-
PDF
- Split View
-
Views
-
Cite
Cite
Marco A Pizo, Vinicius R Tonetti, Living in a fragmented world: Birds in the Atlantic Forest, The Condor: Ornithological Applications, Volume 122, Issue 3, 4 August 2020, duaa023, https://doi.org/10.1093/condor/duaa023
- Share Icon Share
Abstract
The Atlantic Forest is the second largest tropical moist forest domain in South America after the Amazon, home to over 800 bird species (223 endemics or 27% of the avifauna). With only 28% of the original vegetation left, mostly fragmented and altered, the Atlantic Forest is a hotspot for bird conservation. We first introduce the extent, vegetation types, and exploitation history of the domain, and the composition and biogeographic affinities of its birds. We then provide an overview of the knowledge gathered so far on the ways Atlantic Forest birds thrive in the often-fragmented landscape, highlighting the landscape features that influence their occurrence and movement behavior. We end with the conservation issues affecting the Atlantic Forest birds and the actions hitherto taken to address them, including the establishment of conservation units, forest restoration, and rewilding.
Resumo
A Mata Atlântica (MA) é o segundo maior domínio formado principalmente por florestas tropicais úmidas da América do Sul depois da Amazônia, onde ocorrem mais de 800 espécies de aves (223 endêmicas ou 27% da avifauna). Com apenas 28% da vegetação original restante, em sua maior parte fragmentada e alterada, a MA é um hotspot para a conservação das aves. Após uma breve introdução ao bioma (extensão, tipos de vegetação e histórico de exploração) e suas aves (composição e afinidades biogeográficas), trazemos aqui uma visão geral do conhecimento acumulado até o momento sobre o modo como as aves se mantêm nas paisagens frequentemente fragmentadas da MA, destacando as características da paisagem que influenciam sua ocorrência e movimentação. Terminamos com as questões de conservação que afetam as aves da MA e ações até agora adotadas para resolvê-las, incluindo o estabelecimento de unidades de conservação, a restauração florestal e a reintrodução de espécies.
THE ATLANTIC FOREST AND ITS BIRDS
The Atlantic Forest is the second largest tropical moist forest domain in South America after the Amazon. Extending from approximately 3°S to 30°S, the Atlantic Forest stretches for >3,300 km, covering >1,450,000 km2, ~95% of which lies in Brazil and the remainder in Argentina and Paraguay (Silva et al. 2004, Joly et al. 2014; Figure 1). The precise delimitation of the Atlantic Forest is difficult because the transitions with other neighboring domains (the Brazilian Cerrado, Caatinga, and South American Pampas, all formed by savannas, shrublands, and grasslands) may be subtle and run for hundreds of kilometers. The Atlantic Forest extends into such drier domains along gallery forests or as enclaves (e.g., “brejos nordestinos” in 500–1,000 meters above sea level [m.a.s.l.] plateaus in the northern part of the Atlantic Forest; Figure 1) where edaphic and climatic conditions permit (Galindo-Leal and Câmara 2003).
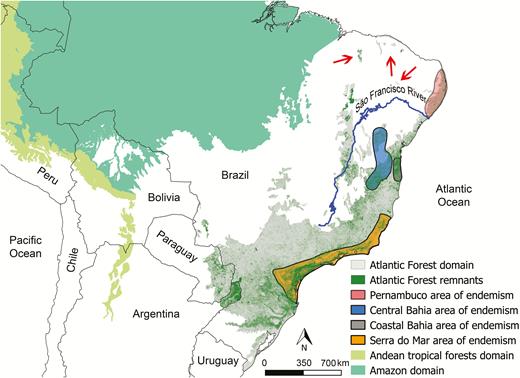
Map showing the original distribution of the Atlantic Forest domain in South America (in light gray) and the remaining vegetation in green. Remaining vegetation was compiled from different databases (M. C. Ribeiro personal communication). The original distributions of other domains with tropical forests in South America (Amazon and Andean forests; Olson et al. 2001) are also shown. Areas of bird endemism in the Atlantic Forest (Pernambuco, Central Bahia, Coastal Bahia, and Serra do Mar according to Silva et al. 2004) are represented by colored polygons. Brejos nordestinos (i.e. Atlantic Forest enclaves in the north extreme of the domain) are indicated by red arrows.
Two main forest types greatly defined by the rainfall regime are recognized: the Atlantic rain forest that mostly covers the eastern slopes of the mountain chain that runs along the Brazilian coast, but extends over 60 km from the coastline in some regions, and the semi-deciduous forest growing on the plateau towards the drier interior of the continent (Morellato and Haddad 2000). Elevation and associated temperature variations cause further differentiation within each of these 2 basic vegetation types leading to the formation of forest subtypes recognized by distinct structure and plant species composition (Oliveira-Filho and Fontes 2000). For instance, restinga forests growing on sand deposits in the coastal fringe have a physiognomically and floristically diverse vegetation generally characterized by halophytic herbs and shrubs to small trees growing on sand soils (Scarano 2002), while the dominance of the coniferous tree Araucaria angustifolia characterizes the mixed ombrophilous forests (or Araucaria forest) found in mountainous regions with subtropical climate and milder temperatures mostly in the southern parts of the Atlantic Forest.
The history of Atlantic Forest degradation dates back to the arrival of European colonizers in 1500. Stimulated by the exploitation of agricultural commodities (sugar cane, coffee) in the first centuries, the forest is now extirpated to give way to infrastructure development, urban expansion, and Eucalyptus plantations (Dean 1996, Joly et al. 2014). Regarding urban occupation, it is noteworthy that ~70% of the Brazilian population of over 200 million people lives in the Atlantic Forest domain, with over 3,000 municipalities, including some of the largest cities in the country (e.g., São Paulo, Belo Horizonte, Rio de Janeiro: sosma.org.br/artigo/mata-atlantica-invisivel-nas-cidades).
With such a long history of forest exploitation, not much is left of the Atlantic Forest, which has lost ~72% of the original forest cover (Rezende et al. 2018; Figure 1). More than 80% of the remaining forest patches are smaller than 50 ha, resulting in widespread edge effects since almost half of the remaining forest is <100 m from the nearest edge. Moreover, forest remnants are well apart from each other (the average distance between fragments is 1,440 m; Ribeiro et al. 2009), which may represent a barrier to the movement of forest birds through the landscapes (see below). Conservation units of full protection (i.e. reserves with restricted land use) comprise only 9% of the remaining natural vegetation on the Brazilian part of the Atlantic Forest, meaning that the vast majority of the remaining forests are in private lands (Rezende et al. 2018).
Notwithstanding this level of forest degradation, the Atlantic Forest is home to one of the richest avifauna in the world, being surpassed in South America only by the Amazonian forest (Marini and Garcia 2005, Mittermeier et al. 2005). Around 830 bird species have been found in the Atlantic Forest (Hasui et al. 2018), a figure that may be underestimated due to historical records with no secure confirmation and ongoing taxonomic revisions that may split taxa in the near future (Buzzetti and Barnett 2014). Even peri-urban areas can achieve high bird richness, as attested by the 326 bird species which were recorded in a forest remnant on the fringe of São Paulo, the largest city in the southern hemisphere (Tonetti et al. 2017).
Most of the species occurring at the Atlantic Forest are resident (~77%), but 68 species are migratory, whereas 132 are either partially migratory or make movements restricted to the domain (Somenzari et al. 2018). With altitudinal ranges spanning from sea level to over 2,000 m, it is not surprising that several bird species engage in seasonal, poorly known altitudinal movements relevant to the temporal dynamics and organization of communities. Hummingbirds, woodpeckers, toucans, and passerines are among the birds that make altitudinal movements within the Atlantic Forest (Willis and Schuchmann 1993, Bencke and Kindel 1999, Galetti et al. 2000), the details of which (e.g., periodicity, proportion of individuals migrating) are, however, not known (Somenzari et al. 2018). Research on these topics is badly needed.
