby, but where food is abundant (Delgado-González et al. 2022). After these movements, individuals two or more years old return to the breeding colonies, although they continue to lead a very erratic life, traveling over large areas of the Iberian Peninsula until they settle as breeders (Zuberogoitia et al. 2013; Martínez et al. 2024b).
The study was carried out mainly in the breeding colony located in and around the Hoces del río Riaza (province of Segovia, north-central Spain). The climate of the area is continental Mediterranean, with long, dry, and cold winters and hot but short summers, with a rainfall of 400–500 mm/year (Cano 1990). Griffon vultures nest in caves and ledges in limestone cliffs along a 12 km stretch of the Riaza river and surrounding ravines (hereafter referred to as the focal colony). In 1975 the area was declared a Raptor Refuge, managed by WWF/Adena, and in 2004 the regional government declared it as Natural Park. In this area, the availability of extensive livestock carcasses (mainly sheep, available at the place of their death in the field) has been radically reduced, coinciding with the decline of sheep herds in recent decades (Blanco 2014), and vultures are highly dependent on stabled livestock carcasses, mainly pigs and poultry, whose carrion is only available at predictable feeding points such as vulture restaurants and farms (changes in the spatio-temporal distribution and predictability of food; Cortés-Avizanda et al. 2016). The number of breeding pairs in this colony has grown from 170 pairs in 1984 to 716 in 2020 (Almaraz et al. 2022). This trend is similar to that of the species in most of the Iberian Peninsula. In Spain, griffon vultures have increased from 3,240 breeding pairs in 1979 to 30,946-37,134 breeding pairs (about 100,000 individuals, including non-breeding birds) in 2018 (del Moral et al. 2018).
Fieldwork procedures
The monitoring of the focal colony began in 1984 and intensified between 1994 and 2020, with at least 5 complete annual censuses between January and June following standardized methods (Martínez et al. 1997). During these censuses, all the rocky areas suitable for nesting of the species were covered on foot, with observations made at a certain distance (usually 300–500m) to avoid disturbing breeding individuals. In each case, the presence of individuals copulating, incubating and/or with nestlings was recorded, confirming in all cases whether or not these nestlings fledged (breeding success; Fargallo et al. 2018; Almaraz et al. 2022). The location of each nest was recorded vertically by GPS. The different cliffs in which the nests were located were delimited according to their continuity and orientation.
From 1990 to 2020, vulture nestlings were ringed in the nests when they were almost fully grown, at an age of ca. 50–80 days. A total of 452 nestlings in the focal colony were marked with metal and PVC rings engraved with unique alphanumeric codes readable at distance, weighed, and measured following standard protocols (López-Rull et al. 2015). Wing length (to the nearest mm) was used to estimate the age of individuals (Gómez-López et al. 2023) and to back-calculate their birth date (Julian day), which was complemented with observational criteria such as the first recording of the egg, the start of incubation and the presence of hatchlings, and considering an incubation period of 55 days (Elosegui 1989). Body condition was estimated as the residuals of a standardized major axis regression of log10 of weight (in g) against log10 of structural body size (tarsus length in mm; type-2 standardized major axis regression, SMA; Peig and Green 2010). Some of these nestlings were also bled (0.5 mL from the brachial vein) to determine their sex by molecular methods (Wink et al. 1998; Fridolfsson and Ellegren 1999), while others were sexed years later, when they entered the breeding population, by observing their copulation position (Gómez-López et al. 2023).
Nestlings from other colonies were also ringed to assess natal dispersal to the focal colony (Figure 1). We considered that nests belonged to the same colony when they were separated by less than 1 km, following the criterion usually used in national censuses. For this purpose, we selected nearby colonies (20–100 km from the focal colony) in the provinces of Segovia and Ávila (Central Spain) and more distant colonies (more than 100 km from the focal colony) in the provinces of Álava, Cantabria, Huesca, Teruel (Northern Spain), Badajoz (Western Spain), and Cádiz (Southern Spain). We completed this information with records of nestlings ringed with PCV bands in Spain by other researchers or naturalists and later resighted in Spain (observations collected in the database of the Estación Biológica de Doñana, EBD-CSIC; http://anillamiento.ebd.csic.es/IniciarAction.do), France (O. Duriez, pers. com.) or elsewhere.

Distribution of locations where nestling griffon vultures have been tagged with rings readable at distance until 2020 in the distribution area of the species in Spain and France. The size of points is proportional to the number of nestlings marked with PVC bands in each location. Map was elaborated with QGIS (Quantum Geographic Information System) v.3.22.7-Białowieża.
From 1993, when the first marked nestlings in the focal colony were old enough to breed (3 years old), we started to look for marked individuals in the nests during the annual censuses carried out in the focal colony until 2020. The search for marked individuals was most intensive during the first months of the breeding season (December–March) when vultures start nesting, copulating, and laying eggs, and both members of the pair are therefore very active near their nesting sites. To locate marked individuals in other colonies, different breeding localities were visited throughout the known range of the species in Spain (February–April) of 1997–1998, 2007–2008, and 2017–2018. The number of breeding individuals observed during each of these surveys was used as an estimate of the search effort for marked individuals outside the focal colony. Each colony was monitored once in each one of the study periods, with the same colonies surveyed in each period (Supplementary Figure S1).
Statistical analysis
We used generalized linear models (GLM) to assess the effects of individual traits (sex, body condition, birth date, and age at recruitment) and social (number of breeding pairs in the natal colony at the time of birth and recruitment of each individual) and environmental variables (food availability, influenced by the mad cow crisis) on the natal dispersal pattern of griffon vultures. For this purpose, we considered philopatry (logit link function, binomial error distribution) at 2 complementary scales, namely: 1) philopatry to the natal colony (i.e., individuals recruiting to the focal colony -where they were born, regardless of the distance between the natal nest and the nest of the first breeding attempt), and 2) philopatry to the natal cliff (i.e., individuals recruiting to the cliff where they were born, regardless of the distance between the natal nest and the nest of the first breeding attempt). Because the vast majority of individuals were recruited as breeders at the natal colony (see results), the natal dispersal distances of these individuals cannot exceed the maximum distance between the geographic boundaries of the colony (12 km). Thus, the influence of being or not philopatric to the natal colony clearly conditions the dispersal distance and therefore this variable could be redundant, uninformative, or biased depending on the scale of the analysis. However, we also analyzed the factors influencing dispersal distance (log-transformed, identity link function, normal error distribution), following the same analytical procedure used for philopatry, to explore potential fine-scale patterns in natal dispersal decisions, but mainly because philopatric individuals greatly outnumbered non-philopatric ones, potentially biasing previous analyses. Natal dispersal distance was calculated as the distance (in m) between the natal nest and the nest of the first breeding attempt.
As information on sex and body condition was available for a subsample of individuals, we first tested the effects of all variables using this dataset. Multivariate models without sex and body condition as explanatory variables were then repeated to include all marked individuals. The effects of changes in food availability were included in models as a factor with three levels corresponding to the main stages of the mad cow crisis: before (1990–2001, when food availability was high and mainly based on dispersed carrions in the countryside), during (2002–2011, when dispersed food in the countryside decreased drastically) and after (from 2012 onward), when food availability gradually increased but its distribution became aggregated and predictable due to the creation of numerous feeding stations and the collapse of the extensive livestock herd (Blanco 2014). We differentiate between the availability of food during the nestling phase and the recruitment phase. All continuous variables were centered and standardized before modeling. We used Tukey’s post hoc tests to compare levels of categorical variables (lsmeans package; Lenth 2016). Model selection was performed using the Akaike Information Criterion corrected for small sample sizes (AICc; Burnham and Anderson 2002), including the null model and discarding those with convergence problems. Models within 2 AICc units of the best model were considered as alternatives, and explanatory variables included were considered to have a significant effect on the dependent variable based on their associated P-value. We calculated the variance explained by each alternative model as the percentage of deviance explained. Statistical analyses were conducted in R 3.1.2.
Finally, because the factors influencing natal dispersal may interact in complex ways, we integrated the relative importance of the variables using structural equation modeling (SEM). SEM resolves multivariate relationships among a set of interrelated variables by linking multiple predictors and response variables into a single causal network. By allowing a variable to serve as a response in a path and a predictor in another, SEM is useful for testing and quantifying indirect or cascading effects that would otherwise go unnoticed in a single model (Grace 2006). As not all of our variables are normally distributed, we used piecewise SEM (piecewise SEM package; Lefcheck 2016) to set up structured linear equations with different distributions depending on the endogenous variable (philopatry: binomial error distribution, logit link function; body condition, birth date and age of first breeding: Gaussian error distribution, identity link function; number of breeding pairs: Poisson error distribution, log link function). Variables were included in models affecting the endogenous variables. Shipley’s test of directed separation was used to evaluate the assumption that all variables are conditionally independent (i.e., no missing relationships between unconnected variables; Shipley, 2000). The significance of all independence statements was combined to estimate Fisher’s C, which was then compared with a χ2 distribution with 2*k (where k is the number of independent statements) degrees of freedom. Suggested links were added to improve SEM fit. Hypothesized relationships were considered consistent with the data if there was weak support for the sum of conditional independence statements (P < 0.05). The proportion of variance explained by the SEM was estimated as R2 values derived from the variance of the fixed effects (Nakagawa and Schielzeth 2013).
