-
PDF
- Split View
-
Views
-
Cite
Cite
Carolyn T Bramante, Kenneth B Beckman, Tanvi Mehta, Amy B Karger, David J Odde, Christopher J Tignanelli, John B Buse, Darrell M Johnson, Ray H B Watson, Jerry J Daniel, David M Liebovitz, Jacinda M Nicklas, Ken Cohen, Michael A Puskarich, Hrishikesh K Belani, Lianne K Siegel, Nichole R Klatt, Blake Anderson, Katrina M Hartman, Via Rao, Aubrey A Hagen, Barkha Patel, Sarah L Fenno, Nandini Avula, Neha V Reddy, Spencer M Erickson, Regina D Fricton, Samuel Lee, Gwendolyn Griffiths, Matthew F Pullen, Jennifer L Thompson, Nancy E Sherwood, Thomas A Murray, Michael R Rose, David R Boulware, Jared D Huling, COVID-OUT Study Team , Favorable Antiviral Effect of Metformin on SARS-CoV-2 Viral Load in a Randomized, Placebo-Controlled Clinical Trial of COVID-19, Clinical Infectious Diseases, Volume 79, Issue 2, 15 August 2024, Pages 354–363, https://doi.org/10.1093/cid/ciae159
- Share Icon Share
Abstract
Metformin has antiviral activity against RNA viruses including severe acute respiratory syndrome coronavirus 2 (SARS-CoV-2). The mechanism appears to be suppression of protein translation via targeting the host mechanistic target of rapamycin pathway. In the COVID-OUT randomized trial for outpatient coronavirus disease 2019 (COVID-19), metformin reduced the odds of hospitalizations/death through 28 days by 58%, of emergency department visits/hospitalizations/death through 14 days by 42%, and of long COVID through 10 months by 42%.
COVID-OUT was a 2 × 3 randomized, placebo-controlled, double-blind trial that assessed metformin, fluvoxamine, and ivermectin; 999 participants self-collected anterior nasal swabs on day 1 (n = 945), day 5 (n = 871), and day 10 (n = 775). Viral load was quantified using reverse-transcription quantitative polymerase chain reaction.
The mean SARS-CoV-2 viral load was reduced 3.6-fold with metformin relative to placebo (−0.56 log10 copies/mL; 95% confidence interval [CI], −1.05 to −.06; P = .027). Those who received metformin were less likely to have a detectable viral load than placebo at day 5 or day 10 (odds ratio [OR], 0.72; 95% CI, .55 to .94). Viral rebound, defined as a higher viral load at day 10 than day 5, was less frequent with metformin (3.28%) than placebo (5.95%; OR, 0.68; 95% CI, .36 to 1.29). The metformin effect was consistent across subgroups and increased over time. Neither ivermectin nor fluvoxamine showed effect over placebo.
In this randomized, placebo-controlled trial of outpatient treatment of SARS-CoV-2, metformin significantly reduced SARS-CoV-2 viral load, which may explain the clinical benefits in this trial. Metformin is pleiotropic with other actions that are relevant to COVID-19 pathophysiology.
NCT04510194.
(See the Invited Commentary by Siedner and Sax on pages 292–4.)
COVID-OUT was a multisite, phase 3, quadruple-blinded, placebo-controlled, randomized clinical trial to test whether outpatient treatment of severe acute respiratory syndrome coronavirus 2 (SARS-CoV-2) prevented severe coronavirus disease 2019 (COVID-19) [1].
The selection of metformin was motivated by in silico modeling, in vitro data, and human lung tissue data that showed that metformin decreased SARS-CoV-2 viral growth and improved cell viability [2–4]. The in silico modeling identified protein translation as a key process in SARS-CoV-2 replication, similar to protein mapping of SARS-CoV-2 [3]. Metformin inhibits the mechanistic target of rapamycin (mTOR) [5], which controls protein translation [6, 7]. Metformin has shown in vitro antiviral actions against the Zika virus and against hepatitis C via mTOR inhibition [8–11].
Severe COVID-19 was defined using a binary, 4-part composite outcome (1 reading <94% SpO2 on a home oximeter/emergency department visit/hospitalization/death) through 14 days and was not significant. After removing the 1 oxygen reading <94% component per the prespecified statistical analysis plan (SAP), metformin reduced the odds of emergency department visits/hospitalizations/death by day 14 by 42%, of hospitalization/death by day 28 by 58%, and of long COVID diagnoses by day 300 by 42% [1, 12].
Here, we present the viral load quantification from samples obtained during the COVID-OUT trial. The trial used a 2 × 3 factorial design of parallel treatments to efficiently assess 3 medications: immediate-release metformin, ivermectin, and fluvoxamine at doses not previously studied in COVID-19 trials.
METHODS
Study Design, Sample, and Oversight
COVID-OUT was an investigator-initiated, multisite, phase 3, quadruple-blinded, placebo-controlled randomized clinical trial (Supplementary Tables 1 and 2) [1] that enrolled from 30 December 2020 to 28 January 2022. COVID-OUT was decentralized to prevent SARS-CoV-2 spread. The participants, care providers, investigators, and outcomes assessors remained blinded to treatment allocation.
Institutional review boards (IRBs) at each site and the Advarra Central IRB approved the protocol. An independent data and safety monitoring board (DSMB) monitored safety and efficacy. All analyses and covariates were prespecified in the SAP, which was submitted to the DSMB before enrollment ended and submitted in February 2022 with the primary outcome manuscript and then published [1]. An independent monitor oversaw study conduct per the Declaration of Helsinki, Good Clinical Practice Guidelines, and local requirements.