In a literature review, Cavarzere and Silveira (2012) found 9 studies that sampled birds along elevation gradients in the Atlantic Forest, but found no clear altitudinal patterns of species richness distribution. In Serra dos Orgãos massif near Rio de Janeiro, for instance, the largest number of bird species occurs in the altitudinal range between 400 and 1,000 m, with species richness decreasing sharply above 1,000 m (Mallet-Rodrigues et al. 2010). Close to the coast, Goerck (1999) detected a linear decrease in bird species richness from low (sea level to 100 m) to high elevation (950–1,150 m) zones. Rather than revealing a true biological pattern, the comparatively lower number of species occurring at lowland forests may only reflect the poor conservation status of such forests, which have been severely impacted by human exploitation over the centuries and are now greatly reduced (Goerck 1999, Ribeiro et al. 2009).
Species inhabiting lowland forests are frequently replaced by congeneric species upward. Mallet-Rodrigues et al. (2010) suggested altitudinal replacements involving 17 genera of passerines and non-passerines. On the other hand, certain species may expand their altitudinal zones of occurrence along the latitudinal range of the Atlantic Forest. For instance, species of high altitudes in southeastern Brazil (e.g., Hooded Berryeater [Carpornis cucullate]) are found at lower altitudes and broader altitudinal range southwards. The absence of lowland congeneric species and abiotic factors (e.g., mild temperatures at southern lowland forests) are factors that may be involved in the occupation of lower altitudes towards the south by such species (Bencke and Kindel 1999).
The number of endemic birds is high in the Atlantic Forest (223 species; Vale et al. 2018), representing ~27% of the avifauna. The proportion of endemic species increases with altitude, from ~25% at 100 m elevation to 40% at 2,000 m (Mallet-Rodrigues et al. 2010). Furthermore, the closer to the coast the higher the number of endemic species (Jenkins et al. 2015). The number and location of the centers of bird endemism are historically controversial, but 4–5 of such centers have been identified, roughly corresponding to the region north of the São Francisco river (Pernambuco Center of Endemism), Bahia, Serra do Mar, the interior semi-deciduous forests, and Araucaria forests (Silva et al. 2004; Figure 1).
From a continental perspective, the Atlantic Forest has been compared to an island, isolated from other large expanses of South American forests (Amazonian and tropical Andean forests; Figure 1) by a corridor of more open and dry vegetation characteristic of Caatinga, Cerrado, and Chaco domains (Ab’Sáber 1977). This isolation per se supposedly contributed to the development of such a great level of endemism, but other mechanisms (e.g., neotectonic activity, riverine barriers) may have acted in consort to produce the uniqueness of the Atlantic Forest avifauna (Dantas et al. 2011). The development of distinct vegetation types has likely also been influenced by altitudinal variation and the huge latitudinal range of the forest. Climatic fluctuations causing the expansion and contraction of vegetation boundaries is another factor that over the millennia may have influenced the formation and distribution of bird species. The so-called refugia theory, originally proposed for Amazonian avifauna (Haffer 1969), has been applied to the Atlantic Forest biota to explain the phylogeography patterns of Atlantic Forest birds (Cabanne et al. 2008, D’Horta et al. 2011, Maldonado‐Coelho 2012).
Of great relevance for the biogeography and species richness of Atlantic Forest birds are the connections that in the past linked the Atlantic Forest to the now isolated Amazonian and Andean tropical forests (Willis 1992). Geological, palynological, phylogeographical, and biogeographical studies suggest that connections between the Amazon and the Atlantic Forest apparently prevailed for certain periods so that the avifauna of northern Atlantic Forest is more closely related to eastern Amazonian than southern Atlantic Forest (Santos et al. 2007, Sobral-Souza et al. 2015). Some species that occur in northern parts of the Atlantic Forest are also found in the Amazon but not in southern Atlantic Forest (e.g., Screaming Piha [Lipaugus vociferans], Cinereous Antshrike [Thamnomanes caesius]). On the other hand, in cold and isolated mountaintops of south-southeast Brazil we can find taxa of clear Andean affinities (e.g., Itatiaia Spinetail [Asthenes moreirae], Cinclodes spp.; Sick 1985). Some of these taxa (e.g., tapaculos, Rhinocryptidae) speciated prodigiously after isolation of their Atlantic Forest ancestors from their source domains (Mauricio 2005).
An issue that has intrigued researchers is the small number of bird extinctions in the whole Atlantic Forest domain despite so great a reduction in forest area. Until 2003, the Glaucous Macaw (Anodorhynchus glaucus) was the only bird occurring in the Atlantic Forest to figure in the Brazilian official list of extinct birds. In spite of that, the Alagoas Curassow (Pauxi mitu) was extinct in the wild, the Rio de Janeiro Antwren (Myrmotherula fluminensis) is only known by the specimen type, a few other species were not recorded for decades (e.g., Kinglet Calyptura [Calyptura cristata], Purple-winged Ground-dove [Claravis geoffroyi]), and others were known to survive in small populations (e.g., Alagoas Antwren [Myrmotherula snowi], Black-hooded Antwren [Formicivora erythronotus], Cherry-throated Tanager [Nemosia rourei]; Lees and Pimm 2015). Three non-mutually exclusive hypotheses have been proposed to explain the apparent resilience of Atlantic Forest birds. First, bird populations are resilient to chronic habitat alteration resulting from millennia of forest exploitation by a considerable human population (Brown and Brown 1992, Dean 1996, Protomastro 1999), although apparently indigenous human populations in the Atlantic Forest never impacted the forest in prehistoric times on a magnitude capable of threatening any bird species. Second, species may have become extinct before we knew them, as exemplified by a mysterious Crax curassow known only from a drawing appearing in the 1648 treatise Historia Naturalis Brasiliae by the German and Dutch naturalists Georg Marcgrave and Willem Piso (Piso and MarcGraff 1948). Third, there may be an extinction time-lag with respect to deforestation (i.e. we should not expect that deforestation and extinctions walk side-by-side; Brooks et al. 1999). Apparently, such extinction debt is currently being paid and has officially been attested to by the Brazilian Government in its most recent list of extinct species that includes 2 furnarids (the Cryptic Treehunter [Cichlocolaptes mazarbarnetti], only described in 2014, and the Alagoas Foliage-gleaner [Philydor novaesi]) and the Pernambuco Pygmy-owl (Glaucidium mooreorum, described in 2002), all of them restricted to the severely degraded Atlantic Forest of the Pernambuco Center of Endemism in northeast Brazil (Pereira et al. 2014, Lees and Pimm 2015; Figure 1).
A dataset compiling the recent (from the 1970s onwards) occurrence of 745 bird species at 576 sites in the Brazilian Atlantic Forest using quantitative sampling methods (mist nets, point counts, and line transects), revealed that the top 10 most frequent bird species occurring in ~60% of the samples, depending on the method, were mostly small, predominantly insectivorous passerines with low or medium sensitivity to forest perturbation (Hasui et al. 2018; Table 1). In relation to forest dependence, the most common bird species vary from dependent to independent of forests, with a clear predominance of dependent species among understory birds (Table 1).