Results
Natal dispersal
A total of 1,393 vulture nestlings were ringed with PVC bands in Spain until 2020, of which 452 were born in the focal colony (32.4%), 204 in nearby colonies (20–100 km from the focal colony; 14.6%), and 737 in colonies more than 100 km away (52.9%) (Fig. 1, Supplementary Table S1). Besides, 2,857 nestlings were also marked in France between 1982 and 2020 (656 in the Pyrenees, 1,496 in the Massif Central, and 705 in the Alps). The geographical distribution of all ringed nestlings considered in this study is shown in Figure 1.
Of the 452 nestlings ringed at the focal colony, 13 were marked in the last 3 years of the study (and therefore would not be old enough to be registered as breeders) and 13 lost their PVC ring before the minimum recruitment age (confirmed by reading the metal ring with telescopes from hiding places at feeding sites or by recapturing them). Taking into account survival estimates calculated for this same population using a multievent capture-mark-recapture framework (first-year vultures: 0.69; 2-, and 3-year-old vultures: 0.93; Gómez-López et al. in review), of the 254 individuals that could have reached the minimum age for recruitment, 144 were recruited at the focal colony (57% of philopatric individuals) and 10 (4%) were recorded as breeders at least once during the study period at one of the sampled colonies. Most individuals breed for the first time at around 6 years of age (n = 154 individuals), with cases up to 14 years. Most of the recruited individuals were male (83 out of the 132 individuals of known sex; binomial test: P = 0.002) and settled in the focal colony (79 males and 46 females; binomial test: P = 0.002) or in nearby colonies (5 males out of 7 individuals of known sex). The only individual recruited in a distant colony was a female. Recapture probabilities were high (0.76, SE: 0.01; Gómez-López et al. in review), which gives us confidence that we were able to detect most of the marked birds.
The number of marked individuals from the focal colony detected as breeders at the colony and their proportion over those sampled in each temporal period are given in Table 1. Note that the marked nestlings detected as breeding at the focal colony in each period represent a subset of the total monitored each year throughout the study period (see above) and that some marked individuals were located in more than one of the sampling periods. Nine individuals marked as nestling in the focal colony were recruited as breeders in nearby colonies in the different sampling periods (Table 1a; one nestling banded in 1996 in the focal colony was recorded as a breeder in the same nearby colony in 2 of the sampling periods). This represented approximately 0.6% of the individuals monitored in the nearby colonies (n = 1,631 breeding griffon vultures; Table 1a), and a 4% of the nestlings banded in the focal colony at or above the recruitment age (see above). After checking 3,860 breeding griffon vultures in distant colonies, none had been marked as a nestling in the focal colony (Table 1a; see Supplementary Figure S1 for the geographical distribution of sampling effort and the number of marked individuals found to be breeding in the different colonies). However, one individual born in the focal colony was recorded by other observers (EBD database) as a breeder in a distant colony (0.4% of the total number of nestlings banded in the focal colony). This individual, which settled 190 km NW of the focal colony, could be monitored during a single failed breeding attempt when it was 6 years old (born in 2000 and observed in 2006) and was found dead 8 months later in northern Spain, at 155 km from the locality where it had been seen breeding. It is worth noting that this high rate of recruitment in the natal colony (philopatry to the colony) is not due to the inability of individuals to move to distant colonies. In fact, during surveys to detect marked individuals throughout the range of the species, we observed 50 individuals born in the focal colony at other colonies or at feeding and roosting sites, mainly in the west and southwest of the Iberian Peninsula (Figure 2). In addition, four individuals from the focal colony were found dead in Africa (2 in Algeria and 2 in Senegal) and one was recovered in Poland after having been seen twice before in Catalonia, northeast Spain (Figure 2). A total of 6 individuals marked as nestlings in colonies other than the focal one were observed breeding in their respective natal localities (i.e., philopatric individuals), 3 in nearby colonies (province of Segovia), and the other 3 in distant colonies (provinces of Teruel, Badajoz, and Cádiz).
(a) Number of griffon vultures marked as nestlings (n vultures marked) and recruited as breeders in the colony of Hoces del río Riaza (focal colony) and in nearby (located in a range of 20–100 km) and distant (more than 100 km) colonies over the total number of vultures observed (n vultures observed) in each one during the monitoring carried out between 1997 and 2018. (b) Number of breeding griffon vultures marked as nestlings (n breeders marked) in the focal colony and recruited in the focal, nearby, and distant colonies over the total number of vultures marked at the focal colony (n vultures marked at the focal colony) up to each monitoring year. Note that the marked nestlings detected as breeders in the focal colony in each period represent a part of the total number of individuals monitored each year during the study period and that some marked individuals were located in more than one of the sampling periods. All the data presented in this Table corresponds to individuals marked as nestling in the focal colony.
(a) n breeders marked/n observed breeders (%) . | ||||
---|---|---|---|---|
Sampling period . | All periods . | |||
1997–1998 . | 2007–2008 . | 2017–2018 . | ||
Focal colony | 40/486 (8.2) | 71/674 (10.5) | 49/941 (5.2) | 160/2101 (7.6) |
Nearby colonies | 2/552 (0.4) | 4/563 (0.7) | 5/516 (1.0) | 10/1631 (0.6) |
Distant colonies | 0/1436 (0.0) | 0/1244 (0.0) | 0/1180 (0.0) | 0/3860 (0.0) |
Total | 42/2474 (1.7) | 75/2481 (3.0) | 54/2637 (2.0) | 170/7592 (2.2) |
(a) n breeders marked/n observed breeders (%) . | ||||
---|---|---|---|---|
Sampling period . | All periods . | |||
1997–1998 . | 2007–2008 . | 2017–2018 . | ||
Focal colony | 40/486 (8.2) | 71/674 (10.5) | 49/941 (5.2) | 160/2101 (7.6) |
Nearby colonies | 2/552 (0.4) | 4/563 (0.7) | 5/516 (1.0) | 10/1631 (0.6) |
Distant colonies | 0/1436 (0.0) | 0/1244 (0.0) | 0/1180 (0.0) | 0/3860 (0.0) |
Total | 42/2474 (1.7) | 75/2481 (3.0) | 54/2637 (2.0) | 170/7592 (2.2) |
(b) n breeders marked/n nestlings marked in the focal colony (%) . | ||||
---|---|---|---|---|
Sampling period . | All periods . | |||
1997–1998 . | 2007–2008 . | 2017–2018 . | ||
Focal colony | 40/169 (23.6) | 71/321 (22.1) | 49/429 (11.4) | 133/429(31.0) |
Nearby colonies | 2/169 (1.2) | 4/321 (1.2) | 5/429 (1.2) | 10/429 (2.3) |
Distant colonies | 0/169 (0.0) | 0/321 (0.0) | 0/429 (0.0) | 0/429 (0.0) |
Total | 42/169 (24.8) | 75/321 (23.3) | 54/429(12.6) | 143/429 (33.3) |
(b) n breeders marked/n nestlings marked in the focal colony (%) . | ||||
---|---|---|---|---|
Sampling period . | All periods . | |||
1997–1998 . | 2007–2008 . | 2017–2018 . | ||
Focal colony | 40/169 (23.6) | 71/321 (22.1) | 49/429 (11.4) | 133/429(31.0) |
Nearby colonies | 2/169 (1.2) | 4/321 (1.2) | 5/429 (1.2) | 10/429 (2.3) |
Distant colonies | 0/169 (0.0) | 0/321 (0.0) | 0/429 (0.0) | 0/429 (0.0) |
Total | 42/169 (24.8) | 75/321 (23.3) | 54/429(12.6) | 143/429 (33.3) |
(a) Number of griffon vultures marked as nestlings (n vultures marked) and recruited as breeders in the colony of Hoces del río Riaza (focal colony) and in nearby (located in a range of 20–100 km) and distant (more than 100 km) colonies over the total number of vultures observed (n vultures observed) in each one during the monitoring carried out between 1997 and 2018. (b) Number of breeding griffon vultures marked as nestlings (n breeders marked) in the focal colony and recruited in the focal, nearby, and distant colonies over the total number of vultures marked at the focal colony (n vultures marked at the focal colony) up to each monitoring year. Note that the marked nestlings detected as breeders in the focal colony in each period represent a part of the total number of individuals monitored each year during the study period and that some marked individuals were located in more than one of the sampling periods. All the data presented in this Table corresponds to individuals marked as nestling in the focal colony.