COVID-OUT excluded low-risk individuals, limiting enrollment to standard-risk adults aged 30 to 85 years with a body mass index (BMI) in the overweight or obesity categories, documented + SARS-CoV-2 within 3 days, and no prior confirmed SARS-CoV-2 infection. Pregnant women were randomized to metformin or placebo and not to ivermectin or fluvoxamine. Exclusion criteria included hospitalized, symptom onset >7 days prior, and unstable heart, liver, or kidney failure [1].
Metformin dosing was as follows: 500 mg on day 1, 500 mg twice daily on days 2–5, and 500 mg in the morning and 1000 mg in the evening on days 6–14. Fluvoxamine dosing was as follows: 50 mg on day 1 and 50 mg twice daily on days 2–14. Ivermectin dosing was as follows: a median of 430 µg/kg/day (range, 390 to 470 µg/kg/day) for 3 days.
Clinical and Virologic End Points
The primary end point was severe COVID-19 by day 14, defined using a binary, 4-part composite end point: 1 reading <94% SpO2 on home oximeter/emergency department visit/hospitalization/death due to COVID-19. Secondary end points included hospitalization or death by day 28 and long COVID over the 10-month follow-up. The virologic secondary end point was overall viral load in follow-up, adjusted for baseline viral load as prespecified in the SAP.
Self-collection of anterior nares samples was an optional component of the randomized trial. Supply chain shortages caused administrative censoring of 78 participants who did not receive materials for collecting day 1, day 5, or day 10 samples; 3 did not receive materials for day 5 or day 10 samples (Supplementary Figure 1, Supplementary Tables 3–6).
Laboratory Procedures
Participants received written instructions with pictures on self-collecting from the anterior mid-turbinate, which has excellent concordance with professionally collected nasal swabs [13]. Viral load was measured via reverse-transcription quantitative polymerase chain reaction using N1 and N2 targets in the SARS-CoV-2 nucleocapsid protein, with relative cycle threshold values converted to absolute copy number via calibration to droplet digital polymerase chain reaction. Detailed methods can be found in Supplementary Table 7.
While participant self-collection may vary between participants, self-collection of samples is done by the same individual at baseline and follow-up. Thus, participant self-collection may have less variability between baseline and follow-up than when study or clinical staff obtain samples.
Statistical Analyses
We evaluated randomized study drug assignment on the impact of log10-transformed viral load on day 5 and day 10 with a linear Tobit regression model where the effect of study drugs was allowed to differ on day 5 and day 10. This was decided a priori as a rigorous analytic approach to account for left censoring due to the viral load limit of quantification. Repeated measures were accounted for using clustered standard errors within participants. Analyses of viral loads estimated the adjusted mean reduction averaged over time and the adjusted mean reduction at day 5 and day 10. We evaluated impact over time on the probability of viral load being undetectable using generalized estimating equations with a logistic link; estimates are reported as adjusted odds ratios (ORs) and 95% confidence intervals (CIs).
The COVID-OUT trial was a 2 × 3 factorial design of parallel distinct treatments (Supplementary Table 2). All analyses were adjusted for baseline viral load, vaccination status, time since last vaccination for those vaccinated before enrollment, receipt of other study medications within factorial trial, laboratory that processed the nasal swabs, and exact time and date of specimen collection. Additional details and the results of the analyses with dropping of adjustment variables are presented in Supplementary Tables 8 and 9.
To handle missing values, we used multiple imputation with chained equations to multiply impute missing viral load outcomes and vaccination status. Missing covariate information was jointly imputed along with missing outcomes using random forests for the univariate imputation models. Along with outcome and vaccination status information, imputation models were informed by sex, BMI, symptom duration, race/ethnicity, baseline comorbidities, clinical outcomes, and enrollment time categorized by the dominant pandemic variant. Complete case analysis without imputation of missing data is presented in Supplementary Figures 2–4. Heterogeneity of effect was assessed across a priori subgroups of baseline characteristics. Starting metformin in <4 days of symptom onset is a subgroup that aligns with antiviral trials and reflects real-world use, as metformin is widely available.
RESULTS
Among 1323 randomized participants in the COVID-OUT trial, 999 (76%) chose to participate in the optional substudy and provided at least 1 nasal swab sample (Table 1, Supplementary Figure 1). The demographics of the participants who submitted swabs were similar to those who did not submit nasal swabs (Supplementary Tables 3–5). Day 1 samples were provided by 945 participants, 871 provided day 5 samples, and 775 provided day 10 samples (Supplementary Table 6). The overall viral load was a median of 4.88 log10 copies/mL (interquartile range [IQR], 2.99 to 6.18) on day 1, 1.90 (IQR, 0 to 3.93) on day 5, and 0 (IQR, 0 to 1.90 with 0 representing the limit of quantification) on day 10.
