-
PDF
- Split View
-
Views
-
Cite
Cite
Constantina Boikos, Gregg C Sylvester, John S Sampalis, James A Mansi, Relative Effectiveness of the Cell-Cultured Quadrivalent Influenza Vaccine Compared to Standard, Egg-derived Quadrivalent Influenza Vaccines in Preventing Influenza-like Illness in 2017–2018, Clinical Infectious Diseases, Volume 71, Issue 10, 15 November 2020, Pages e665–e671, https://doi.org/10.1093/cid/ciaa371
- Share Icon Share
Abstract
Influenza antigens may undergo adaptive mutations during egg-based vaccine production. In the 2017–2018 influenza season, quadrivalent, inactivated cell-derived influenza vaccine (ccIIV4) vaccine was produced using A(H3N2) seed virus propagated exclusively in cell culture, thus lacking egg adaptive changes. This United States study estimated relative vaccine effectiveness (rVE) of ccIIV4 vs egg-derived quadrivalent vaccines (egg-derived IIV4) for that season.
Vaccination, outcome, and covariate data were ascertained retrospectively from a electronic medical record (EMR) dataset and analyzed. The study cohort included patients ≥ 4 years of age. rVE was estimated against influenza-like illness (ILI) using diagnostic International Classification of Diseases, Ninth or Tenth Revision codes. The adjusted odds ratios used to derive rVE estimates were estimated from multivariable logistic regression models adjusted for age, sex, race/ethnicity, geographic region, and health status.
Overall, 92 187 individuals had a primary care EMR record of ccIIV4 and 1 261 675 had a record of egg-derived IIV4. In the ccIIV4 group, 1705 narrowly defined ILI events occurred, and 25 645 occurred in the standard egg-derived IIV4 group. Crude rVE was 9.2% (95% confidence interval [CI], 4.6%–13.6%). When adjusted for age, sex, health status, comorbidities, and geographic region, the estimated rVE changed to 36.2% (95% CI, 26.1%–44.9%).
ccIIV4, derived from A(H3N2) seed virus propagated exclusively in cell culture, was more effective than egg-derived IIV4 in preventing ILI during the 2017–2018 influenza season. This result suggests that cell-derived influenza vaccines may have greater effectiveness than standard egg-derived vaccines.
The United States (US) Centers for Disease Control and Prevention (CDC) estimates that influenza has resulted in 9.2–60.8 million illnesses, 140 000–710 000 hospitalizations, and 12 000–56 000 deaths annually in the US since 2010 [1]. Although influenza vaccines include the expected circulating strains each year, the effectiveness of standard, egg-derived seasonal influenza vaccines varies. For the 2017–2018 US season, the estimated interim influenza vaccine effectiveness (VE) was 40%, with the lowest strain-specific effectiveness estimated at 24% against influenza A(H3N2)—the dominant strain in the US that season [2].
While the gold-standard outcome for influenza VE is laboratory-confirmed influenza infection (typically by reverse-transcription polymerase chain reaction [PCR]), multiple studies have sought to predict influenza from among cases of influenza-like illness (ILI) based upon clinical data, with varied success [3–8]. Nonetheless, ILI accounts for a large portion of illness, work loss, and impaired work performance during the influenza season [9]. ILI has also been associated with impaired school performance among college students [10] and impaired productivity while at work among healthy working adults [11, 12].
Most contemporary influenza virus vaccines are produced from viruses grown in embryonated chicken eggs [13, 14]. In response to the selective pressures in the eggs [13, 14], mutations accumulate in the hemagglutinin proteins of influenza viruses propagated in avian cells, most notably for influenza A(H3N2) viruses [15–18].. These mutations alter glycosylation and impair the neutralizing antibody response and are believed to reduce influenza VE [15, 19–21]. Furthermore, evidence from a retrospective analysis of the 2002–2018 influenza seasons suggests that such mutations have affected antigenicity against H3N2 viruses during a number of past influenza seasons [22].