(a) n breeders marked/n observed breeders (%) . | ||||
---|---|---|---|---|
Sampling period . | All periods . | |||
1997–1998 . | 2007–2008 . | 2017–2018 . | ||
Focal colony | 40/486 (8.2) | 71/674 (10.5) | 49/941 (5.2) | 160/2101 (7.6) |
Nearby colonies | 2/552 (0.4) | 4/563 (0.7) | 5/516 (1.0) | 10/1631 (0.6) |
Distant colonies | 0/1436 (0.0) | 0/1244 (0.0) | 0/1180 (0.0) | 0/3860 (0.0) |
Total | 42/2474 (1.7) | 75/2481 (3.0) | 54/2637 (2.0) | 170/7592 (2.2) |
(a) n breeders marked/n observed breeders (%) . | ||||
---|---|---|---|---|
Sampling period . | All periods . | |||
1997–1998 . | 2007–2008 . | 2017–2018 . | ||
Focal colony | 40/486 (8.2) | 71/674 (10.5) | 49/941 (5.2) | 160/2101 (7.6) |
Nearby colonies | 2/552 (0.4) | 4/563 (0.7) | 5/516 (1.0) | 10/1631 (0.6) |
Distant colonies | 0/1436 (0.0) | 0/1244 (0.0) | 0/1180 (0.0) | 0/3860 (0.0) |
Total | 42/2474 (1.7) | 75/2481 (3.0) | 54/2637 (2.0) | 170/7592 (2.2) |
(b) n breeders marked/n nestlings marked in the focal colony (%) . | ||||
---|---|---|---|---|
Sampling period . | All periods . | |||
1997–1998 . | 2007–2008 . | 2017–2018 . | ||
Focal colony | 40/169 (23.6) | 71/321 (22.1) | 49/429 (11.4) | 133/429(31.0) |
Nearby colonies | 2/169 (1.2) | 4/321 (1.2) | 5/429 (1.2) | 10/429 (2.3) |
Distant colonies | 0/169 (0.0) | 0/321 (0.0) | 0/429 (0.0) | 0/429 (0.0) |
Total | 42/169 (24.8) | 75/321 (23.3) | 54/429(12.6) | 143/429 (33.3) |
(b) n breeders marked/n nestlings marked in the focal colony (%) . | ||||
---|---|---|---|---|
Sampling period . | All periods . | |||
1997–1998 . | 2007–2008 . | 2017–2018 . | ||
Focal colony | 40/169 (23.6) | 71/321 (22.1) | 49/429 (11.4) | 133/429(31.0) |
Nearby colonies | 2/169 (1.2) | 4/321 (1.2) | 5/429 (1.2) | 10/429 (2.3) |
Distant colonies | 0/169 (0.0) | 0/321 (0.0) | 0/429 (0.0) | 0/429 (0.0) |
Total | 42/169 (24.8) | 75/321 (23.3) | 54/429(12.6) | 143/429 (33.3) |
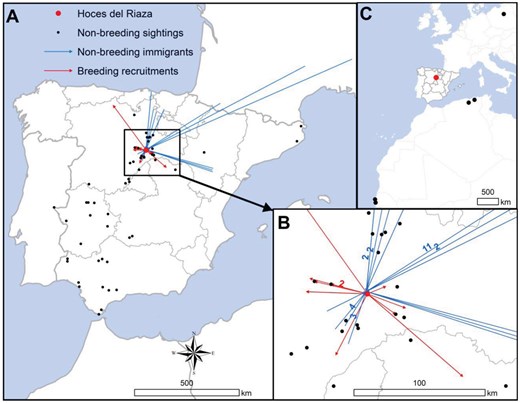
(A) Location of the focal colony (Hoces del río Riaza), and sightings of marked non-breeding individuals in that colony (black dots). The blue lines represent dispersals to the focal colony of non-breeding immigrants marked in other colonies, while the red lines represent natal dispersals of individuals marked in the focal colony and observed as breeders in other colonies. (B) Details of the above observations over an enlarged area around the focal colony, corresponding to the 20–100 km buffer that defines the “nearby colonies.” The numbers represent the number of individuals to which the observations correspond. (C) Recoveries in northern Africa and northern Europe of nestlings marked at the focal colony. Maps were elaborated with QGIS (Quantum Geographic Information System) v.3.22.7-Białowieża.
Foreign individuals observed in the focal colony
During the breeding seasons 1993–2020, we observed (on 44 occasions, on different dates) 32 non-breeding individuals marked as nestlings in other colonies in the focal colony (Fig. 2). Of these, 14 individuals were born in colonies in France, 9 from colonies in northern and eastern Spain (6 from different localities of the provinces of Álava and Cantabria, and 3 from colonies in the province of Teruel), and 9 from nearby colonies (province of Segovia). Outside the breeding season (September-December), these individuals were also observed in the focal colony on 27 occasions (61.4 % of the total). These observations generally corresponded to juveniles and sub-adults less than 5 years old (29 observations, 65.9 % of the total). None of these individuals showed breeding behavior during their stay at the focal colony. No individuals born in southern colonies were observed at the focal colony (Fig. 2).
Factors affecting philopatry to the natal colony and cliff
Overall, vultures showed high colony philopatry (all individuals recruited in the focal colony were born there, whereas a small proportion were recruited in other colonies, see above). A proportion (6.0%) of individuals recruited at the focal colony even recruited to the same natal cliff, including 3 individuals that recruited at their natal nests (1 male and 2 females). These patterns were the same for males and females (colony philopatry: 93.83% and 93.88%, respectively, χ2 = 0.0001, df = 1, P = 0.9908; cliff philopatry: 7.41% and 6.12%, respectively, χ2 = 0.08, df = 1, P = 0.7789).
The models obtained for the subsample of individuals for which we know sex and body condition (n = 31 individuals) showed no significant effects of any of the variables considered on colony or cliff philopatry (Supplementary Tables S2 and S3). However, when including all individuals in the dataset (n = 148 individuals), we found significant effects of food availability in the year of recruitment and intraspecific competition in the year of birth on the probability of a vulture settling to breed in its natal colony (colony philopatry; Table 2). Note that some recruited individuals were not included in the analyses because the coordinate of the nest of origin in the focal colony was not recorded during banding. In general, philopatry to the colony was higher for individuals born when the colony had more breeding pairs, although changes in food availability during and after the mad cow crisis favored dispersal to other colonies, with the recruitment to the natal colony occurring when it had a greater number of breeding pairs (Figure 3). One of the alternative models suggested that some individual characteristics were also related to the probability of recruitment to the natal colony. Individuals born later in the breeding season and those recruiting at an older age showed lower philopatry than those born earlier and recruited at a younger age (Figure 3). When analyzing the factors influencing the probability of an individual becoming a breeder on its natal cliff, we found a positive effect of conspecific density, that is, philopatry to the natal cliff was higher for vultures born in years with more breeding pairs in the colony. Moreover, individuals tended to recruit to their natal cliff when these cliffs have a larger number of breeding pairs (Table 3).