Characteristic . | Overall n = 999 . | Placebo n = 495 . | Metformin n = 504 . |
---|---|---|---|
Age | 46 (38–55) | 45 (38–54) | 46 (38–55) |
Biologic sex, female | 56% (559) | 57% (282) | 55% (277) |
Race Native American | 2.2% (22) | 2.6% (13) | 1.8% (9) |
Asian | 3.6% (36) | 3.8% (19) | 3.4% (17) |
Hawaiian, Pacific Islander | 0.7% (7) | 0.4% (2) | 1.0% (5) |
Black or African American | 6.2% (62) | 6.1% (30) | 6.3% (32) |
White | 85% (849) | 85% (420) | 85% (429) |
Other, missing, declined | 5.0% (50) | 4.4% (22) | 5.6% (28) |
Ethnicity, Hispanic | 12% (118) | 13% (63) | 11% (55) |
Medical history | |||
BMI | 30.0 (27.1–34.3) | 30.0 (26.9–34.7) | 29.8 (27.2–34.0) |
BMI ≥30 kg/m2 | 50% (496) | 51% (250) | 49% (246) |
Cardiovascular disease | 28% (282) | 28% (140) | 28% (142) |
Diabetes | 2.0% (20) | 2.6% (13) | 1.4% (7) |
Vaccination status at baseline | |||
No vaccine | 46% (457) | 48% (240) | 43% (217) |
Primary series only | 50% (495) | 47% (232) | 52% (263) |
Monovalent booster | 4.7% (47) | 4.6% (23) | 4.8% (24) |
Days since last vaccine dose | 194 (132–240) | 195 (132–235) | 192 (132–245) |
Time from symptom onset to first dosea | |||
Days, mean (± standard deviation) | 4.7 (±1.9) | 4.7 (±1.8) | 4.7 (±1.9) |
≤4 days | 46% (453) | 48% (230) | 45% (223) |
Severe acute respiratory syndrome coronavirus 2 variant period | |||
Alpha (before 19 June 2021) | 13% (132) | 13% (65) | 13% (67) |
Delta (2021 June 19 2021 to 2021 December 12) | 65% (645) | 65% (320) | 64% (325) |
Omicron (after 2021 December 12,) | 22% (222) | 22% (110) | 22% (112) |
Insurance status | |||
Private | 65% (652) | 65% (324) | 65% (328) |
Medicare | 7.5% (75) | 6.9% (34) | 8.1% (41) |
Medicaid | 14% (136) | 14% (69) | 13% (67) |
No insurance | 12% (123) | 12% (60) | 12% (63) |
Unknown | 1.3% (13) | 1.6% (8) | 1.0% (5) |
Characteristic . | Overall n = 999 . | Placebo n = 495 . | Metformin n = 504 . |
---|---|---|---|
Age | 46 (38–55) | 45 (38–54) | 46 (38–55) |
Biologic sex, female | 56% (559) | 57% (282) | 55% (277) |
Race Native American | 2.2% (22) | 2.6% (13) | 1.8% (9) |
Asian | 3.6% (36) | 3.8% (19) | 3.4% (17) |
Hawaiian, Pacific Islander | 0.7% (7) | 0.4% (2) | 1.0% (5) |
Black or African American | 6.2% (62) | 6.1% (30) | 6.3% (32) |
White | 85% (849) | 85% (420) | 85% (429) |
Other, missing, declined | 5.0% (50) | 4.4% (22) | 5.6% (28) |
Ethnicity, Hispanic | 12% (118) | 13% (63) | 11% (55) |
Medical history | |||
BMI | 30.0 (27.1–34.3) | 30.0 (26.9–34.7) | 29.8 (27.2–34.0) |
BMI ≥30 kg/m2 | 50% (496) | 51% (250) | 49% (246) |
Cardiovascular disease | 28% (282) | 28% (140) | 28% (142) |
Diabetes | 2.0% (20) | 2.6% (13) | 1.4% (7) |
Vaccination status at baseline | |||
No vaccine | 46% (457) | 48% (240) | 43% (217) |
Primary series only | 50% (495) | 47% (232) | 52% (263) |
Monovalent booster | 4.7% (47) | 4.6% (23) | 4.8% (24) |
Days since last vaccine dose | 194 (132–240) | 195 (132–235) | 192 (132–245) |
Time from symptom onset to first dosea | |||
Days, mean (± standard deviation) | 4.7 (±1.9) | 4.7 (±1.8) | 4.7 (±1.9) |
≤4 days | 46% (453) | 48% (230) | 45% (223) |
Severe acute respiratory syndrome coronavirus 2 variant period | |||
Alpha (before 19 June 2021) | 13% (132) | 13% (65) | 13% (67) |
Delta (2021 June 19 2021 to 2021 December 12) | 65% (645) | 65% (320) | 64% (325) |
Omicron (after 2021 December 12,) | 22% (222) | 22% (110) | 22% (112) |
Insurance status | |||
Private | 65% (652) | 65% (324) | 65% (328) |
Medicare | 7.5% (75) | 6.9% (34) | 8.1% (41) |
Medicaid | 14% (136) | 14% (69) | 13% (67) |
No insurance | 12% (123) | 12% (60) | 12% (63) |
Unknown | 1.3% (13) | 1.6% (8) | 1.0% (5) |
Values are percent (n) or median (interquartile range) unless specified. Cardiovascular disease defined as hypertension, hyperlipidemia, coronary artery disease, past myocardial infarction, congestive heart failure, pacemaker, arrhythmias, or pulmonary hypertension.
Abbreviation: BMI, body mass index.
aUnknown n = 22.