The 10 most common bird species in the Atlantic Forest (according to their frequency of occurrence) sampled with 3 different methods (mist nets, point counts, line transects) and some of their morphological (body mass), and ecological features (sensitivity to habitat disturbance, forest dependence, and predominant diet). Species that are most common by all 3 methods are in bold and underlined, whereas those common in any of 2 methods are underlined only. Frequency data based on Hasui et al. (2018).
Order . | Family . | Species . | Frequency of occurrence a . | Body mass (g) b . | Sensitivity to habitat disturbance c . | Forest dependence d . | Predominant diet b . |
---|---|---|---|---|---|---|---|
Mist nets | |||||||
Passeriformes | Parulidae | Basileuterus culicivorus | 0.84 | 10.5 | Low | Dependent | Invertebrate |
Passeriformes | Tyrannidae | Platyrinchus mystaceus | 0.69 | 9.7 | Medium | Dependent | Invertebrate |
Passeriformes | Conopophagidae | Conopophaga lineata | 0.65 | 25.2 | Low | Dependent | Invertebrate |
Passeriformes | Thraupidae | Trichothraupis melanops | 0.65 | 22.6 | Medium | Dependent | Invertebrate |
Passeriformes | Pipridae | Chiroxiphia caudata | 0.63 | 25.6 | Low | Dependent | Frugivore |
Passeriformes | Thamnophilidae | Dysithamnus mentalis | 0.60 | 14.9 | Medium | Dependent | Invertebrate |
Passeriformes | Tyrannidae | Leptopogon amaurocephalus | 0.57 | 11.7 | Medium | Dependent | Invertebrate |
Passeriformes | Furnariidae | Sittasomus griseicapillus | 0.57 | 13.1 | Medium | Dependent | Invertebrate |
Passeriformes | Turdidae | Turdus albicollis | 0.56 | 54.0 | Medium | Dependent | Frugivore |
Passeriformes | Thamnophilidae | Pyriglena leucoptera | 0.54 | 28.8 | Medium | Dependent | Invertebrate |
Point counts | |||||||
Passeriformes | Vireonidae | Cyclarhis gujanensis | 0.84 | 28.8 | Low | Semi-dependent | Invertebrate |
Passeriformes | Parulidae | Basileuterus culicivorus | 0.83 | 10.5 | Low | Dependent | Invertebrate |
Passeriformes | Tyrannidae | Camptostoma obsoletum | 0.75 | 8.1 | Low | Independent | Invertebrate |
Passeriformes | Thamnophilidae | Thamnophilus caerulescens | 0.74 | 21.1 | Low | Dependent | Invertebrate |
Passeriformes | Tyrannidae | Lathrotriccus euleri | 0.71 | 11.3 | Low | Dependent | Invertebrate |
Columbiformes | Columbidae | Patagioenas picazuro | 0.71 | 279.0 | Medium | Semi-dependent | Granivore |
Cuculiformes | Cuculidae | Piaya cayana | 0.69 | 102.0 | Low | Semi-dependent | Invertebrate |
Passeriformes | Vireonidae | Vireo chivi | 0.68 | 16.1 | Low | Dependent | Invertebrate |
Passeriformes | Tyrannidae | Pitangus sulphuratus | 0.67 | 62.9 | Low | Independent | Omnivore |
Passeriformes | Conopophagidae | Conopophaga lineata | 0.66 | 25.2 | Low | Dependent | Invertebrate |
Line transects | |||||||
Passeriformes | Vireonidae | Cyclarhis gujanensis | 0.95 | 28.8 | Low | Semi-dependent | Invertebrate |
Passeriformes | Turdidae | Turdus leucomelas | 0.83 | 69.1 | Low | Semi-dependent | Invertebrate |
Columbiformes | Columbidae | Leptotila verreuaxi | 0.80 | 146.9 | Low | Semi-dependent | Granivore |
Passeriformes | Tyrannidae | Megarynchus pitangua | 0.72 | 69.9 | Low | Semi-dependent | Invertebrate |
Passeriformes | Tyrannidae | Camptostoma obsoletum | 0.70 | 8.1 | Low | Independent | Invertebrate |
Columbiformes | Columbidae | Patagioenas picazuro | 0.70 | 279.0 | Medium | Semi-dependent | Granivore |
Passeriformes | Parulidae | Basileuterus culicivorus | 0.69 | 10.5 | Low | Dependent | Invertebrate |
Cuculiformes | Cuculidae | Piaya cayana | 0.69 | 102.0 | Low | Semi-dependent | Invertebrate |
Passeriformes | Tyrannidae | Tyrannus melancholicus | 0.69 | 37.4 | Low | Independent | Invertebrate |
Passeriformes | Tyrannidae | Pitangus sulphuratus | 0.66 | 62.9 | Low | Independent | Omnivore |
Order . | Family . | Species . | Frequency of occurrence a . | Body mass (g) b . | Sensitivity to habitat disturbance c . | Forest dependence d . | Predominant diet b . |
---|---|---|---|---|---|---|---|
Mist nets | |||||||
Passeriformes | Parulidae | Basileuterus culicivorus | 0.84 | 10.5 | Low | Dependent | Invertebrate |
Passeriformes | Tyrannidae | Platyrinchus mystaceus | 0.69 | 9.7 | Medium | Dependent | Invertebrate |
Passeriformes | Conopophagidae | Conopophaga lineata | 0.65 | 25.2 | Low | Dependent | Invertebrate |
Passeriformes | Thraupidae | Trichothraupis melanops | 0.65 | 22.6 | Medium | Dependent | Invertebrate |
Passeriformes | Pipridae | Chiroxiphia caudata | 0.63 | 25.6 | Low | Dependent | Frugivore |
Passeriformes | Thamnophilidae | Dysithamnus mentalis | 0.60 | 14.9 | Medium | Dependent | Invertebrate |
Passeriformes | Tyrannidae | Leptopogon amaurocephalus | 0.57 | 11.7 | Medium | Dependent | Invertebrate |
Passeriformes | Furnariidae | Sittasomus griseicapillus | 0.57 | 13.1 | Medium | Dependent | Invertebrate |
Passeriformes | Turdidae | Turdus albicollis | 0.56 | 54.0 | Medium | Dependent | Frugivore |
Passeriformes | Thamnophilidae | Pyriglena leucoptera | 0.54 | 28.8 | Medium | Dependent | Invertebrate |
Point counts | |||||||
Passeriformes | Vireonidae | Cyclarhis gujanensis | 0.84 | 28.8 | Low | Semi-dependent | Invertebrate |
Passeriformes | Parulidae | Basileuterus culicivorus | 0.83 | 10.5 | Low | Dependent | Invertebrate |
Passeriformes | Tyrannidae | Camptostoma obsoletum | 0.75 | 8.1 | Low | Independent | Invertebrate |
Passeriformes | Thamnophilidae | Thamnophilus caerulescens | 0.74 | 21.1 | Low | Dependent | Invertebrate |
Passeriformes | Tyrannidae | Lathrotriccus euleri | 0.71 | 11.3 | Low | Dependent | Invertebrate |
Columbiformes | Columbidae | Patagioenas picazuro | 0.71 | 279.0 | Medium | Semi-dependent | Granivore |
Cuculiformes | Cuculidae | Piaya cayana | 0.69 | 102.0 | Low | Semi-dependent | Invertebrate |
Passeriformes | Vireonidae | Vireo chivi | 0.68 | 16.1 | Low | Dependent | Invertebrate |
Passeriformes | Tyrannidae | Pitangus sulphuratus | 0.67 | 62.9 | Low | Independent | Omnivore |
Passeriformes | Conopophagidae | Conopophaga lineata | 0.66 | 25.2 | Low | Dependent | Invertebrate |
Line transects | |||||||
Passeriformes | Vireonidae | Cyclarhis gujanensis | 0.95 | 28.8 | Low | Semi-dependent | Invertebrate |
Passeriformes | Turdidae | Turdus leucomelas | 0.83 | 69.1 | Low | Semi-dependent | Invertebrate |
Columbiformes | Columbidae | Leptotila verreuaxi | 0.80 | 146.9 | Low | Semi-dependent | Granivore |
Passeriformes | Tyrannidae | Megarynchus pitangua | 0.72 | 69.9 | Low | Semi-dependent | Invertebrate |
Passeriformes | Tyrannidae | Camptostoma obsoletum | 0.70 | 8.1 | Low | Independent | Invertebrate |
Columbiformes | Columbidae | Patagioenas picazuro | 0.70 | 279.0 | Medium | Semi-dependent | Granivore |
Passeriformes | Parulidae | Basileuterus culicivorus | 0.69 | 10.5 | Low | Dependent | Invertebrate |
Cuculiformes | Cuculidae | Piaya cayana | 0.69 | 102.0 | Low | Semi-dependent | Invertebrate |
Passeriformes | Tyrannidae | Tyrannus melancholicus | 0.69 | 37.4 | Low | Independent | Invertebrate |
Passeriformes | Tyrannidae | Pitangus sulphuratus | 0.66 | 62.9 | Low | Independent | Omnivore |
a Frequency of occurrence calculated as the ratio of the number of sites where each species occurred by the total number of sites sampled with mist nets (n = 181), point counts (n = 312), and line transects (n = 79).