The first 10 models obtained to assess the relative importance of individual traits (birth: birth date and age at recruitment) and of social (NcolB and NcolR: number of breeding pairs in the natal colony at the time of birth and recruitment of each individual, respectively) and environmental variables (MCowB and MCowR: food availability at the time of birth and recruitment of each individual, as influenced by the mad cow crisis) on the probability that a griffon vulture recruits to its natal colony. The significance of the variables included in the alternative models (∆ AICc < 2) and their percentage of deviance explained (%) are shown. k: number of estimated parameters, AICc: Akaike information criteria with small sample bias adjustment, ∆ AICc: difference between each model and the best model (i.e., the model with the lowest AICc), weight: Akaike weight, df: degrees of freedom. Estimates (and their SE, standard errors) are provided only for significant continuous variables (P < 0.05)
Model selection . | Alternative models . | ||||||||||
---|---|---|---|---|---|---|---|---|---|---|---|
Model . | k . | AICc . | ∆AICc . | w . | Variables . | χ2 . | df . | P . | Estimate . | SE . | % . |
MCowR + NcolB | 4 | 73.88 | 0.00 | 0.14 | MCowR | 9.83 | 2 | 0.0073 | 16.26 | ||
birth + MCowR + NcolB | 5 | 74.37 | 0.49 | 0.11 | NcolB | 4.56 | 1 | 0.0328 | 1.68 | 0.78 | |
birth + age | 3 | 74.59 | 0.71 | 0.10 | birth | 1.62 | 1 | 0.2027 | 18.37 | ||
birth + MCowR + NcolB + NcolR | 6 | 74.68 | 0.80 | 0.09 | MCowR | 7.14 | 2 | 0.0281 | |||
MCowR + NcolB + NcolR | 5 | 75.01 | 1.13 | 0.08 | NcolB | 4.26 | 1 | 0.0390 | 1.68 | 0.81 | |
MCowR + age + NcolB | 5 | 75.94 | 2.05 | 0.05 | birth | 6.11 | 1 | 0.0135 | −0.68 | 0.27 | 12.67 |
birth + MCowR + age + NcolB | 6 | 76.54 | 2.66 | 0.04 | age | 4.66 | 1 | 0.0309 | −0.58 | 0.27 | |
birth + age + NcolR | 4 | 76.67 | 2.79 | 0.03 | birth | 2.39 | 1 | 0.1219 | 20.75 | ||
birth + age + NcolB | 4 | 76.68 | 2.79 | 0.03 | MCowR | 8.54 | 2 | 0.0140 | |||
birth + MCowR + age + NcolR | 6 | 76.81 | 2.93 | 0.03 | NcolB | 3.84 | 1 | 0.0502 | 1.65 | 0.84 | |
NcolR | 1.75 | 1 | 0.1860 |
Model selection . | Alternative models . | ||||||||||
---|---|---|---|---|---|---|---|---|---|---|---|
Model . | k . | AICc . | ∆AICc . | w . | Variables . | χ2 . | df . | P . | Estimate . | SE . | % . |
MCowR + NcolB | 4 | 73.88 | 0.00 | 0.14 | MCowR | 9.83 | 2 | 0.0073 | 16.26 | ||
birth + MCowR + NcolB | 5 | 74.37 | 0.49 | 0.11 | NcolB | 4.56 | 1 | 0.0328 | 1.68 | 0.78 | |
birth + age | 3 | 74.59 | 0.71 | 0.10 | birth | 1.62 | 1 | 0.2027 | 18.37 | ||
birth + MCowR + NcolB + NcolR | 6 | 74.68 | 0.80 | 0.09 | MCowR | 7.14 | 2 | 0.0281 | |||
MCowR + NcolB + NcolR | 5 | 75.01 | 1.13 | 0.08 | NcolB | 4.26 | 1 | 0.0390 | 1.68 | 0.81 | |
MCowR + age + NcolB | 5 | 75.94 | 2.05 | 0.05 | birth | 6.11 | 1 | 0.0135 | −0.68 | 0.27 | 12.67 |
birth + MCowR + age + NcolB | 6 | 76.54 | 2.66 | 0.04 | age | 4.66 | 1 | 0.0309 | −0.58 | 0.27 | |
birth + age + NcolR | 4 | 76.67 | 2.79 | 0.03 | birth | 2.39 | 1 | 0.1219 | 20.75 | ||
birth + age + NcolB | 4 | 76.68 | 2.79 | 0.03 | MCowR | 8.54 | 2 | 0.0140 | |||
birth + MCowR + age + NcolR | 6 | 76.81 | 2.93 | 0.03 | NcolB | 3.84 | 1 | 0.0502 | 1.65 | 0.84 | |
NcolR | 1.75 | 1 | 0.1860 |
The first 10 models obtained to assess the relative importance of individual traits (birth: birth date and age at recruitment) and of social (NcolB and NcolR: number of breeding pairs in the natal colony at the time of birth and recruitment of each individual, respectively) and environmental variables (MCowB and MCowR: food availability at the time of birth and recruitment of each individual, as influenced by the mad cow crisis) on the probability that a griffon vulture recruits to its natal colony. The significance of the variables included in the alternative models (∆ AICc < 2) and their percentage of deviance explained (%) are shown. k: number of estimated parameters, AICc: Akaike information criteria with small sample bias adjustment, ∆ AICc: difference between each model and the best model (i.e., the model with the lowest AICc), weight: Akaike weight, df: degrees of freedom. Estimates (and their SE, standard errors) are provided only for significant continuous variables (P < 0.05)
Model selection . | Alternative models . | ||||||||||
---|---|---|---|---|---|---|---|---|---|---|---|
Model . | k . | AICc . | ∆AICc . | w . | Variables . | χ2 . | df . | P . | Estimate . | SE . | % . |
MCowR + NcolB | 4 | 73.88 | 0.00 | 0.14 | MCowR | 9.83 | 2 | 0.0073 | 16.26 | ||
birth + MCowR + NcolB | 5 | 74.37 | 0.49 | 0.11 | NcolB | 4.56 | 1 | 0.0328 | 1.68 | 0.78 | |
birth + age | 3 | 74.59 | 0.71 | 0.10 | birth | 1.62 | 1 | 0.2027 | 18.37 | ||
birth + MCowR + NcolB + NcolR | 6 | 74.68 | 0.80 | 0.09 | MCowR | 7.14 | 2 | 0.0281 | |||
MCowR + NcolB + NcolR | 5 | 75.01 | 1.13 | 0.08 | NcolB | 4.26 | 1 | 0.0390 | 1.68 | 0.81 | |
MCowR + age + NcolB | 5 | 75.94 | 2.05 | 0.05 | birth | 6.11 | 1 | 0.0135 | −0.68 | 0.27 | 12.67 |
birth + MCowR + age + NcolB | 6 | 76.54 | 2.66 | 0.04 | age | 4.66 | 1 | 0.0309 | −0.58 | 0.27 | |
birth + age + NcolR | 4 | 76.67 | 2.79 | 0.03 | birth | 2.39 | 1 | 0.1219 | 20.75 | ||
birth + age + NcolB | 4 | 76.68 | 2.79 | 0.03 | MCowR | 8.54 | 2 | 0.0140 | |||
birth + MCowR + age + NcolR | 6 | 76.81 | 2.93 | 0.03 | NcolB | 3.84 | 1 | 0.0502 | 1.65 | 0.84 | |
NcolR | 1.75 | 1 | 0.1860 |
Model selection . | Alternative models . | ||||||||||
---|---|---|---|---|---|---|---|---|---|---|---|
Model . | k . | AICc . | ∆AICc . | w . | Variables . | χ2 . | df . | P . | Estimate . | SE . | % . |
MCowR + NcolB | 4 | 73.88 | 0.00 | 0.14 | MCowR | 9.83 | 2 | 0.0073 | 16.26 | ||
birth + MCowR + NcolB | 5 | 74.37 | 0.49 | 0.11 | NcolB | 4.56 | 1 | 0.0328 | 1.68 | 0.78 | |
birth + age | 3 | 74.59 | 0.71 | 0.10 | birth | 1.62 | 1 | 0.2027 | 18.37 | ||
birth + MCowR + NcolB + NcolR | 6 | 74.68 | 0.80 | 0.09 | MCowR | 7.14 | 2 | 0.0281 | |||
MCowR + NcolB + NcolR | 5 | 75.01 | 1.13 | 0.08 | NcolB | 4.26 | 1 | 0.0390 | 1.68 | 0.81 | |
MCowR + age + NcolB | 5 | 75.94 | 2.05 | 0.05 | birth | 6.11 | 1 | 0.0135 | −0.68 | 0.27 | 12.67 |
birth + MCowR + age + NcolB | 6 | 76.54 | 2.66 | 0.04 | age | 4.66 | 1 | 0.0309 | −0.58 | 0.27 | |
birth + age + NcolR | 4 | 76.67 | 2.79 | 0.03 | birth | 2.39 | 1 | 0.1219 | 20.75 | ||
birth + age + NcolB | 4 | 76.68 | 2.79 | 0.03 | MCowR | 8.54 | 2 | 0.0140 | |||
birth + MCowR + age + NcolR | 6 | 76.81 | 2.93 | 0.03 | NcolB | 3.84 | 1 | 0.0502 | 1.65 | 0.84 | |
NcolR | 1.75 | 1 | 0.1860 |
The first 10 models were obtained to assess the relative importance of individual traits (birth: birth date and age: age at recruitment) and of social (NcolB and NcolR: number of breeding pairs in the natal colony at the time of birth and recruitment of each individual, respectively; and NcliffB and NcliffR: number of breeding pairs in the natal cliff at the time of birth and recruitment of each individual, respectively) and environmental variables (MCowB and MCowR: food availability at the time of birth and recruitment of each individual, as influenced by the mad cow crisis) on the probability that a griffon vulture recruits to its natal cliff. The significance of the variables included in the alternative models (∆ AICc < 2) and their percentage of deviance explained (%) are shown. k: number of estimated parameters, AICc: Akaike information criteria with small sample bias adjustment, ∆ AICc: difference between each model and the best model (i.e., the model with the lowest AICc), weight: Akaike weight, df: degrees of freedom. Estimates (and their SE, standard errors) are provided only for significant continuous variables (P < 0.05)