Characteristic . | Overall n = 999 . | Placebo n = 495 . | Metformin n = 504 . |
---|---|---|---|
Age | 46 (38–55) | 45 (38–54) | 46 (38–55) |
Biologic sex, female | 56% (559) | 57% (282) | 55% (277) |
Race Native American | 2.2% (22) | 2.6% (13) | 1.8% (9) |
Asian | 3.6% (36) | 3.8% (19) | 3.4% (17) |
Hawaiian, Pacific Islander | 0.7% (7) | 0.4% (2) | 1.0% (5) |
Black or African American | 6.2% (62) | 6.1% (30) | 6.3% (32) |
White | 85% (849) | 85% (420) | 85% (429) |
Other, missing, declined | 5.0% (50) | 4.4% (22) | 5.6% (28) |
Ethnicity, Hispanic | 12% (118) | 13% (63) | 11% (55) |
Medical history | |||
BMI | 30.0 (27.1–34.3) | 30.0 (26.9–34.7) | 29.8 (27.2–34.0) |
BMI ≥30 kg/m2 | 50% (496) | 51% (250) | 49% (246) |
Cardiovascular disease | 28% (282) | 28% (140) | 28% (142) |
Diabetes | 2.0% (20) | 2.6% (13) | 1.4% (7) |
Vaccination status at baseline | |||
No vaccine | 46% (457) | 48% (240) | 43% (217) |
Primary series only | 50% (495) | 47% (232) | 52% (263) |
Monovalent booster | 4.7% (47) | 4.6% (23) | 4.8% (24) |
Days since last vaccine dose | 194 (132–240) | 195 (132–235) | 192 (132–245) |
Time from symptom onset to first dosea | |||
Days, mean (± standard deviation) | 4.7 (±1.9) | 4.7 (±1.8) | 4.7 (±1.9) |
≤4 days | 46% (453) | 48% (230) | 45% (223) |
Severe acute respiratory syndrome coronavirus 2 variant period | |||
Alpha (before 19 June 2021) | 13% (132) | 13% (65) | 13% (67) |
Delta (2021 June 19 2021 to 2021 December 12) | 65% (645) | 65% (320) | 64% (325) |
Omicron (after 2021 December 12,) | 22% (222) | 22% (110) | 22% (112) |
Insurance status | |||
Private | 65% (652) | 65% (324) | 65% (328) |
Medicare | 7.5% (75) | 6.9% (34) | 8.1% (41) |
Medicaid | 14% (136) | 14% (69) | 13% (67) |
No insurance | 12% (123) | 12% (60) | 12% (63) |
Unknown | 1.3% (13) | 1.6% (8) | 1.0% (5) |
Characteristic . | Overall n = 999 . | Placebo n = 495 . | Metformin n = 504 . |
---|---|---|---|
Age | 46 (38–55) | 45 (38–54) | 46 (38–55) |
Biologic sex, female | 56% (559) | 57% (282) | 55% (277) |
Race Native American | 2.2% (22) | 2.6% (13) | 1.8% (9) |
Asian | 3.6% (36) | 3.8% (19) | 3.4% (17) |
Hawaiian, Pacific Islander | 0.7% (7) | 0.4% (2) | 1.0% (5) |
Black or African American | 6.2% (62) | 6.1% (30) | 6.3% (32) |
White | 85% (849) | 85% (420) | 85% (429) |
Other, missing, declined | 5.0% (50) | 4.4% (22) | 5.6% (28) |
Ethnicity, Hispanic | 12% (118) | 13% (63) | 11% (55) |
Medical history | |||
BMI | 30.0 (27.1–34.3) | 30.0 (26.9–34.7) | 29.8 (27.2–34.0) |
BMI ≥30 kg/m2 | 50% (496) | 51% (250) | 49% (246) |
Cardiovascular disease | 28% (282) | 28% (140) | 28% (142) |
Diabetes | 2.0% (20) | 2.6% (13) | 1.4% (7) |
Vaccination status at baseline | |||
No vaccine | 46% (457) | 48% (240) | 43% (217) |
Primary series only | 50% (495) | 47% (232) | 52% (263) |
Monovalent booster | 4.7% (47) | 4.6% (23) | 4.8% (24) |
Days since last vaccine dose | 194 (132–240) | 195 (132–235) | 192 (132–245) |
Time from symptom onset to first dosea | |||
Days, mean (± standard deviation) | 4.7 (±1.9) | 4.7 (±1.8) | 4.7 (±1.9) |
≤4 days | 46% (453) | 48% (230) | 45% (223) |
Severe acute respiratory syndrome coronavirus 2 variant period | |||
Alpha (before 19 June 2021) | 13% (132) | 13% (65) | 13% (67) |
Delta (2021 June 19 2021 to 2021 December 12) | 65% (645) | 65% (320) | 64% (325) |
Omicron (after 2021 December 12,) | 22% (222) | 22% (110) | 22% (112) |
Insurance status | |||
Private | 65% (652) | 65% (324) | 65% (328) |
Medicare | 7.5% (75) | 6.9% (34) | 8.1% (41) |
Medicaid | 14% (136) | 14% (69) | 13% (67) |
No insurance | 12% (123) | 12% (60) | 12% (63) |
Unknown | 1.3% (13) | 1.6% (8) | 1.0% (5) |
Values are percent (n) or median (interquartile range) unless specified. Cardiovascular disease defined as hypertension, hyperlipidemia, coronary artery disease, past myocardial infarction, congestive heart failure, pacemaker, arrhythmias, or pulmonary hypertension.
Abbreviation: BMI, body mass index.
aUnknown n = 22.
The overall mean SARS-CoV-2 viral load reduction with metformin was −0.56 log10 copies/mL (95% CI, −1.05 to −0.06) greater than placebo across all follow-up (P = .027). The antiviral effect of metformin compared with placebo was −0.47 log10 copies/mL (95% CI, −0.93 to −0.014) on day 5 and −0.64 log10 copies/mL (95% CI, −1.42 to 0.13) on day 10 (Figure 1). Neither ivermectin nor fluvoxamine had virologic effect (Figure 2, Supplementary Figure 2, Supplementary Tables 8–10).