b Body mass and predominant diet from Wilman et al. (2014), with modifications by the author in the last parameter.
c According to Stotz et al. (1996), with modifications by the author.
d According to Silva (1995), with modifications by the author. Dependent species are found mainly in forest habitats; semi-dependent species occur in forest but are also found frequently in open habitats, usually with scattered trees; whereas independent species occur mostly in open vegetation like pastures and urban areas.
The 10 most common bird species in the Atlantic Forest (according to their frequency of occurrence) sampled with 3 different methods (mist nets, point counts, line transects) and some of their morphological (body mass), and ecological features (sensitivity to habitat disturbance, forest dependence, and predominant diet). Species that are most common by all 3 methods are in bold and underlined, whereas those common in any of 2 methods are underlined only. Frequency data based on Hasui et al. (2018).
Order . | Family . | Species . | Frequency of occurrence a . | Body mass (g) b . | Sensitivity to habitat disturbance c . | Forest dependence d . | Predominant diet b . |
---|---|---|---|---|---|---|---|
Mist nets | |||||||
Passeriformes | Parulidae | Basileuterus culicivorus | 0.84 | 10.5 | Low | Dependent | Invertebrate |
Passeriformes | Tyrannidae | Platyrinchus mystaceus | 0.69 | 9.7 | Medium | Dependent | Invertebrate |
Passeriformes | Conopophagidae | Conopophaga lineata | 0.65 | 25.2 | Low | Dependent | Invertebrate |
Passeriformes | Thraupidae | Trichothraupis melanops | 0.65 | 22.6 | Medium | Dependent | Invertebrate |
Passeriformes | Pipridae | Chiroxiphia caudata | 0.63 | 25.6 | Low | Dependent | Frugivore |
Passeriformes | Thamnophilidae | Dysithamnus mentalis | 0.60 | 14.9 | Medium | Dependent | Invertebrate |
Passeriformes | Tyrannidae | Leptopogon amaurocephalus | 0.57 | 11.7 | Medium | Dependent | Invertebrate |
Passeriformes | Furnariidae | Sittasomus griseicapillus | 0.57 | 13.1 | Medium | Dependent | Invertebrate |
Passeriformes | Turdidae | Turdus albicollis | 0.56 | 54.0 | Medium | Dependent | Frugivore |
Passeriformes | Thamnophilidae | Pyriglena leucoptera | 0.54 | 28.8 | Medium | Dependent | Invertebrate |
Point counts | |||||||
Passeriformes | Vireonidae | Cyclarhis gujanensis | 0.84 | 28.8 | Low | Semi-dependent | Invertebrate |
Passeriformes | Parulidae | Basileuterus culicivorus | 0.83 | 10.5 | Low | Dependent | Invertebrate |
Passeriformes | Tyrannidae | Camptostoma obsoletum | 0.75 | 8.1 | Low | Independent | Invertebrate |
Passeriformes | Thamnophilidae | Thamnophilus caerulescens | 0.74 | 21.1 | Low | Dependent | Invertebrate |
Passeriformes | Tyrannidae | Lathrotriccus euleri | 0.71 | 11.3 | Low | Dependent | Invertebrate |
Columbiformes | Columbidae | Patagioenas picazuro | 0.71 | 279.0 | Medium | Semi-dependent | Granivore |
Cuculiformes | Cuculidae | Piaya cayana | 0.69 | 102.0 | Low | Semi-dependent | Invertebrate |
Passeriformes | Vireonidae | Vireo chivi | 0.68 | 16.1 | Low | Dependent | Invertebrate |
Passeriformes | Tyrannidae | Pitangus sulphuratus | 0.67 | 62.9 | Low | Independent | Omnivore |
Passeriformes | Conopophagidae | Conopophaga lineata | 0.66 | 25.2 | Low | Dependent | Invertebrate |
Line transects | |||||||
Passeriformes | Vireonidae | Cyclarhis gujanensis | 0.95 | 28.8 | Low | Semi-dependent | Invertebrate |
Passeriformes | Turdidae | Turdus leucomelas | 0.83 | 69.1 | Low | Semi-dependent | Invertebrate |
Columbiformes | Columbidae | Leptotila verreuaxi | 0.80 | 146.9 | Low | Semi-dependent | Granivore |
Passeriformes | Tyrannidae | Megarynchus pitangua | 0.72 | 69.9 | Low | Semi-dependent | Invertebrate |
Passeriformes | Tyrannidae | Camptostoma obsoletum | 0.70 | 8.1 | Low | Independent | Invertebrate |
Columbiformes | Columbidae | Patagioenas picazuro | 0.70 | 279.0 | Medium | Semi-dependent | Granivore |
Passeriformes | Parulidae | Basileuterus culicivorus | 0.69 | 10.5 | Low | Dependent | Invertebrate |
Cuculiformes | Cuculidae | Piaya cayana | 0.69 | 102.0 | Low | Semi-dependent | Invertebrate |
Passeriformes | Tyrannidae | Tyrannus melancholicus | 0.69 | 37.4 | Low | Independent | Invertebrate |
Passeriformes | Tyrannidae | Pitangus sulphuratus | 0.66 | 62.9 | Low | Independent | Omnivore |
Order . | Family . | Species . | Frequency of occurrence a . | Body mass (g) b . | Sensitivity to habitat disturbance c . | Forest dependence d . | Predominant diet b . |
---|---|---|---|---|---|---|---|
Mist nets | |||||||
Passeriformes | Parulidae | Basileuterus culicivorus | 0.84 | 10.5 | Low | Dependent | Invertebrate |
Passeriformes | Tyrannidae | Platyrinchus mystaceus | 0.69 | 9.7 | Medium | Dependent | Invertebrate |
Passeriformes | Conopophagidae | Conopophaga lineata | 0.65 | 25.2 | Low | Dependent | Invertebrate |
Passeriformes | Thraupidae | Trichothraupis melanops | 0.65 | 22.6 | Medium | Dependent | Invertebrate |
Passeriformes | Pipridae | Chiroxiphia caudata | 0.63 | 25.6 | Low | Dependent | Frugivore |
Passeriformes | Thamnophilidae | Dysithamnus mentalis | 0.60 | 14.9 | Medium | Dependent | Invertebrate |
Passeriformes | Tyrannidae | Leptopogon amaurocephalus | 0.57 | 11.7 | Medium | Dependent | Invertebrate |
Passeriformes | Furnariidae | Sittasomus griseicapillus | 0.57 | 13.1 | Medium | Dependent | Invertebrate |
Passeriformes | Turdidae | Turdus albicollis | 0.56 | 54.0 | Medium | Dependent | Frugivore |
Passeriformes | Thamnophilidae | Pyriglena leucoptera | 0.54 | 28.8 | Medium | Dependent | Invertebrate |
Point counts | |||||||
Passeriformes | Vireonidae | Cyclarhis gujanensis | 0.84 | 28.8 | Low | Semi-dependent | Invertebrate |
Passeriformes | Parulidae | Basileuterus culicivorus | 0.83 | 10.5 | Low | Dependent | Invertebrate |
Passeriformes | Tyrannidae | Camptostoma obsoletum | 0.75 | 8.1 | Low | Independent | Invertebrate |
Passeriformes | Thamnophilidae | Thamnophilus caerulescens | 0.74 | 21.1 | Low | Dependent | Invertebrate |
Passeriformes | Tyrannidae | Lathrotriccus euleri | 0.71 | 11.3 | Low | Dependent | Invertebrate |
Columbiformes | Columbidae | Patagioenas picazuro | 0.71 | 279.0 | Medium | Semi-dependent | Granivore |
Cuculiformes | Cuculidae | Piaya cayana | 0.69 | 102.0 | Low | Semi-dependent | Invertebrate |