Model selection . | Alternative models . | ||||||||||
---|---|---|---|---|---|---|---|---|---|---|---|
Model . | k . | AICc . | ∆AICc . | w . | Variables . | χ2 . | df . | P . | Estimate . | SE . | % . |
NcliffR + NcolB | 3 | 64.65 | 0.00 | 0.05 | NcliffR | 2.88 | 1 | 0.0895 | 11.95 | ||
NcolB | 2 | 65.44 | 0.79 | 0.04 | NcolB | 3.12 | 1 | 0.0775 | |||
NcliffR | 2 | 65.67 | 1.02 | 0.03 | NcolB | 5.05 | 1 | 0.0246 | 0.75 | 0.34 | 7.61 |
NcliffR + NcolR | 3 | 66.35 | 1.70 | 0.02 | NcliffR | 4.82 | 1 | 0.0281 | 0.68 | 0.30 | 7.26 |
NcliffB + NcliffR | 3 | 66.38 | 1.73 | 0.02 | NcliffR | 2.32 | 1 | 0.1278 | 9.39 | ||
NcliffR + Age + NcolB | 4 | 66.39 | 1.74 | 0.02 | NcolR | 1.42 | 1 | 0.2342 | |||
NcolR | 2 | 66.58 | 1.93 | 0.02 | NcliffB | 1.39 | 1 | 0.2384 | 9.35 | ||
NcliffR + NcolB + NcolR | 4 | 66.58 | 1.93 | 0.02 | NcliffR | 4.25 | 1 | 0.0392 | 0.63 | 0.30 | |
NcliffR + Birth + NcolB | 4 | 66.64 | 1.99 | 0.02 | NcliffR | 2.70 | 1 | 0.1005 | 12.53 | ||
NcliffR + NcliffB + NcolB | 4 | 66.77 | 2.12 | 0.02 | Age | 0.38 | 1 | 0.5366 | |||
NcolB | 3.20 | 1 | 0.0736 | ||||||||
NcolR | 3.92 | 1 | 0.0478 | 0.55 | 0.26 | 5.90 | |||||
NcliffR | 3.00 | 1 | 0.0831 | 12.24 | |||||||
NcolB | 1.89 | 1 | 0.1687 | ||||||||
NcolR | 0.19 | 1 | 0.6602 | ||||||||
NcliffR | 2.95 | 1 | 0.0857 | 12.14 | |||||||
Birth | 0.13 | 1 | 0.7208 | ||||||||
NcolB | 2.45 | 1 | 0.1177 |
Model selection . | Alternative models . | ||||||||||
---|---|---|---|---|---|---|---|---|---|---|---|
Model . | k . | AICc . | ∆AICc . | w . | Variables . | χ2 . | df . | P . | Estimate . | SE . | % . |
NcliffR + NcolB | 3 | 64.65 | 0.00 | 0.05 | NcliffR | 2.88 | 1 | 0.0895 | 11.95 | ||
NcolB | 2 | 65.44 | 0.79 | 0.04 | NcolB | 3.12 | 1 | 0.0775 | |||
NcliffR | 2 | 65.67 | 1.02 | 0.03 | NcolB | 5.05 | 1 | 0.0246 | 0.75 | 0.34 | 7.61 |
NcliffR + NcolR | 3 | 66.35 | 1.70 | 0.02 | NcliffR | 4.82 | 1 | 0.0281 | 0.68 | 0.30 | 7.26 |
NcliffB + NcliffR | 3 | 66.38 | 1.73 | 0.02 | NcliffR | 2.32 | 1 | 0.1278 | 9.39 | ||
NcliffR + Age + NcolB | 4 | 66.39 | 1.74 | 0.02 | NcolR | 1.42 | 1 | 0.2342 | |||
NcolR | 2 | 66.58 | 1.93 | 0.02 | NcliffB | 1.39 | 1 | 0.2384 | 9.35 | ||
NcliffR + NcolB + NcolR | 4 | 66.58 | 1.93 | 0.02 | NcliffR | 4.25 | 1 | 0.0392 | 0.63 | 0.30 | |
NcliffR + Birth + NcolB | 4 | 66.64 | 1.99 | 0.02 | NcliffR | 2.70 | 1 | 0.1005 | 12.53 | ||
NcliffR + NcliffB + NcolB | 4 | 66.77 | 2.12 | 0.02 | Age | 0.38 | 1 | 0.5366 | |||
NcolB | 3.20 | 1 | 0.0736 | ||||||||
NcolR | 3.92 | 1 | 0.0478 | 0.55 | 0.26 | 5.90 | |||||
NcliffR | 3.00 | 1 | 0.0831 | 12.24 | |||||||
NcolB | 1.89 | 1 | 0.1687 | ||||||||
NcolR | 0.19 | 1 | 0.6602 | ||||||||
NcliffR | 2.95 | 1 | 0.0857 | 12.14 | |||||||
Birth | 0.13 | 1 | 0.7208 | ||||||||
NcolB | 2.45 | 1 | 0.1177 |
The first 10 models were obtained to assess the relative importance of individual traits (birth: birth date and age: age at recruitment) and of social (NcolB and NcolR: number of breeding pairs in the natal colony at the time of birth and recruitment of each individual, respectively; and NcliffB and NcliffR: number of breeding pairs in the natal cliff at the time of birth and recruitment of each individual, respectively) and environmental variables (MCowB and MCowR: food availability at the time of birth and recruitment of each individual, as influenced by the mad cow crisis) on the probability that a griffon vulture recruits to its natal cliff. The significance of the variables included in the alternative models (∆ AICc < 2) and their percentage of deviance explained (%) are shown. k: number of estimated parameters, AICc: Akaike information criteria with small sample bias adjustment, ∆ AICc: difference between each model and the best model (i.e., the model with the lowest AICc), weight: Akaike weight, df: degrees of freedom. Estimates (and their SE, standard errors) are provided only for significant continuous variables (P < 0.05)
Model selection . | Alternative models . | ||||||||||
---|---|---|---|---|---|---|---|---|---|---|---|
Model . | k . | AICc . | ∆AICc . | w . | Variables . | χ2 . | df . | P . | Estimate . | SE . | % . |
NcliffR + NcolB | 3 | 64.65 | 0.00 | 0.05 | NcliffR | 2.88 | 1 | 0.0895 | 11.95 | ||
NcolB | 2 | 65.44 | 0.79 | 0.04 | NcolB | 3.12 | 1 | 0.0775 | |||
NcliffR | 2 | 65.67 | 1.02 | 0.03 | NcolB | 5.05 | 1 | 0.0246 | 0.75 | 0.34 | 7.61 |
NcliffR + NcolR | 3 | 66.35 | 1.70 | 0.02 | NcliffR | 4.82 | 1 | 0.0281 | 0.68 | 0.30 | 7.26 |
NcliffB + NcliffR | 3 | 66.38 | 1.73 | 0.02 | NcliffR | 2.32 | 1 | 0.1278 | 9.39 | ||
NcliffR + Age + NcolB | 4 | 66.39 | 1.74 | 0.02 | NcolR | 1.42 | 1 | 0.2342 | |||
NcolR | 2 | 66.58 | 1.93 | 0.02 | NcliffB | 1.39 | 1 | 0.2384 | 9.35 | ||
NcliffR + NcolB + NcolR | 4 | 66.58 | 1.93 | 0.02 | NcliffR | 4.25 | 1 | 0.0392 | 0.63 | 0.30 | |
NcliffR + Birth + NcolB | 4 | 66.64 | 1.99 | 0.02 | NcliffR | 2.70 | 1 | 0.1005 | 12.53 | ||
NcliffR + NcliffB + NcolB | 4 | 66.77 | 2.12 | 0.02 | Age | 0.38 | 1 | 0.5366 | |||
NcolB | 3.20 | 1 | 0.0736 | ||||||||
NcolR | 3.92 | 1 | 0.0478 | 0.55 | 0.26 | 5.90 | |||||
NcliffR | 3.00 | 1 | 0.0831 | 12.24 | |||||||
NcolB | 1.89 | 1 | 0.1687 | ||||||||
NcolR | 0.19 | 1 | 0.6602 | ||||||||
NcliffR | 2.95 | 1 | 0.0857 | 12.14 | |||||||
Birth | 0.13 | 1 | 0.7208 | ||||||||
NcolB | 2.45 | 1 | 0.1177 |
Model selection . | Alternative models . | ||||||||||
---|---|---|---|---|---|---|---|---|---|---|---|
Model . | k . | AICc . | ∆AICc . | w . | Variables . | χ2 . | df . | P . | Estimate . | SE . | % . |
NcliffR + NcolB | 3 | 64.65 | 0.00 | 0.05 | NcliffR | 2.88 | 1 | 0.0895 | 11.95 | ||
NcolB | 2 | 65.44 | 0.79 | 0.04 | NcolB | 3.12 | 1 | 0.0775 | |||
NcliffR | 2 | 65.67 | 1.02 | 0.03 | NcolB | 5.05 | 1 | 0.0246 | 0.75 | 0.34 | 7.61 |
NcliffR + NcolR | 3 | 66.35 | 1.70 | 0.02 | NcliffR | 4.82 | 1 | 0.0281 | 0.68 | 0.30 | 7.26 |
NcliffB + NcliffR | 3 | 66.38 | 1.73 | 0.02 | NcliffR | 2.32 | 1 | 0.1278 | 9.39 | ||
NcliffR + Age + NcolB | 4 | 66.39 | 1.74 | 0.02 | NcolR | 1.42 | 1 | 0.2342 | |||
NcolR | 2 | 66.58 | 1.93 | 0.02 | NcliffB | 1.39 | 1 | 0.2384 | 9.35 | ||
NcliffR + NcolB + NcolR | 4 | 66.58 | 1.93 | 0.02 | NcliffR | 4.25 | 1 | 0.0392 | 0.63 | 0.30 | |
NcliffR + Birth + NcolB | 4 | 66.64 | 1.99 | 0.02 | NcliffR | 2.70 | 1 | 0.1005 | 12.53 | ||
NcliffR + NcliffB + NcolB | 4 | 66.77 | 2.12 | 0.02 | Age | 0.38 | 1 | 0.5366 | |||
NcolB | 3.20 | 1 | 0.0736 | ||||||||
NcolR | 3.92 | 1 | 0.0478 | 0.55 | 0.26 | 5.90 | |||||
NcliffR | 3.00 | 1 | 0.0831 | 12.24 | |||||||
NcolB | 1.89 | 1 | 0.1687 | ||||||||
NcolR | 0.19 | 1 | 0.6602 | ||||||||
NcliffR | 2.95 | 1 | 0.0857 | 12.14 | |||||||
Birth | 0.13 | 1 | 0.7208 | ||||||||
NcolB | 2.45 | 1 | 0.1177 |
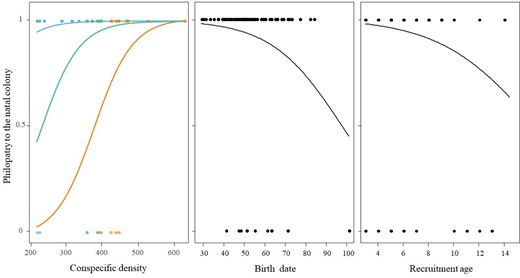
Effects of food availability (estimated using the 3 main stages of the mad cow crisis as a surrogate): before (blue symbols): 1990–2001, food availability was high; during (green symbols: 2002–2011, food dramatically dropped for vultures, and after (orange symbols): 2012 onwards, food availability increased progressively) and conspecific density (measured as the number of breeding pairs in the natal colony) in the year of recruitment, birth date and age at recruitment on the probability that a griffon vulture settling in its natal colony.