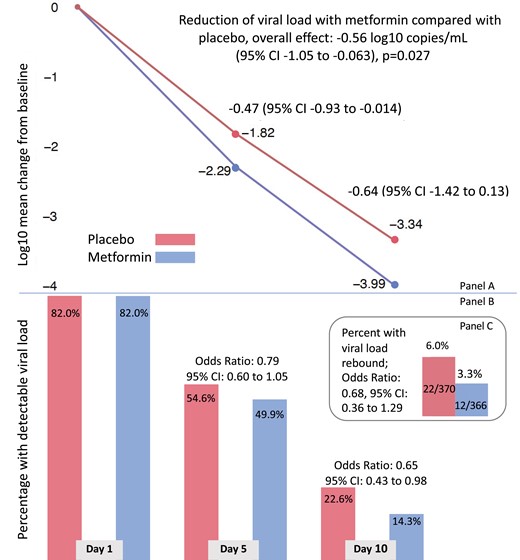
Effect of metformin versus placebo on viral load over time, detectable viral load, and rebound viral load. A, Adjusted mean change in log10 copies per milliliter (viral load) from baseline (day 1) to day 5 and day 10 for metformin (lower line) and placebo (upper line). Mean change estimates are based on the adjusted, multiply imputed Tobit analysis (the primary analytic approach) that corresponds to the overall metformin analysis presented in Figure 2. B, Adjusted percent of viral load samples that were detectable at day 1, day 5, and day 10. The percent viral load detected estimates were based on the adjusted, multiply imputed logistic generalized estimating equations (GEE) analysis corresponding to the overall metformin analysis depicted in Figure 3. Odds ratios correspond to adjusted effects on the odds ratio scale. C, Bar chart depicting the percent of participants whose day 10 viral load was greater than the day 5 viral load and the odds ratio for having viral load rebound using the multiply imputed logistic GEE. Abbreviation: CI, confidence interval.
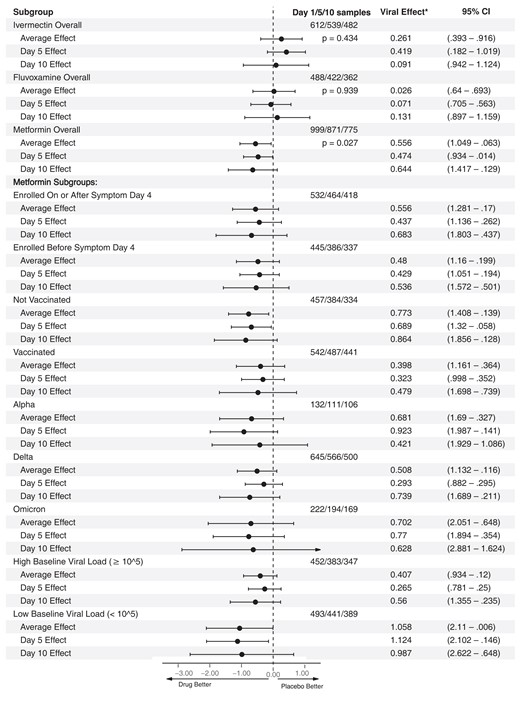
Overall results for metformin, ivermectin, and fluvoxamine on viral load; heterogeneity of treatment effect of metformin versus placebo. This is a forest plot that depicts the effect of active medication compared with control on log10 copies per milliliter (viral load), overall, and at day 5 and day 10. Viral Effect* denotes the adjusted mean change in viral load in log10 copies per milliliter with 95% confidence intervals for the adjusted mean change. Analyses were conducted using the primary analytic approach, a multiply imputed Tobit model. The vertical dashed line indicates the value for a null effect. The top 3 rows show ivermectin, the next 3 rows show fluvoxamine, and the following 3 rows show metformin. Below these, the effect of metformin compared with placebo is shown by a priori subgroups of baseline characteristics. Abbreviation: CI, confidence interval.
When the adjustment covariates were dropped one at a time—baseline viral load, vaccination status, time since last vaccination, other study medications within the factorial trial, and the laboratory processing the nasal swabs—in addition to dropping all adjustment covariates, the results were similar. The range in the estimated average effect was −0.51 log10 copies/mL (95% CI, −1.04 to 0.01; P = .056) to −0.66 log10 copies/mL (95% CI, −1.215 to −0.097; P = .021) with the latter arising from the unadjusted model (Supplementary Table 9).
Those in the metformin group were less likely to have a detectable viral load than those in the placebo group (OR, 0.72; 95% CI, .55 to .94; Figure 1). This effect was higher at day 10 (OR, 0.65; 95% CI, .43 to .98) when 1500 mg/d of metformin was being prescribed than at day 5 (OR, 0.79; 95% CI, .60 to 1.05) when 1000 mg/d was prescribed. Viral rebound was defined as having a higher viral load at day 10 than day 5. In the placebo group, 5.95% (22 of 370) of participants had viral rebound compared with 3.28% (12 of 366) in the metformin group (adjusted OR, .68; 95% CI, .36 to 1.29) for metformin compared with placebo (Figure 1).
Metformin's effect on continuous viral load and conversion to undetectable viral load was consistent across a priori identified subgroups of baseline characteristics (Figures 2 and 3). Subgroups should be interpreted with caution because of low power, risk of making multiple comparisons without correction, and sparse data bias. One subgroup warrants additional detail for interpretation. The antiviral effect on geometric log10 scale was greater among those with baseline viral loads <100 000 copies/mL (mean −1.17 log10 copies/mL reduction) than among those with >100 000 copies/mL (mean −0.49 log10 copies/mL reduction); although the reduction in absolute copies per milliliter would be greater among those with higher viral loads (Figures 2 and 3). Mean, median viral load levels are presented in Supplementary Table 11; sensitivity analyses are presented in Supplementary Figures 5–7 and Supplementary Table 12.