Passeriformes | Vireonidae | Vireo chivi | 0.68 | 16.1 | Low | Dependent | Invertebrate |
Passeriformes | Tyrannidae | Pitangus sulphuratus | 0.67 | 62.9 | Low | Independent | Omnivore |
Passeriformes | Conopophagidae | Conopophaga lineata | 0.66 | 25.2 | Low | Dependent | Invertebrate |
Line transects | |||||||
Passeriformes | Vireonidae | Cyclarhis gujanensis | 0.95 | 28.8 | Low | Semi-dependent | Invertebrate |
Passeriformes | Turdidae | Turdus leucomelas | 0.83 | 69.1 | Low | Semi-dependent | Invertebrate |
Columbiformes | Columbidae | Leptotila verreuaxi | 0.80 | 146.9 | Low | Semi-dependent | Granivore |
Passeriformes | Tyrannidae | Megarynchus pitangua | 0.72 | 69.9 | Low | Semi-dependent | Invertebrate |
Passeriformes | Tyrannidae | Camptostoma obsoletum | 0.70 | 8.1 | Low | Independent | Invertebrate |
Columbiformes | Columbidae | Patagioenas picazuro | 0.70 | 279.0 | Medium | Semi-dependent | Granivore |
Passeriformes | Parulidae | Basileuterus culicivorus | 0.69 | 10.5 | Low | Dependent | Invertebrate |
Cuculiformes | Cuculidae | Piaya cayana | 0.69 | 102.0 | Low | Semi-dependent | Invertebrate |
Passeriformes | Tyrannidae | Tyrannus melancholicus | 0.69 | 37.4 | Low | Independent | Invertebrate |
Passeriformes | Tyrannidae | Pitangus sulphuratus | 0.66 | 62.9 | Low | Independent | Omnivore |
a Frequency of occurrence calculated as the ratio of the number of sites where each species occurred by the total number of sites sampled with mist nets (n = 181), point counts (n = 312), and line transects (n = 79).
b Body mass and predominant diet from Wilman et al. (2014), with modifications by the author in the last parameter.
c According to Stotz et al. (1996), with modifications by the author.
d According to Silva (1995), with modifications by the author. Dependent species are found mainly in forest habitats; semi-dependent species occur in forest but are also found frequently in open habitats, usually with scattered trees; whereas independent species occur mostly in open vegetation like pastures and urban areas.
The present-day Atlantic Forest avifauna still preserves astonishing foraging specialization. Although professional ant-followers do not occur as in the Amazon, several species regularly follow army ants in the Atlantic Forest (Pizo and Melo 2010). Additionally, the avifauna contains species that concentrate foraging in bamboo stands (e.g., Dusky-cheeked Foliage-gleaner [Anabazenops fuscus]; Rodrigues et al. 1994), epiphytic bromeliads (e.g., Pale-browed Treehunter [Cichlocolaptes leucophrus]; Cestari 2009), and suspended dead leafs (e.g., Ochre-rumped Antbird [Drymophila ochropyga]; Leme 2001). The highly specialized Araucaria Tit-spinetail (Leptasthenura setaria) forages and nests only in the canopy of Araucaria angustifolia, the coniferous tree native to the Atlantic Forest (Cabanne et al. 2007).
In relation to foraging associations, several bird species participate in mixed-species flocks, but most of them are not regular or common (i.e. present in <25% of the flocks; Powell 1985) at such flocks. At Intervales State Park, which forms with adjacent reserves one of the largest blocks (>120,000 ha) of Atlantic Rain Forest in southeast Brazil, 64, 110, and 121 birds species were recorded in mixed-species flocks at sites located at 70, 600, and 800 m.a.s.l., respectively (M. A. Pizo, A. Aleixo, and C. G. Machado personal communication). The average number of species in these flocks varied from 5.0 to 6.8 (range: 2–28, n = 869 flocks). The distinction between canopy and understory flocks is not straightforward in the Atlantic Forest as in the Amazon, likely because the lower stature and frequently degraded state of most Atlantic Forest vegetation intermingles these 2 flock systems. As a consequence, the most commonly observed flock type is dubbed heterogeneous flocks, not clearly associated with a given vegetation stratum. Such flocks are known to occur in forest fragments and disturbed forests, but it is not clear whether they are widespread (Davis 1946), or mainly associated with disturbed habitats (Maldonado-Coelho and Marini 2004). Where the integrity of the forest vertical structure is preserved, typical canopy and understory flocks are found. Canopy flocks prominently have tanagers (e.g., Tangara spp., Rufous-headed Tanager [Hemithraupis ruficapilla]) and tyrant flycatchers (Sibilant Sirystes [Sirystes sybilator], Three-striped Flycatcher [Conopias trivirgatus]), while understory flocks often have Red-crowned Ant-Tanager (Habia rubica) as the nuclear species, followed by foliage-gleaners (Phyllidor spp.) and woodcreepers (e.g., Lesser Woodcreeper [Xiphorhynchus fuscus], Plain-winged Woodcreeper [Dendrocincla turdina]). With a great latitudinal span, the composition of Atlantic Forest mixed-species flocks may change substantially with latitude. For instance, the Cinereous Antshrike often replaces H. rubica as the nuclear species in understory flocks occurring north of Rio de Janeiro (Stotz 1993).
LIVING IN FRAGMENTED LANDSCAPES
With a rich avifauna, long history of forest conversion, widespread fragmentation, and an active community of ornithologists and ecologists interested in the effects of forest fragmentation on birds, the Atlantic Forest is a source of information on the responses of tropical birds to the shrinking and alteration of habitats. Although the most sensitive species have already disappeared from most Atlantic Forest fragments, the present avifauna obviously encompasses ranges of sensitivity to perturbation and responses to landscape features. Which factors affect the local occurrence and movement of birds through the landscape, and how such factors vary among species, are frequent questions posed about Atlantic Forest birds. The answers that arose point to a myriad of factors, including patch (e.g., size, shape, isolation) and landscape features (e.g., the composition of the matrix, connectivity, vegetation cover), the relative importance of which depends on the species and their characteristics (Uezu et al. 2005, Hansbauer et al. 2008, Martensen et al. 2008, 2012, Boscolo and Metzger 2009, 2011, Banks-Leite et al. 2010, 2013, Uezu and Metzger 2011, Coelho et al. 2016, Barbosa et al. 2017).