The SEMs revealed complex relationships between our variables (Table 4). On the one hand, changes in food availability associated with the mad cow crisis had a direct effect on philopatry by increasing the proportion of vultures dispersing outside the breeding colony in recent years, after the mad cow crisis. Food availability also had an indirect effect on philopatry by delaying breeding phenology after the mad cow crisis, with vultures born earlier in the breeding season showing greater philopatry to the colony than those born later. Colony size and food availability also influenced the age of vulture recruitment, with recruitment delayed after the mad cow crisis as the number of breeding pairs increased. However, age of recruitment was not directly related to philopatry. SEM performed at the cliff-scale showed similar relationships between the variables, but no clear effects of any variable on philopatry (Fig. 4).
Structural equation model (SEM) was obtained to relate individual traits (birth: birth date and age: age at recruitment) and social (NcolB and NcolR: number of breeding pairs in the natal colony at the time of birth and recruitment of each individual, respectively; and NcliffB and NcliffR: number of breeding pairs in the natal cliff at the time of birth and recruitment of each individual, respectively) and environmental variables (MCowB and MCowR: food availability at the time of birth and recruitment of each individual, as influenced by the mad cow crisis) to the probability that a griffon vulture recruits to its natal colony and cliff. Standardized estimates (Estimates) are provided only for continuous variables
Philopatry to the colony . | Philopatry to the cliff . | ||||||||
---|---|---|---|---|---|---|---|---|---|
Response . | Predictor . | Estimate . | df . | χ2 . | P . | Estimate . | df . | χ2 . | P . |
Philopatry | Birth | −0.23 | 139 | −2.03 | 0.0422 | ||||
MCowR | 2 | 3.10 | 0.0483 | ||||||
Birth | MCowB | 2 | 22.82 | <0.0001 | 2 | 14.15 | <0.0001 | ||
Age | NcolB | −0.92 | 141 | −6.19 | <0.0001 | −0.98 | 129 | −5.59 | <0.0001 |
NcolR | 0.41 | 141 | 2.75 | 0.0067 | 0.65 | 129 | 3.24 | 0.0015 | |
MCowR | 2 | 23.38 | <0.0001 | 2 | 15.86 | <0.0001 | |||
NcolB | MCowB | 2 | 62.54 | <0.0001 | 2 | 60.27 | <0.0001 | ||
NcolR | NcolB | 0.01 | 142 | 2.21 | 0.0270 | 0.01 | 133 | 7.10 | <0.0001 |
MCowB | 2 | 10.84 | <0.0001 | ||||||
MCowR | 2 | 57.72 | <0.0001 | 2 | 79.56 | <0.0001 | |||
NcliffB | NcolB | 0.03 | 133 | 21.81 | <0.0001 | ||||
MCowB | 2 | 6.60 | 0.0018 | ||||||
NcliffR | NcolB | 0.00 | 132 | −2.25 | 0.0246 | ||||
NcolR | 0.01 | 132 | 3.76 | 0.0002 |
Philopatry to the colony . | Philopatry to the cliff . | ||||||||
---|---|---|---|---|---|---|---|---|---|
Response . | Predictor . | Estimate . | df . | χ2 . | P . | Estimate . | df . | χ2 . | P . |
Philopatry | Birth | −0.23 | 139 | −2.03 | 0.0422 | ||||
MCowR | 2 | 3.10 | 0.0483 | ||||||
Birth | MCowB | 2 | 22.82 | <0.0001 | 2 | 14.15 | <0.0001 | ||
Age | NcolB | −0.92 | 141 | −6.19 | <0.0001 | −0.98 | 129 | −5.59 | <0.0001 |
NcolR | 0.41 | 141 | 2.75 | 0.0067 | 0.65 | 129 | 3.24 | 0.0015 | |
MCowR | 2 | 23.38 | <0.0001 | 2 | 15.86 | <0.0001 | |||
NcolB | MCowB | 2 | 62.54 | <0.0001 | 2 | 60.27 | <0.0001 | ||
NcolR | NcolB | 0.01 | 142 | 2.21 | 0.0270 | 0.01 | 133 | 7.10 | <0.0001 |
MCowB | 2 | 10.84 | <0.0001 | ||||||
MCowR | 2 | 57.72 | <0.0001 | 2 | 79.56 | <0.0001 | |||
NcliffB | NcolB | 0.03 | 133 | 21.81 | <0.0001 | ||||
MCowB | 2 | 6.60 | 0.0018 | ||||||
NcliffR | NcolB | 0.00 | 132 | −2.25 | 0.0246 | ||||
NcolR | 0.01 | 132 | 3.76 | 0.0002 |
Structural equation model (SEM) was obtained to relate individual traits (birth: birth date and age: age at recruitment) and social (NcolB and NcolR: number of breeding pairs in the natal colony at the time of birth and recruitment of each individual, respectively; and NcliffB and NcliffR: number of breeding pairs in the natal cliff at the time of birth and recruitment of each individual, respectively) and environmental variables (MCowB and MCowR: food availability at the time of birth and recruitment of each individual, as influenced by the mad cow crisis) to the probability that a griffon vulture recruits to its natal colony and cliff. Standardized estimates (Estimates) are provided only for continuous variables
Philopatry to the colony . | Philopatry to the cliff . | ||||||||
---|---|---|---|---|---|---|---|---|---|
Response . | Predictor . | Estimate . | df . | χ2 . | P . | Estimate . | df . | χ2 . | P . |
Philopatry | Birth | −0.23 | 139 | −2.03 | 0.0422 | ||||
MCowR | 2 | 3.10 | 0.0483 | ||||||
Birth | MCowB | 2 | 22.82 | <0.0001 | 2 | 14.15 | <0.0001 | ||
Age | NcolB | −0.92 | 141 | −6.19 | <0.0001 | −0.98 | 129 | −5.59 | <0.0001 |
NcolR | 0.41 | 141 | 2.75 | 0.0067 | 0.65 | 129 | 3.24 | 0.0015 | |
MCowR | 2 | 23.38 | <0.0001 | 2 | 15.86 | <0.0001 | |||
NcolB | MCowB | 2 | 62.54 | <0.0001 | 2 | 60.27 | <0.0001 | ||
NcolR | NcolB | 0.01 | 142 | 2.21 | 0.0270 | 0.01 | 133 | 7.10 | <0.0001 |
MCowB | 2 | 10.84 | <0.0001 | ||||||
MCowR | 2 | 57.72 | <0.0001 | 2 | 79.56 | <0.0001 | |||
NcliffB | NcolB | 0.03 | 133 | 21.81 | <0.0001 | ||||
MCowB | 2 | 6.60 | 0.0018 | ||||||
NcliffR | NcolB | 0.00 | 132 | −2.25 | 0.0246 | ||||
NcolR | 0.01 | 132 | 3.76 | 0.0002 |
Philopatry to the colony . | Philopatry to the cliff . | ||||||||
---|---|---|---|---|---|---|---|---|---|
Response . | Predictor . | Estimate . | df . | χ2 . | P . | Estimate . | df . | χ2 . | P . |
Philopatry | Birth | −0.23 | 139 | −2.03 | 0.0422 | ||||
MCowR | 2 | 3.10 | 0.0483 | ||||||
Birth | MCowB | 2 | 22.82 | <0.0001 | 2 | 14.15 | <0.0001 | ||
Age | NcolB | −0.92 | 141 | −6.19 | <0.0001 | −0.98 | 129 | −5.59 | <0.0001 |
NcolR | 0.41 | 141 | 2.75 | 0.0067 | 0.65 | 129 | 3.24 | 0.0015 | |
MCowR | 2 | 23.38 | <0.0001 | 2 | 15.86 | <0.0001 | |||
NcolB | MCowB | 2 | 62.54 | <0.0001 | 2 | 60.27 | <0.0001 | ||
NcolR | NcolB | 0.01 | 142 | 2.21 | 0.0270 | 0.01 | 133 | 7.10 | <0.0001 |
MCowB | 2 | 10.84 | <0.0001 | ||||||
MCowR | 2 | 57.72 | <0.0001 | 2 | 79.56 | <0.0001 | |||
NcliffB | NcolB | 0.03 | 133 | 21.81 | <0.0001 | ||||
MCowB | 2 | 6.60 | 0.0018 | ||||||
NcliffR | NcolB | 0.00 | 132 | −2.25 | 0.0246 | ||||
NcolR | 0.01 | 132 | 3.76 | 0.0002 |
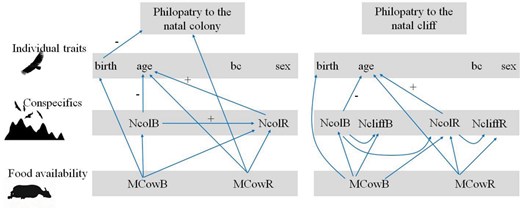
Structural equation model (SEM) exploring the relationships between individual traits (sex, bc: body condition, birth: birth date and age: age at recruitment) and social (NcolB and NcolR: number of breeding pairs in the natal colony at the time of birth and recruitment of each individual, respectively, and NcliffB and NcliffR: number of breeding pairs in the natal cliff at the time of birth and recruitment of each individual, respectively) and environmental variables (MCowB and MCowR: food availability at the time of birth and recruitment of each individual, as influenced by the mad cow crisis) to the probability that a griffon vulture recruits to its natal colony and cliff. Arrows represent unidirectional significant paths among variables (P < 0.05). For continuous variables, the signs of the significant relationships are provided (+ and −: positive and negative relationships, respectively). For details on SEM output, see Table 4.