The first quadrivalent, cell-derived inactivated influenza vaccine (ccIIV4) has been approved in the US (Flucelvax Quadrivalent, Seqirus) and in the European Union (Flucelvax Tetra, Seqirus). This ccIIV4 is manufactured from influenza viruses propagated in Madin-Darby canine kidney cells [23–25], rather than avian cells, and has been demonstrated to induce a similar immune responses compared with previously licensed trivalent cell-derived vaccines [26, 27]. Using mammalian cells to propagate vaccine-strain influenza viruses reduces the chance of mutations that arise during the vaccine production process. As a result, cell culture technology improves the match between the vaccine virus strain and the vaccine selected strain, and has been hypothesized to increased VE.
Whilst some comparative effectiveness studies have been published [28–32], more are needed to elucidate the clinical and public health benefits of ccIIV4. The 2017–2018 season presented the first opportunity to evaluate the relative clinical benefit of ccIIV4. The 2017–2018 influenza season in the US was the first season that the A(H3N2) vaccine strain was fully cell-derived (including the vaccine seed virus), thus lacking egg adaptive changes notably prominent with this strain; the other 3 flu strains originated from egg isolates in that season [33]. The objective of this study was thus to estimate the relative benefit of ccIIV4 vs standard, egg-derived, quadrivalent, seasonal influenza vaccines (egg-derived IIV4) against ILI using real-world, observational data in the 2017–2018 influenza season.
METHODS
Study Design
Data were derived retrospectively from Allscripts electronic medical records (EMRs) from primary care practices in the US and analyzed using a retrospective cohort study design. The EMR platform used is one of the largest in the US with a catchment population of > 55 million patients and is considered representative of the US population based on the US Census/National Ambulatory Medical Care Survey [34]. EMR records include data on patient history, medications, diagnoses, procedures, vital signs, and diagnostic International Classification of Diseases, Ninth Revision (ICD-9) and International Classification of Diseases, Tenth Revision (ICD-10) codes that describe illnesses and immunizations.
Study Population and Time Period
The study cohort included all patients ≥ 4 years of age who presented for primary care at a medical facility enrolled in the Allscripts Health Insights research database. Health Insights is a de-identified database derived from Allscripts Touchworks or PRO ambulatory care EMR systems. The Allscripts EMRs are used at approximately 5% of outpatient sites throughout the US. Healthcare providers who participate in Health Insights have agreed to make patients’ de-identified clinical records available for retrospective observational research. Given this agreement, informed consent was not necessary or obtained from individual patients. The time period for the study (ie, the influenza season) was considered to be 1 August 2017 to 30 March 2018, as this corresponded to the majority of the influenza season as described by the CDC.
Exposure
Exposure ascertainment (receipt of either ccIIV4 or egg-derived IIV4) was derived from subjects’ EMR records using Current Procedural Terminology and National Drug Codes to identify specific influenza vaccines. Only subjects with a complete record of immunization (including date of vaccine receipt) in their EMR were included in the study cohort. Individuals were considered fully vaccinated 14 days after administration of the seasonal influenza vaccine and were represented only once in the dataset. Of note, an unvaccinated cohort was not evaluated as a comparator group in this study as it was not possible to validate the absence of a flu record in a subject’s EMR [35, 36].
Outcome
The outcome evaluated was ILI recorded within primary care practice, defined by presence of specific diagnostic codes in subject primary care EMR database. The EMR database used is expected to capture primary care visits for ILI as EMRs are intended to document patient interactions with their primary care providers, including visits for ILI. A subject was considered not to have the outcome of interest if there was no record for an ILI in the subject’s EMR during the study period.