Of historical relevance in the construction of this knowledge was Willis’ (1979) pioneering study on the effects of forest patch size on birds. Through surveys of 3 forest fragments of 21, 250, and 1,400 ha, Willis documented the expected reduction in species richness with fragment size and that local extinction of bird species was not random with respect to foraging guilds. Large canopy frugivores (e.g., cotingids, toucans) and understory insectivores (e.g., antbirds, woodcreepers) were particularly affected, while species typical of forest edges were favored (Bovo et al. 2018; see also Giraudo et al. 2008 for Argentina). When Aleixo and Vielliard (1995) returned to the medium-sized fragment 15 yr later, an additional 30 species characteristic of forest interior, notably understory insectivores, had disappeared. Other studies using the before-and-after design to reveal patterns of bird extinction found similar non-random extinction among functional groups (e.g., Willrich et al. 2016), which in general mirrors what has been found in Amazonian fragmented landscapes (Bierregaard and Lovejoy 1989). Large predators, such as the Harpy Eagle (Harpia harpyja) and the Crested Eagle (Morphnus guianensis), have few recent records in the Atlantic Forest, which suggests that these birds are on the verge of extinction in the domain (Hasui et al. 2018). Interestingly and particularly relevant for conservation purposes, Atlantic Forest endemics are particularly affected by forest fragmentation (Ribon et al. 2003, Antunes 2007).
Apparently counteracting the effects of fragmentation, Atlantic Forest fragments have been colonized by bird species over the years, mostly species not totally dependent on forested habitats (Faria et al. 2006, Antunes 2007). Even though this process may offset the loss of local species richness and phylogenetic diversity of forest-dependent birds, ecological functions promoted by forest birds (e.g., seed dispersal, predation on leaf herbivores) may be compromised (Morante-Filho et al. 2018).
Size and shape influence the amount of edge in a given forest patch, because the smaller and more irregular a patch is then the greater the relative coverage of edge area it has. Among Atlantic Forest birds, as in any other forested domains, we find edge-loving (i.e. species preferring forest borders such as the Rufous-bellied Thrush [Turdus rufiventris], Pale-breasted Thrush [T. leucomelas]; Silveira et al. 2016), edge-tolerant (e.g., White-shouldered Fire-eye [Pyriglena leucoptera]; Hansbauser et al. 2008), and edge-avoiding birds (e.g., Swallow-tailed Manakin [Chiroxiphia caudata], Rufous-breasted Leaftosser [Sclerurus scansor]; Hansbauser et al. 2008). Similar to Amazonian birds, specialist insectivores, such as the Star-throated Antwren (Rhopias gularis), are negatively affected by edges, while nectarivores are apparently benefited, resulting in a shift in population abundances, community composition, and functional profile at forest edges (Banks-Leite et al. 2010).
Apart from patch area, patch isolation emerged as one of the most important factors affecting the occurrence of birds in forest fragments, stimulating studies on the capacity of forest birds to move across non-forested habitats, the factors that influence such capacity, and what management actions are needed to enhance the functional connectivity of fragmented landscapes (Boscolo and Metzger 2011). Using the playback technique (Bélisle 2005), Awade and Metzger (2008) studied 2 insectivorous birds typical of the forest under/midstory (Golden-crowned Warbler [Basileuterus culicivorus] and Variable Antshrike [Thamnophilus caerulescens]) and found a reduced probability of crossing open matrixes (formed by pastures, agricultural fields, and power line areas) with increasing gap width. The probability of crossing 40 m gaps was 50% for both species, decreasing to 10% when the gaps were 60 m (for B. culicivorus) or 80 m (for T. caerulescens). Shorter crossing distances were obtained for another understory species, P. leucoptera, with a 50% chance of crossing a 25 m gap and zero chance of crossing a gap of >55 m (Awade et al. 2012). Such distances, however, may not properly represent the complete ability of species to travel through fragmented landscapes, since they were obtained under specific situations of agonistic behavior. In a more natural setting, Marini (2010) noted that birds recaptured with mist nets crossed up to 650 m of open areas between forest fragments. Data available so far thus indicate that even forest birds not particularly sensitive to forest disturbance (low to medium sensitivity according to Stotz et al. 1996) do not cross open matrixes regularly, and species differ in their abilities to use open matrixes surrounding their home forest fragments.
Intraspecific variation in crossing open matrixes also arises from the origin of birds. Individuals of P. leucoptera from fragmented landscapes are more resistant to cross matrixes than birds from continuous forest, whose low resistance to cross boundaries likely results from their fast exploratory behavior. With slow exploratory behavior, individuals from fragmented landscapes are, however, more successful in crossing open spaces, likely due to their better perception of predation risk, which is higher in the matrix (Cornelius et al. 2017). This difference suggests that P. leucoptera is capable of adaptive behavioral adjustments to fragmentation important to its persistence in fragmented landscapes. Whether such adjustments have a genetic basis is open to investigation. The effects of sex-biased dispersal, a common phenomenon in birds and an additional source of intraspecific variation in the ability to move through fragmented landscapes, also merits further research. In the case of P. leucoptera, females exhibit a higher propensity to leave forest fragments than males, and are subjected to a lower risk of predation when moving through the matrix (Awade et al. 2017, Biz et al. 2017). Female-biased dispersal may lead to male-biased sex ratios in fragments with potentially negative consequences for the dynamics of populations in fragmented landscapes (Dale 2001), although research in this area is lacking.
As a generalization, we can say that the movement of birds through the matrix is influenced by its “quality” for birds, with consequences for the connectivity of the landscape or, in the case of Atlantic Forest birds, the capacity of the landscape to facilitate their movements among forest fragments. The quality of the matrix includes its composition, the structure of the vegetation, and the risk of predation it poses to birds. Eucalyptus plantations, for instance, seem more permeable to forest birds than open matrixes like pastures. For understory birds, this is especially true if understory vegetation is left to grow within plantations, thus providing food resources and better structural and microclimate conditions (Barbosa et al. 2017, Biz et al. 2017). Similarly, coffee plantations are friendlier for forest-dependent birds than pastures or sugar cane plantations, land uses that presently dominate great parts of the Atlantic Forest (Coelho et al. 2016, Boesing et al. 2017). In sum, these studies confirm the common sense that for forest-dependent birds, usually of great conservation concern, the greater the similarity of the matrix to a forest the better. Obviously, it does not suffice to have a commercial plantation of Eucalyptus with no or poorly structured understory, which is better called “green desert” for native organisms in general (Giubbina et al. 2018, Barros et al. 2019; see below).
Landscape elements such as small forest or agroforestry patches, isolated trees, and live fences contribute to the functional connectivity of isolated forest fragments for birds, which use such elements as stepping stones or corridors to move through the landscape (Gabriel and Pizo 2005, Uezu et al. 2008, Barbosa et al. 2017). In doing so, birds may not take the shortest but a longer route between neighboring fragments if it permits the use of such landscape elements (Boscolo et al. 2008). Stepping stones, however, are not the universal solution for enhancing the functional connectivity of forest fragments as their effectiveness in doing so decreases with increasing isolation. For instance, fruiting trees isolated in pastures that were >70 m from the nearest forest fragment were used by 60% fewer species and received 80% fewer bird visits than trees within 20 m of fragments (Pizo and Santos 2011).