Dispersal distance
The median natal dispersal distance for all recruited individuals was 2.64 km (range = 0–190 km, n = 148 individuals), with similar values for males (median = 2.35 km, range = 0–51 km, n = 81 individuals) and females (median = 2.65 km, range = 0–190 km, n = 49 individuals) (χ2 = 0.29, df = 1, P = 0.5916). For individuals recruited to the focal colony, males (median = 2.16, range = 0–11.39 km, n = 76 individuals) and females (median = 2.38 km, range = 0–10.53 km, n = 44 individuals) also settled at similar distances from their natal nests (χ2 = 0.40, df = 1, P = 0.5295), whereas the dispersal distance of individuals recruited in other colonies (median = 41 km, range = 20–190 km, n = 10 individuals) was greater for females (median = 41 km, range = 25 - 190 km, n = 3 individuals) than for males (median = 44 km, range = 27–51 km, n = 5 individuals). In the latter case, the small number of individuals recorded (especially females) and the fact that the only long-distance recruitment was a female do not allow a proper statistical comparison between the sexes.
In models built using the subset of individuals for which sex and body condition were available (i.e., all those recruited to the focal colony), we found shorter dispersal distances for vultures born after the mad cow crisis and when the colony had fewer breeding pairs (Supplementary Table S4). A similar pattern emerged for the whole dataset (excluding sex and body condition as explanatory variables). In general, dispersing individuals tended to recruit in areas with high numbers of conspecifics using two alternative strategies: staying close to the natal area when the number of breeding pairs is high or, when not possible, moving to other large colonies further away (Supplementary Table S5). It is worth mentioning that, for individuals recruiting at the focal colony, there is a negative relationship between age and dispersal distance, with older vultures recruiting closer than younger ones.
Finally, SEMs suggested a combined effect of changes in food availability due to the mad cow crisis, colony size, and age at recruitment on natal dispersal distances. The vultures that dispersed farthest were the youngest individuals, possibly born after the mad cow crisis when the focal colony already had numerous breeding pairs (Supplementary Figure S2).
Discussion
We show strong natal philopatry in griffon vultures. Over three decades, all marked individuals that were observed breeding at our focal colony were born there. This was not due to a lack of marked nestling in other nearby or distant colonies and geographical regions, as the number of marked nestlings throughout the breeding range of the species has been relatively high and continuous for decades. Indeed, the number of marked nestlings in the focal colony represents only 11% of the total number of marked nestlings in Spain and France, and individuals from other colonies and regions have been observed in the focal colony, but have never shown breeding behavior. The high philopatry and low dispersal distances observed were also unlikely due to sampling bias, as the search effort for breeding individuals was similar in nearby colonies and even higher in colonies located more than 100 km away. In the three surveys that we conducted over the species’ extensive breeding range in Spain, several thousand breeding vultures were observed, but only a small number of individuals marked as nestling in our focal colony recruited as breeders in nearby colonies (<0.2% of marked individuals). Importantly, of the total number of individuals marked as nestlings in our focal colony, 144 were recorded as breeding there, representing almost 60% of philopatric individuals. In contrast, only 4% of nestlings banded in the focal colony that reached the minimum age for recruitment dispersed to other colonies. These percentages imply a very high detection rate of recruited individuals, considering the pre-reproductive mortality (Almaraz et al. 2022; Gómez-López et al. in review). In fact, our data of 16 nestlings marked in the study area with GPS devices show that mortality in the first 2 years of life alone is at least 44% (authors’ unpublished data). Thus, we are confident that we have detected the vast majority of recruited individuals. Finally, although we have considered that the minimum age for recruitment is 3 years, most individuals monitored during our study breed for the first time later (at around 6 years of age). Thus, our estimates of philopatry are conservative and would increase if corrected by the age of individuals (and the age-specific survival of individuals). The strong philopatry shown by griffon vultures is also reflected in the tendency of some individuals to breed on the same cliffs and even the same nests where they were born. Although the proportion of these fine-scale philopatric individuals is relatively small, the mere existence of this unlikely possibility in such a large colony suggests a strong individual imprint on the natal place. The sparse data on marked nestlings recruited to other colonies supports this low dispersal rate, as all breeding records of marked individuals occurred in their natal areas. This suggests that this philopatric behavior occurs throughout the breeding range of the species and is a general feature of the life history of griffon vultures.
Recruits in both focal and distant colonies show a bias towards males, even though the sex-ratio of nestlings is balanced (Gómez-López et al. 2023), suggesting a higher mortality of females in the pre-breeding stage (Gómez-López et al. in review). Thus, the number of females would be a limiting factor for the population and could explain their earlier reproductive age compared with males (Blanco and Martínez, 1996). Although our work may have limitations due to the extensive sampling of part of the breeding area of the species, both males and females show similar philopatric behavior, which would not derive from a sex bias in the recruited individuals. The few individuals recruited outside the focal colony dispersed in all directions, mainly to nearby colonies (20–100 km). These destination colonies were often in areas of recent colonization (del Moral et al. 2018), so they could be growing due to immigration from larger or saturated colonies, such as our focal one. In this colony, only vultures born in colonies located further north have been observed, although all these records correspond to non-breeding or southward-migrating individuals. Despite the efforts made over the years to mark the southern populations, particularly in Andalusia, and the intensive monitoring carried out during both the breeding and non-breeding seasons, the absence of marked individuals from these colonies in our focal colony is significant. This pattern has been corroborated with vultures tracked with GPS transmitters from different Spanish regions, which have shown that the home range of individuals is restricted to the surroundings of their breeding colonies, with few movements towards the north, especially for individuals from colonies located in the south of the Iberian Peninsula (Morant et al. 2023). Nevertheless, some marked individuals occasionally reach localities in the north of the Iberian Peninsula and, exceptionally, in central Europe. These results suggest some spatial structuring of the populations, which may be related to the southward dispersal of both trans-Saharan migrants (Onrubia, 2021) and sub-adult individuals in their pre-reproductive stage (Delgado-González et al. 2022). GPS tracking of nestlings from different colonies is needed to determine the extent to which this pattern of movements, with a clear south-southeast component and limited north-northwest movements, can be generalized. This technology can also help to confirm the philopatric behavior of the species, provided that the lifespan of transmitters reaches the age of first reproduction.