Overall results for metformin, ivermectin, and fluvoxamine on detectability of viral load; heterogeneity of treatment effect of metformin versus placebo. This is a forest plot that depicts the effect of active medication compared with control on the proportion of participants with a detectable viral load, overall and at days 5 and 10. Estimate* denotes the adjusted mean risk difference in the percent of samples with detected viral load with 95% confidence intervals for the adjusted risk difference. The vertical dashed line indicates the value for a null effect. The estimated risk differences are derived from the adjusted, multiply imputed logistic generalized estimating equations (GEE) analytic approach. The top 3 rows show ivermectin, the next 3 rows show fluvoxamine, and the following 3 rows show metformin. Below these, the effect of metformin compared with placebo is shown by a priori subgroups of baseline characteristics. Abbreviation: CI, confidence interval.
DISCUSSION
In the virologic end point of the COVID-OUT phase 3, randomized trial, metformin significantly reduced SARS-CoV-2 viral load over 10 days [1]. The mean reduction was −0.56 log10 copies/mL greater than placebo. The antiviral response is consistent with the statistically significant and clinically relevant effects of metformin in preventing clinical outcomes: severe COVID-19 (emergency department visit, hospitalization, or death) through day 14, hospitalization or death by day 28, and the diagnosis of long COVID [1, 12]. The magnitude of effect on clinical outcomes was larger when metformin was started earlier in the course of infection at <4 days from symptom onset, with metformin reducing the odds of severe COVID-19 by 55% (OR, 0.45; 95% CI, .22 to .93) and of long COVID by 65% (hazard ratio = 0.35; 95% CI, .15 to .95; Figure 4). An improved effect size for clinical outcomes when therapies are started earlier in the course of infection is consistent with an antiviral action [14].
![Overview of results from the COVID-OUT trial. This is a forest plot that combines the severe, acute coronavirus disease 2019 outcome as well as the long-term follow-up outcome from the COVID-OUT trial [1, 12]. Two a priori subgroups from the COVID-OUT trial are also presented: pregnant individuals and those who started the study drug within 4 days of symptom onset, to match the primary analytic sample of other antivirals. Abbreviations: COVID-19, coronavirus disease 2019; ITT, intention to treat; mITT, modified intention to treat; SARS-CoV-2, severe acute respiratory syndrome coronavirus 2.](https://oup.silverchair-cdn.com/oup/backfile/Content_public/Journal/cid/79/2/10.1093_cid_ciae159/1/m_ciae159f4.jpeg?Expires=1750825048&Signature=yb9bJnC7EDGdkXxHuHKE-Geg~28SdvsM~BaFUeeh5BKRf4ZytkjWyDkXzsVeuumKSZPaLtmZGcvVCY2mBs3CnEN~D96Et9lp6UqwPBIqIppAlUgKfuC66btpw58I6sEeNAU88fxYyxMEwMEJJcq78ixrzAGSWLQKXJshcAqmAkUzrfZ0segQjmSW8lh5O6Jjq~tvrJ1JCwAa7maJ6MZoxKVE4xUi-GTv8~QLuhe-FoWzlTXs1EcF1XHhcoVsYG86oaDDl-YlIflO7pmlWqHD46x0pJIMhrpl2Zw3KdL2Tk6a6lk0N-FfkrygFjSlKF6jSfd01bYQtzMxlTxGzkCBPQ__&Key-Pair-Id=APKAIE5G5CRDK6RD3PGA)
Overview of results from the COVID-OUT trial. This is a forest plot that combines the severe, acute coronavirus disease 2019 outcome as well as the long-term follow-up outcome from the COVID-OUT trial [1, 12]. Two a priori subgroups from the COVID-OUT trial are also presented: pregnant individuals and those who started the study drug within 4 days of symptom onset, to match the primary analytic sample of other antivirals. Abbreviations: COVID-19, coronavirus disease 2019; ITT, intention to treat; mITT, modified intention to treat; SARS-CoV-2, severe acute respiratory syndrome coronavirus 2.
The objective of the COVID-OUT trial was to determine whether metformin prevented severe COVID-19. Severe COVID-19 was defined with a binary, 4-part composite outcome (<94% SpO2 on a home oximeter/emergency department visit/hospitalization/death) at a time when the implications of “silent hypoxia” were unknown and fears of overwhelmed emergency services caused concern that deaths would occur at home before patients reached the emergency department. As a scientific community, we now understand that 1 reading below 94% is not severe COVID-19. An accurate definition of severe COVID-19 (emergency department visit/hospitalization/death) was ascertained within the same data-generation process. In such situations, recommendations are sometimes made based on the totality of evidence from a single randomized trial [15–17].
The antiviral effect in this phase 3, randomized trial is also consistent with emerging data from other trials. In a phase 2, randomized trial with 20 participants, the metformin group had better clinical outcomes, achieved an undetectable viral load 2.3 days faster than placebo (P = .03), and had a larger proportion of patients with an undetectable viral load at 3.3 days in the metformin group (P = .04) [18]. A recent in vitro study showed that metformin decreased infectious SARS-CoV-2 titers and viral RNA in 2 cell lines, Caco2 and Calu3, at a clinically appropriate concentration [19].
Conversely, an abandoned randomized trial testing extended-release metformin 1500 mg/d without a dose titration did not report improved SARS-CoV-2 viral clearance at day 7 [20]. Several differences between the Together Trial and the COVID-OUT trial are important for understanding the data. First, the Together Trial allowed individuals already taking metformin to enroll and be randomized to placebo or more metformin [20, 21]. To compare starting metformin versus placebo, the authors excluded those already taking metformin at baseline and reported that emergency department visit or hospitalization occurred in 9.2% (17 of 185) randomized to metformin compared with 14.8% (27 of 183) randomized to placebo (relative risk, 0.63; 95% confidence interval, .35 to 1.10, Probability of superiority = 0.949) [22]. Thus, the Together Trial results for starting metformin versus placebo are similar. Second, 1500 mg/day without escalating the dose over 6 days would cause side effects, especially if the study participant was already taking metformin [23]. Third, extended-release and immediate-release metformin have different pharmacokinetic properties. Immediate-release metformin has higher systemic exposure than extended-release metformin, which may improve antiviral actions, but this is not known [24, 25]. Given the similar clinical outcomes between immediate and extended-release, a direct comparison of the 2 may be important for understanding pharmacokinetics against SARS-CoV-2.