The movements of frugivores or other functional groups (e.g., hummingbirds as nectarivores, canopy insectivores) are much less known than understory insectivores, which is explained by the ease with which understory insectivores are captured with mist nets and (at least some of them) radio-tracked compared with other guilds. This functional bias is hindering our capacity to propose management actions designed to benefit a greater portion of the bird communities thriving in fragmented landscapes. For instance, live fences, which can improve landscape connectivity, are used more frequently by frugivorous than insectivorous birds (Gabriel and Pizo 2005; Figure 2). This is not surprising given that frugivores usually wander more widely than insectivores (Levey and Stiles 1992)
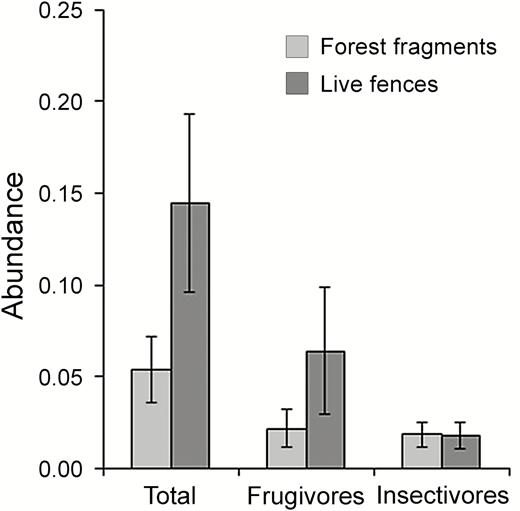
The abundance (captures per 10 m2 hr–1; see Straube and Bianconi 2002 for the sampling effort unit used here) of frugivores, insectivores (both categories according to Wilman et al. 2014), and the whole community of birds (Total) sampled with 36 mm mist nests at 5 forest fragments (<1–20 ha) and 3 live fences (4–12 m width, 150–400 m long, formed by regenerating native vegetation) connecting them at a fragmented landscape of the semi-deciduous Atlantic forest. Birds were sampled in 13 consecutive mo for a total sampling effort of 34.830 m2 hr–1 in fragments, and 14.170 m2 hr–1 in live fences. Vertical lines represent standard errors. (Source: V. A. Gabriel and M. A. Pizo personal communication).
Of overwhelming importance for the persistence of birds in the fragmented landscapes of the Atlantic Forest, even mediating the relative importance of the other patch and landscape features previously mentioned, is the amount of forest cover (Martensen et al. 2012, Boesing et al. 2017). Forest cover (30–50%) at the landscape level seems to represent a threshold below which the persistence of Atlantic Forest bird populations is compromised in the long term. This threshold, however, varies depending on the sensitivity of the species to forest perturbation, with the most sensitive species exhibiting a stronger positive response to increasing forest cover (Lopes et al. 2016, Martensen et al. 2012, Morante-Filho et al. 2015). As a result, the phylogenetic richness of forest-dependent birds increases with forest cover at the landscape scale, while the phylogenetic richness of birds not dependent on forests follows the opposite trend (Morante-Filho et al. 2018).
What has emerged so far is that nearby forest configuration, through its influence on isolation, often has a stronger effect on bird communities than surrounding forest cover or even forest patch size (Anjos et al. 2004, Develey and Metzger 2006). The relative effect of each of these factors is influenced by intrinsic characteristics of the species, including diet and foraging strategy (Uezu et al. 2005, Boscolo and Metzger 2011, Uezu and Metzger 2011). As a consequence, the spatial scale upon which landscape features influence the occurrence of species or functional group is variable (Boscolo and Metzger 2009, Banks-Leite et al. 2013). Martensen et al. (2008), for instance, noted that while terrestrial insectivores, omnivores, and frugivores were affected by both patch area and connectivity, only connectivity affected understory insectivores and nectarivores. While the occurrence of 2 understory insectivores (P. leucoptera and X. fuscus) was influenced by the landscape structure at 1,000 and 800 m radii around fragments, the occurrence of an understory frugivore (C. caudata) was best described by a 600 m scale (Boscolo and Metzger 2009).
CONSERVATION
With 66 threatened and 54 near-threatened species (Hasui et al. 2018), the Atlantic Forest is among the top 10 domains in the world in number of threatened bird species, deserving the status of a hotspot for bird conservation (Myers et al. 2000). In addition, 163 important bird areas (IBAs)—areas with a great number of threatened, endemic, or species with restricted distribution—have been identified in the Brazilian Atlantic Forest (Bencke et al. 2006). Twenty-five and 22 additional IBAs occur in the Argentinian and Paraguayan Atlantic Forest, respectively (Di Giacomo et al. 2007, Guyra Paraguay 2008). The main threat to birds does not differ from the worldwide pattern: habitat alteration (a term encompassing a diverse range of anthropogenic impacts upon habitats, including selective logging, habitat loss, and fragmentation) stands as the leading cause of bird declines (Marini and Garcia 2005). To this we can add the pet trade for specific groups (e.g., thraupids and parrots; Regueira and Bernard 2012) and hunting (e.g., cracids; Gama et al. 2016), the extent and relevance of which remains largely unevaluated for bird populations in the Atlantic Forest.
The establishment of reserves was the traditional way to protect the Atlantic Forest fauna. According to the Brazilian Ministry of the Environment (Ministério do Meio Ambiente 2017), the Atlantic Forest has 194 governmental reserves of restricted use plus 567 private reserves (Reservas Particulares do Patrimônio Natural). The importance of such a system of preserved areas for the most forest-dependent and sensitive bird species is unquestionable (Oliveira et al. 2017). However, it is clear that formal reserves will not guarantee the preservation of many Atlantic Forest birds into the future given the diversity of Atlantic Forest birds in terms of species and habitats used, the ups and downs of the economy, and the characteristic low priority of environmental issues in governmental agendas. To this end, the activity of non-governmental organizations (e.g., SAVE Brasil: savebrasil.org.br) and web-based initiatives (e.g., Wiki Aves: wikiaves.com.br; e-Bird Brasil: ebird.org/content/brasil) to enhance public education and increase knowledge of species distribution and awareness about birds is important. These initiatives are in tandem with the growing interest of laypersons in birds, resulting in a recent increase in the number of birdwatchers visiting Atlantic Forest sites, in the launching of bird fairs (e.g., AVISTAR: avistarbrasil.com.br), and projects based on the citizen science concept (e.g., the Neotropical Census of Aquatic Birds: wetlands.org).
These urban-based initiatives are welcome, since urban areas are growing and the Atlantic Forest is home to most of the Brazilian human population. Urban environments cannot be neglected as important habitats for birds, some of them of conservation concern (e.g., Southern Bristle-tyrant [Phylloscartes eximius]; Tonetti and Pizo 2016, Tonetti et al. 2017). It is remarkable, for instance, that new bird species were recently described in the vicinity of some of the biggest Atlantic Forest cities (such as the São Paulo Marsh Antwren [Formicivora paludicola] near São Paulo city, and the Cipó Cinclodes [Cinclodes espinhacensis] near Belo Horizonte; Freitas et al. 2012, Buzzetti et al. 2013). Besides contributing to educational activities (e.g., birdwatching), urban forest reserves play a crucial role in protecting a great proportion of the regional species richness (Enedino et al. 2018), and even the vegetation of the most urbanized areas may be managed for as friendly habitat for birds (Fontana et al. 2011, Pena et al. 2017).