The median dispersal distance of griffon vultures born in the focal colony (ca. 3 km) is much shorter than that recorded for other vulture species, such as the semi-colonial cinereous vultures (Dobado et al. 2012) or the territorial Egyptian (Neophron percnopterus) (Serrano et al. 2021) and bearded vulture (Gypaetus barbatus) (Margalida et al. 2005; López-López et. al. 2013). The natal dispersal distances obtained for these species are similar to those for individuals dispersing outside our focal colony, suggesting that colonial breeding may favor philopatry. Indeed, in the colonial Cape vulture (Gyps coprotheres), individuals also return to their natal colonies to breed, although data are too sparse to draw firm conclusions about the patterns and drivers of philopatry (Piper 1994; Hirschauer et al. 2017). Overall, this suggests that the philopatry exhibited by colonial vultures may have evolved in association with the social exploitation of dispersed and unpredictable food (van Overveld et al. 2020).
Regardless of the evolutionary mechanisms that cause it (McNamara and Dall 2011), philopatry has many benefits for individuals, which would explain its widespread occurrence among birds (Greenwood and Harvey 1982; Paradis et al. 1998). However, social and environmental factors can determine differences in the degree of philopatry exhibited by individuals within a population (Serrano 2018). In the case of griffon vultures, our results show that conspecific density and food availability are important variables that interact in a complex way to influence the probability of recruitment of individuals to the natal colony and cliff. The mad cow crisis broke out in Europe in the early 2000s and led to health regulations restricting the abandonment of livestock carcasses in the field (Donázar et al. 2009; Almaraz et al. 2022), which drastically reduced food availability for scavengers (ca. 80%; Donázar et al. 2009). From 2012, more flexible regulations on the use of livestock carcasses for scavengers were implemented (EC 142/2011 and RD 1632/2011), gradually increasing food availability (Blanco 2014; Almaraz et al. 2022; Morales-Reyes et al. 2016). While food has followed a high-low-high pattern in terms of quantity, one of the most important changes following the mad cow crisis was that the distribution of food became highly spatially concentrated. On the one hand, several vulture restaurants were opened near scavenging areas, where large amounts of food (mainly carcasses from intensive livestock farming) were dumped, while at the same time, the amount of food dispersed over the countryside decreased due to the abandonment of extensive livestock farming. In addition, the quality of the food has also changed, especially in recent years, as a result of the increased availability of carcasses from intensive livestock farming, which transfers huge amounts of pharmaceutical drugs to the vultures, with as yet unknown consequences for their fitness (Blanco et al. 2024).
The focal colony responded to the reduction in food availability during the mad cow crisis by changing the age structure of the breeding fraction (i.e., the proportion of breeding sub-adults was substantially reduced) and by decreasing breeding success. However, adult survival was not affected, which could have promoted some accumulation of individuals that did not reproduce during the mad cow crisis, but incorporated into the breeding population with a rapid recovery of reproductive parameters once EU health policies authorized the abandonment of carcasses in the field again (Almaraz et al. 2022). This also allowed the population to recover from the positive trend that started years before the mad cow crisis (Martínez et al., 1997; Almaraz et al. 2022). Thus, the increase in conspecific density after the mad cow crisis may have favored the dispersal of individuals to other colonies due to habitat saturation. In particular, young breeders could be more motivated to disperse after the mad cow crisis to newly formed colonies with fewer competitors (Duriez et al. 2012), Furthermore, philopatry appears to be affected by the number of breeding pairs on each cliff, supporting conspecific attraction at small spatial scales (cliff and nest). Although negative density-dependent effects on reproduction have been detected in the focal colony (Almaraz et al. 2022), nest sites were still available throughout the study period (i.e., more than twice as many nest sites were vacant in 2020, the last year of monitoring). This highlights the importance of the aggregate distribution of nest sites as a determinant of colonial nesting, modulated by conspecific attraction even at small spatial scales (natal cliff and nest), and the preference for specific known sites for reproduction. It is also possible that the significant decline in extensive livestock farming, together with food aggregation in vulture restaurants after the mad cow crisis (Blanco 2014), may have also influenced the movement of non-breeding individuals, allowing their recruitment to colonies other than the natal one.
Changes in food availability also had an indirect effect on philopatry by delaying breeding phenology during the mad cow crisis (Almaraz et al. 2022). Food shortage may force individuals to forage for longer periods and over larger areas to reach breeding conditions, thereby delaying laying dates (Williams, 2012). Delaying the laying date in the breeding season can affect the birth date and then nestling condition by causing a mismatch between their needs and food availability or by affecting the time available for parental care (Martínez et al. 1998). Although our results showed no effect of body condition on griffon vulture dispersal patterns, nestlings born earlier in the breeding season would achieve better body condition than those born later (Lindström 1999; Ronget et al. 2018). Thus, individuals born earlier may be more competitive to recruit into the natal colony in situations of high density of conspecifics if birth date may have an influence on condition with long-term effects on individual fitness (“silver spoon” effect; Van De Pol et al., 2006), while those born later would be forced to disperse to other colonies (Bonte et al. 2012; Serrano 2018). Finally, food availability also modulated the age at which individuals were recruited throughout its effect on the number of breeding pairs in the colony. The effort required by breeding vultures to raise a nestling is enormous (four months of parental care and about 9 months for the entire reproductive process), so delaying the age of recruitment would allow individuals to acquire a dominance status sufficient to occupy a breeding site in the natal colony, which could be perceived as of high quality by birds born there (Sergio and Penteriani 2005; Nocera et al. 2006). Younger individuals, on the other hand, may not recruit to a saturated colony until they acquire the necessary experience and skills, so they may disperse to smaller colonies (Bonte et al. 2012; Cardador et al. 2012; Serrano 2018). Our results do not allow us to be conclusive in this sense, as we find a negative trend between age at recruitment and philopatry, which is no longer significant when we simultaneously analyze the possible causalities between the variables (SEM models). Further research is needed to assess the consequences of philopatry on recruitment age and the fitness of griffon vultures, which is extremely complicated as it requires individual monitoring of survival and reproduction throughout the life of philopatric and dispersing individuals. In addition, natal dispersal should be ideally studied together with survival through multi-event capture-recapture models (see Rousteau et al. 2022).
There is no previous information on natal dispersal patterns in the griffon vulture, despite being such an abundant and widespread species in the Western Palearctic. Therefore, this study contributes to the understanding of how natal dispersal decisions can govern the population dynamics and demography of this key scavenger. Due to the high availability of nesting sites, the philopatry and natal dispersal distances obtained in this study may differ from the situation in other colonies. In fact, our focal colony is one of the largest in Spain due to the high availability of cliffs and food supplied in vulture restaurants. In smaller colonies, dispersal distances would be longer, depending on the availability of nesting sites and the carrying capacity of the environment (i.e., food abundance). More research on smaller colonies and more isolated populations is needed to better understand the dispersal patterns of this species in other contexts and on a global scale. This information, obtained from long-term, multi-scale studies, should be combined with that already available to provide a holistic understanding of the factors that determine the population dynamics of this long-lived species sensitive to human activities, the effects of which we should try to anticipate and manage for conservation purposes.
Supplementary Material
Supplementary material can be found at https://dbpia.nl.go.kr/cz.
Acknowledgments
We thank Ó. Frías, J.L. Gonzalez del Barrio, J. M. Garcia, B. Arroyo, A. González, J. Garcia, G. Doval, J. Griesinger, J. Hernando, and J.F. Martín by their help in the ringing over the years. We thank P. Salinas helped with data management, and J.A. Cuevas for the elaboration of maps. A. Seguí (FAPAS), C. Fernández, and O. Duriez kindly gave us their data on nestlings marked in Alicante, Guipúzcoa-Navarra, and France, respectively. Junta de Castilla- León authorized the ringing from 1990 to the present, and the Management of the Parque Natural de las Hoces del Riaza offered facilities to work in the protected area with the valuable help of its staff. WWF-Adena helped us with logistics and with its facilities at the Montejo de la Vega Bird of Prey Refuge. The Ringing Office of the Doñana Biological Station allowed us to consult their ringing databases.
Funding
Research was partially funded through projects PID2019-109685GB-I00, CGL2007-61395, CGL2010-15726, and CGL2013-42451-P, of the Spanish Ministry of Science and Innovation.
Conflict of Interest
The authors declare no conflict of interests.
Authors’ Contributions
F.M, M.C., and G.B. conceived the study; F.M. and G.B. undertook the fieldwork; F.M, M.C., and G.B. conducted the analyses; F.M, M.C., and G.B. wrote the manuscript.
Ethics Statement
Fieldwork and procedures were conducted under permits from the Spanish Bird Ringing Centre (permit numbers: 530109 to F.M, and 530115 to G.B.) and the regional government of Castilla y León (expedient, EP/CyL/282/2013), Dirección General del Medio Natural, Servicio de Espacios Naturales, in accordance with the approved guidelines proposed by the Spanish Royal Decree 1205/2005 on the protection of animals used in experiments and scientific research. This study complies with the ARRIVE guidelines.
Data Availability
The datasets used and/or analysed during the current study are available from the corresponding author on reasonable request.