In comparison with other SARS-CoV-2 antivirals, when considering all enrolled participants, at day 5, the antiviral effect over placebo was 0.47 log10 copies/mL for metformin, 0.30 log10 copies/mL for molnupiravir, and 0.80 log10 copies/mL for nirmatrelvir/ritonavir [26, 27]. At day 10, the viral load reduction over blinded placebo was 0.64 log10 copies/mL for metformin, 0.35 log10 copies/mL for nirmatrelvir, and 0.19 log10 copies/mL for molnupiravir [26, 27]. We note that the 3 trials enrolled different populations and at different times and locations during the pandemic. In the COVID-OUT metformin trial, half were vaccinated [1, 12].
The magnitude of metformin's antiviral effect was larger at day 10 than at day 5 overall and across subgroups, which correlates with the dose titration from 1000 mg on days 2–5 to 1500 mg on days 6–14. The dose titration to 1500 mg over 6 days used in the COVID-OUT trial was faster than typical use. When used chronically, that is, for diabetes, prediabetes, or weight loss, metformin is slowly titrated to 2000 mg daily over 4–8 weeks. While metformin's effect on diabetes control is not consistently dose-dependent, metformin's gastrointestinal side effects are known to be dose-dependent [25]. Thus, despite what appears to be dose-dependent antiviral effects, a faster dose titration should likely only be considered in individuals with no gastrointestinal side effects from metformin.
When assessing for heterogeneity of effect, metformin was consistent across subgroups. Metformin's antiviral effect in vaccinated versus unvaccinated of −0.48 versus −0.86 log10 copies/mL at day 10 mirrors nirmatrelvir, for which the effect in seropositive participants was smaller than in the overall trial population, −0.13 versus −0.35 log10 copies/mL at day 10 [26]. Effective primed memory B- and T-cell anamnestic immunity prompting effective response by day 5 in vaccinated persons may account for this trend in both trials. Subgroups should be interpreted with caution because of low power and multiple comparisons [28].
Both nirmatrelvir and molnupiravir are pathogen-directed antiviral agents. Therapeutics may have an important role in targeting host factors rather than viral factors, as targeting the host may be less likely to induce drug-resistant viral variants through mutation–selection [11, 29]. We did not study the mechanism for the antiviral activity or an antiinflammatory action in this trial. Previous work has shown that metformin's inhibition of mTOR complex 1 may depend on AMP-activated protein kinase (AMPK) at low doses but not high doses [5]. An AMPK-independent inhibition of mTOR may be more efficient. Additionally, metformin demonstrates a dose-dependent ability to inhibit interleukin (IL)-1, IL-6, and tumor necrosis factor-alpha in the presence of lipopolysaccharide, inflammatory products that correlate with COVID-19 severity [30, 31].
In addition to antiviral activity, metformin appears to have relevant antiinflammatory actions. In mice without diabetes, metformin inhibited mitochondrial ATP and DNA synthesis to evade NLRP3 inflammasome activation [32]. In macrophages of mice without diabetes infected with SARS-CoV-2, metformin inhibited inflammasome activation, IL-1 production, and IL-6 secretion and also increased the IL-10 antiinflammatory response to lipopolysaccharide, thereby attenuating lipopolysaccharide-induced lung injury [32]. In a recent assay of human lung epithelial cell lines, metformin inhibited the cleavage of caspase-1 by NSP6, inhibiting the maturation and release of IL-1, a key factor that mediates inflammatory responses [7]. The idea of pleiotropic effects is being embraced in novel therapeutics being developed for both antiviral and anti-inflammatory actions [33].
Strengths of our study include the large sample size and detailed participant information collected, including the exact time and date of specimen collection. One limitation was the sampling time frame of only day 1, day 5, and day 10 due to limited resources. By day 10 post-randomization, 77% of participants in the placebo group and 86% in the metformin group had an undetectable viral load. As viral load is lower in vaccinated persons [34], this degree of undetectable viral loads differs from findings from earlier clinical trials conducted in unvaccinated participants without known prior infection [26, 27]. Sampling earlier and more frequently, that is, day 1, day 3, day 6, and day 9 in future trials, may better characterize differences in viral shedding earlier in the infection and over time, dependent on the duration of therapy and timing of enrollment.
Future work could assess whether synergy exists between metformin and direct SARS-CoV-2 antivirals, as previous work showed that metformin improved sustained virologic clearance of hepatitis C virus and improved outcomes in other respiratory infections [35–37]. The biophysical modeling that motivated this trial predicts additive/cooperative effects in combination with transcription inhibitors. Combination therapy might decrease selective pressure, and metformin has few medication interactions, so treatment with metformin could continue beyond 5 days while home medications are restarted. Additionally, continuing metformin could reduce symptom rebound, given its effects on T-cell immunity [38, 39]. Further data are needed to understand whether decreased viral load and faster viral clearance decrease onward transmission of SARS-CoV-2.
Metformin is safe in children and pregnant individuals with and without preexisting diabetes [40–42]. Individuals with or without diabetes do not need to check blood sugar when taking metformin. Historical concerns about lactic acidosis were driven by other biguanides; metformin does not increase risk of lactic acidosis [43]. Metformin improves outcomes in patients with heart, liver, and kidney failure, as well as during hospitalizations and perioperatively [44–48].