The diagnostic codes used to ascertain ILI status were derived from published and validated diagnostic code sets based on ICD codes evaluated by the US Armed Forces Health Surveillance Center (AFHSC) [37]. The AFHSC describes 2 groups of ICD codes: Code Set A and Code Set B. Code Set B is based on diagnostic ICD codes specific to influenza (ICD-10 codes: J09*–J11* in any diagnostic position) and thus represents a narrower definition of ILI. The primary outcome was identified a priori as narrowly defined ILI (Code Set B), which was shown to have a higher positive predictive value for laboratory-confirmed influenza. See Supplementary Data (ILI Definitions and Coding) for information on Code Set A and the sensitivity and specificity of the AFHSC ILI outcomes.
Variables of Interest
Confounders of the association of interest were identified a priori. Data on preidentified covariates were ascertained from each subject’s EMR on age (continuous); sex (binary); race/ethnicity (African American, white, Hispanic, other); health status; and geographic regions.
Statistical Analyses
Descriptive statistics were evaluated for all covariates by vaccination group (ccIIV4 and egg-derived IIV4). Continuous and categorical variables were reported as mean (standard deviation) and proportional values, respectively. Crude relative VE (rVE) was estimated by running a univariable logit model.
Primary Analytical Model
The main analysis model was identified a priori as a multivariable logistic regression model. The model building process began by evaluating the Hosmer-Lemeshow model fit statistic and the statistical significance (α = .05) of all identified confounding variables. Specifically, 1 categorical variable for race/ethnicity was included in the model (categories: white, black, Hispanic, other). Similarly, 1 categorical variable was included for geographic location. Originally, 10 categories were included in the variable (1 for each of the 10 Department of Health and Human Services regions); however, these 10 regions were further grouped based on ILI incidence (Supplementary Table 1). Furthermore, health status was adjusted for by evaluating each of the 17 comorbidity categories of the Charlson comorbidity index [38] as distinct, binary variables. Values for each of the 17 binary variables were ascertained based on the presence of diagnostic ICD codes in a subject’s EMR within 5 years of vaccination index date. Of note, some comorbidity variables were not included in the final model despite statistical significance given very small sample sizes within these comorbid categories. Information on the exact comorbidity variables, and region categories, included in the final model are included in Supplementary Tables 2–5. Last, 1 continuous variable (age) was included in the model; square and inverse transformations of age were tested to determine any improvements model fit using the Hosmer-Lemeshow χ 2 statistic. The model with the best fit of age with the dependent variable was used in the final analysis. An age × vaccine interaction term was tested in the final model based on biological plausibility and was retained based on statistical significance (P = .05).
Following selection of the most parsimonious model with the best fit, the odds ratio (OR) was estimated using 1 multivariable, logistic regression model for each of the 2 ILI outcomes. Finally, the adjusted rVE was calculated as 100% × (1 – OR), with the adjusted OR estimated from multivariable logistic regression model. Only results evaluating ILI defined narrowly are presented in the body of the manuscript; results from broadly defined ILI (AFHSC’s Code Set A) are presented in the Supplementary Data.
Missing Data
All subjects have complete information on influenza immunizations, ILI, and race/ethnicity. Multiple imputation of missing values was conducted only for the sex variable. Statistics are based on all cases with valid data for age and geographic location. With respect to comorbidities, when no record of a comorbidity was found in the dataset, the subject was classified as not having the condition.
Additional Analyses
A conditional logistic regression model using propensity score (PS)–matched pairs was run as a second, confirmatory analysis for the overall cohort (all ages) and for the following age strata: 4–17, 18–64, and ≥ 65 years. Details on the exact methodology can be found in Supplementary Table 6. All analyses were conducted using SPSS version 25.