Bird-friendly management should also be applied to plantations. In recent decades, the Atlantic Forest saw an increase in the area of tree plantations, as is happening worldwide (Forest Stewardship Council 2012). With regional differences, Eucalyptus and Pinus predominate as the tree species planted in the Atlantic Forest but, with a short harvesting cycle, such plantations have small conservation value for birds and other organisms, being used mostly by birds not dependent on forests (Marsden et al. 2001, Zurita et al. 2006). Eucalyptus and Pinus plantations were worse for birds than plantations of the native Araucaria angustifolia (Zurita et al. 2006, Volpato et al. 2010). However, low intensity management of exotic tree plantations involving, among other practices, a longer harvesting cycle and less frequent understory thinning, may substantially enhance the conservation value of plantations for birds (Fonseca et al. 2009, Lopes et al. 2015). Such practices permit a greater development of forest structure, important for the most sensitive forest birds (Zurita and Bellocq 2012).
Of great relevance for a domain so altered and reduced as the Atlantic Forest is forest restoration. Restoration in the Atlantic Forest has a long history dating back to the 19th century, but it was only in recent decades that accumulated knowledge on restoration techniques permitted the development of high-diversity self-perpetuating forests (Rodrigues et al. 2009). The area in need of restoration is immense. The Pact for Atlantic Forest Restoration, one of the most ambitious ecological restoration programs in the world, aims to restore 15 million hectares of forest by 2050 (Calmon et al. 2011). In consonance with this important enterprise, several restoration projects were launched in the last few years, representing valuable opportunities to investigate the response of birds to forest restoration and to evaluate the role of restored areas to the conservation of threatened bird species. For instance, the drivers of species composition in bird communities at restored sites are poorly known. A comparison between actively (i.e. sites planted with a high diversity of native trees) and passively (i.e. sites regenerating naturally) restored forest fragments revealed that the species composition of the latter is more homogeneous than the former (Figure 3). Differences in the tree species planted, management actions, and the resulting structure of the vegetation at actively restored sites are candidate factors to explain their more heterogeneous species composition. Regarding species richness, studies so far indicate that it takes ~10 yr for restored sites to reach about half the number of forest-dependent birds typical of reference sites (Becker et al. 2013), a result similar to Australian restored forests (Caterall et al. 2012). Not surprisingly, frugivores, carnivores, and understory insectivores are the functional groups that take longer to fully recover (Santos et al. 2016). The efficiency of restoration for the most sensitive bird species is likely dependent on landscape context (e.g., the amount of forest cover and patch isolation; Dias et al. 2015). In landscapes where human activity is intense, enlarging or improving (with enrichment planting, liana cutting, or any other intervention that improves vegetation structure) the best and largest fragments, and increasing connectivity among fragments (with stepping stones and corridors), should be important strategies for forest-dependent birds (Boscolo and Metzger 2011, Uezu and Metzger 2016). At any rate, a study based on ecological thresholds of habitat amount for amphibians, mammals, and birds has showed that it is feasible to restore extensive forest areas in the Atlantic Forest taking into account the economic and social realities in a way that future extinctions of endemic species can be avoided (Banks-Leite et al. 2014).
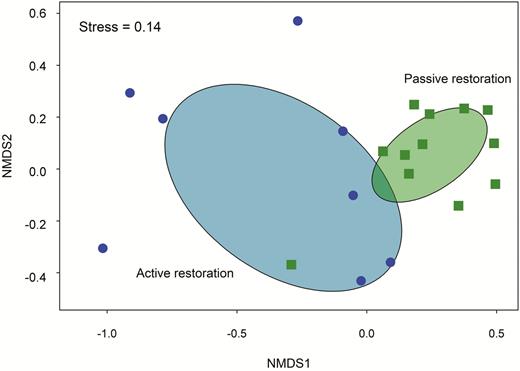
Non-metric multidimensional scaling (NMDS) on the similarity (given by the Bray–Curtis index) of bird communities sampled in actively (i.e. sites planted with a high diversity of native trees; 5–60 yr old; 8.3–305.0 ha; blue circles) and passively restored areas (i.e. sites regenerating naturally mostly from 1960 onwards; 1.3–170.0 ha; green squares) in semi-deciduous Atlantic forest in southeast Brazil. Birds were censused with 10 min point counts (1–10 points per area depending on the size of the area) during the 2015 breeding season (September–December). Ellipses represent the 95% confidence space for the 2 area categories. (Source: C. O. A. Gussoni and M. A. Pizo personal communication).
Forest restoration often must be accompanied by rewilding or the reintroduction of locally extinct animal species (Fernandez et al. 2017). With regard to Atlantic Forest birds, reintroduction attempts have been performed mostly with cracids, including the dramatic example of the Alagoas Curassow (Mitu mitu), a species that in the 1970s was on the brink of extinction. Starting from only 3 reproducing individuals in captivity, constituting one of the most severe bottlenecks ever documented for a wild animal that has survived, a breeding program substantially augmented the population. One drawback was the use of the Razor-billed Curassow (M. tuberosa) to deliberately produce hybrids (Silveira et al. 2004). With a growing captive population, Costa et al. (2017) embarked on a challenging technical enterprise to recover the genetic identity of the species, aiming at reintroduction in the wild. This process was not free from additional challenges, including the ongoing tradition of hunting in the original areas of occurrence (Gama et al. 2016). Other cracids reintroduced in the Atlantic Forest were the endangered Red-billed Curassow (Crax blumenbachii) (São Bernardo 2012), Black-fronted Piping-guan (Aburria jacutinga) (http://savebrasil.org.br/projeto-jacutinga), and guans Penelope spp. (Pereira and Wajntal 1999). Besides contributing to recovery of the bird species, the reintroduction of such large frugivorous birds also contributes to the dispersal of large seeds, normally devoid of seed dispersers in small forest fragments of the Atlantic Forest (Silva and Tabarelli 2000).
While dealing with old conservation challenges, such as hunting and deforestation, researchers are only beginning to address the possible influence of the new issue of climate change on Atlantic Forest birds. Using ecological niche modeling to assess the effect of climate change on the distribution of 51 endemic Atlantic Forest birds, an average loss of 45% of distribution areas was estimated for 44 species by 2050 (Souza et al. (2011). For 2060, a loss of 47% of the potential distribution area of the endangered Red-spectacled Amazon (Amazona pretrei) and a displacement of its distribution in the eastern direction of its current area of occurrence was estimated (Marini et al. 2010). For P. eximius, an uncommon insectivore bird endemic to the Atlantic Forest, an estimated reduction of 40% in its distribution area and altitudinal shift of 105 m upward by 2070 may occur (Tonetti 2015). With the exception of the latter, these studies did not take into account the role of forest fragmentation, which can synergistically augment the effects of climate change (Loiselle et al. 2010).
In conclusion, the task of maintaining the huge diversity of Atlantic Forest avifauna over the long term is immense. Given the small population sizes of several species, defeats will inevitably come, but successes are equally likely if we consider the myriad of people interested in the Atlantic Forest birds, either for academic, ethical, or recreational purposes. In the meantime, we continue to learn about the way birds cope within an anthropogenically altered, fragmented domain. This knowledge will inform strategies that will permit Atlantic Forest birds, and hopefully birds thriving in other tropical forests, to persist into the future.
ACKNOWLEDGMENTS
We thank Luis Fábio Silveira for comments that greatly improved the manuscript.
Funding statement: We thank the São Paulo Research Foundation (FAPESP) for funding studies whose unpublished data were presented here (grants 2002/1200–5 and 2015/13383-0). MAP receives a research grant from the Brazilian National Council for Scientific and Technological Development (CNPq), and VRT receives a fellowship from FAPESP (2018/20691-1).
Author contributions: MAP and VRT contributed equally to the writing of the paper and preparation of the figures.