CONCLUSIONS
In a large randomized, controlled trial conducted in nonhospitalized, standard-risk adults, metformin reduced the incidence of severe COVID-19 by day 14, of hospitalizations by day 28, and of long COVID diagnosis by day 300. In this virologic analysis, we found a corresponding significant reduction in viral load with metformin compared with placebo and a lower likelihood of viral load rebound. While 22% of participants in the trial were enrolled during the Omicron era, metformin has not been assessed in individuals with a history of prior infection and thus should be trialed in the current state of the pandemic. Metformin is currently being trialed in low-risk adults [49].
Supplementary Data
Supplementary materials are available at Clinical Infectious Diseases online. Consisting of data provided by the authors to benefit the reader, the posted materials are not copyedited and are the sole responsibility of the authors, so questions or comments should be addressed to the corresponding author.
Notes
Disclaimer. The funders had no influence on the design or conduct of the trial and were not involved in data collection or analysis, writing of the manuscript, or decision to submit for publication. The authors assume responsibility for trial fidelity and the accuracy and completeness of the data and analyses.
Financial support. The fluvoxamine placebo tablets were donated by the Apotex Pharmacy. The ivermectin placebo and active tablets were donated by the Edenbridge Pharmacy. The trial was funded by the Parsemus Foundation, Rainwater Charitable Foundation, Fast Grants, and the UnitedHealth Group Foundation. C. T. B. was supported by grants (KL2TR002492 and UL1TR002494) from the National Center for Advancing Translational Sciences (NCATS) of the National Institutes of Health (NIH) and by a grant (K23 DK124654) from the National Institute of Diabetes and Digestive and Kidney Diseases of the NIH. J. B. B. was supported by a grant (UL1TR002489) from NCATS. J. M. N. was supported by a grant (K23HL133604) from the National Heart, Lung, and Blood Institute (NHLBI) of the NIH. D. J. O. was supported by the Institute for Engineering in Medicine, University of Minnesota Office of Academic and Clinical Affairs COVID-19 Rapid Response Grant, the Earl E. Bakken Professorship for Engineering in Medicine, and by grants (U54 CA210190 and P01 CA254849) from the National Cancer Institute of the NIH. D. M. L. receives funding from NIH RECOVER (OT2HL161847). L. K. S. was supported by NIH grants (18X107CF6 and 18X107CF5) through a contract with Leidos Biomedical and by grants from the HLBI of the NIH (T32HL129956) and the NIH (R01LM012982 and R21LM012744). M. A. P. receives grants from the Bill and Melinda Gates Foundation (INV-017069), Minnesota Partnership for Biotechnology and Medical Genomics (00086722) and NHLBI (OT2HL156812).
References
Expression of concern – Effect of early treatment with metformin on risk of emergency care and hospitalization among patients with COVID-19: the TOGETHER randomized platform clinical trial.
Author notes
D. R. B. and J. D. H. contributed equally to this work.
Potential conflicts of interest. J. B. B. reports contracted fees and travel support for contracted activities for consulting work paid to the University of North Carolina by Novo Nordisk; grant support by NIH, PCORI, Bayer, Boehringer-Ingelheim, Carmot, Corcept, Dexcom, Eli Lilly, Insulet, MannKind, Novo Nordisk, and vTv Therapeutics; personal compensation for consultation from Alkahest, Altimmune, Anji, Aqua Medical Inc, AstraZeneca, Boehringer-Ingelheim, CeQur, Corcept Therapeutics, Eli Lilly, embecta, GentiBio, Glyscend, Insulet, Mellitus Health, Metsera, Moderna, Novo Nordisk, Pendulum Therapeutics, Praetego, Stability Health, Tandem, Terns Inc, and Vertex.; personal compensation for expert testimony from Medtronic MiniMed; participation on advisory boards for Altimmune, AstraZeneca, and Insulet; a leadership role for the Association of Clinical and Translational Science; and stock/options in Glyscend, Mellitus Health, Pendulum Therapeutics, Praetego, and Stability Health. M. A. P. receives consulting fees from Opticyte and Cytovale. A. B. K. has served as an external consultant for Roche Diagnostics; received speaker honoraria from Siemens Healthcare Diagnostics, the American Kidney Fund, the National Kidney Foundation, the American Society of Nephrology, and Yale University Department of Laboratory Medicine; research support unrelated to this work from Siemens Healthcare Diagnostics, Kyowa Kirin Pharmaceutical Development, the Juvenile Diabetes Research Foundation, and the NIH; support for travel from College of American Pathologists Point-Of-Care Testing Committee; participation on an advisory board for the Minnesota Newborn Screening Advisory Committee; grants from NIH and JDRF for multiple unrelated clinical research projects and Kyowa Kirin Pharmaceutical Development and Siemens Healthcare Diagnostics for unrelated clinical research studies; and leadership roles for the American Board of Clinical Chemistry, Association for Diagnostics and Laboratory Medicine (ADLM) Evidence-Based Laboratory Medicine Subcommittee, and ADLM Academy Test Utilization Committee. M. R. R. reports consulting fees from 20/20 Gene Systems for coronavirus disease 2019 testing. D. B. R. reports grants from the NIH NCATS ACTIV-6 Steering Committee Chair. K. C. reports stock or stock options for United Health Group. C. T. B. reports consulting fees from NCATS/DCRI and the ACTIV-6 Executive Committee and support for travel from Academic Medical Education. All other authors report no potential conflicts.
All authors have submitted the ICMJE Form for Disclosure of Potential Conflicts of Interest. Conflicts that the editors consider relevant to the content of the manuscript have been disclosed.