RESULTS
In the study cohort, a total of 1 353 862 seasonal influenza vaccinations were administered; 92 187 (6.8%) were ccIIV4 and 1 261 675 (93%) were egg-derived IIV4. The mean age of the study cohort was 39.8 years (95% confidence interval [CI], 39.8–39.9 years) and the median age was was 44.0 years (SD, 24.5 years). Overall 55% of all vaccinations were given to individuals between 18 and 64 years of age (Table 1). The proportion of missing values for the covariates of interest was relatively low (Table 1): 44 subjects were missing information on age (< 0.01%), and 282 subjects were missing data on geographic location (0.02%). Sex was randomly imputed for 242 participants (< 0.1%) and assigned as 56.4% female and 43.6% male. As expected—given the lack of a differential Advisory Committee on Immunization Practices (ACIP) recommendation for ccIIV4 and egg-derived IIV4s—the completeness of covariate information was not observed to differ greatly between the 2 vaccine groups.
Characteristic . | Influenza Vaccination . | . |
---|---|---|
ccIIV4 (n = 92 187) | Egg-derived IIV4 (n = 1 261 675) | |
Age, y | ||
Missing, No. | 1 | 43 |
Mean (95% CI) | 55.6 (55.5–55.8) | 38.7 (38.6–38.7) |
Median (SD) | 59.0 (18.6) | 42 (24.4) |
Age group, No. | ||
4–17 | 7465 | 404 510 |
18–64 | 55 104 | 693 014 |
65–75 | 17 554 | 103 741 |
≥ 75 | 12 064 | 60 410 |
Female sex | 53 952 (58.5) | 709 626 (56.2) |
Geographical regiona | ||
Missing, No. | 7 | 275 |
Boston | 8181 (9) | 74 327 (6) |
New York | 659 (1) | 57 081 (5) |
Philadelphia | 9744 (11) | 167 908 (13) |
Atlanta | 53 751 (58) | 325 913 (26) |
Chicago | 7649 (8) | 251 584 (20) |
Dallas | 6302 (7) | 77 201 (6) |
Kansas City | 1637 (2) | 105 975 (8) |
Denver | 99 (< 1) | 17 476 (1) |
San Francisco | 3854 (4) | 149 088 (12) |
Seattle | 305 (< 1) | 34 856 (3) |
Race/ethnicity | ||
Missing, No. | 0 | 0 |
White | 70 283 (76.2) | 812 931 (64.4) |
Black | 7176 (7.8) | 90 351 (7.2) |
Hispanic | 4261 (4.6) | 123 077 (9.8) |
Other | 10 467 (11.4) | 235 316 (18.7) |
Comorbidity category | ||
Myocardial infarctionb | 1443 (1.6) | 9375 (0.7) |
Congestive heart failure | 1309 (1.4) | 12 334 (1) |
Peripheral vascular diseaseb | 3979 (4.3) | 26 727 (2.1) |
Cerebrovascular disease | 819 (0.9) | 5397 (0.4) |
Dementia | 2 (< 1) | 18 (< 1) |
COPDb | 12 190 (13.2) | 95 393 (7.6) |
Rheumatic disease | 1025 (1.1) | 7768 (0.6) |
Peptic ulcer disease | 4 (< 1) | 16 (< 1) |
Mild liver disease | 3642 (4.0) | 33 212 (2.6) |
Diabetes without complicationsb | 16 587 (18.0) | 142 483 (11.3) |
Diabetes with chronic complication | 4 (< 1) | 14 (< 1) |
Hemiplegia or paraplegia | 115 (0.1) | 1551 (0.1) |
Renal diseaseb | 1183 (1.3) | 4310 (0.3) |
Any malignancy, including lymphoma and leukemia, except malignant neoplasm of skin | 1968 (2.1) | 15 669 (1.2) |
Moderate or severe liver disease | 224 (0.2) | 1903 (0.2) |
Metastatic solid tumor | 0 | 0 |
HIV/AIDS | 315 (0.3) | 2983 (0.2) |
Characteristic . | Influenza Vaccination . | . |
---|---|---|
ccIIV4 (n = 92 187) | Egg-derived IIV4 (n = 1 261 675) | |
Age, y | ||
Missing, No. | 1 | 43 |
Mean (95% CI) | 55.6 (55.5–55.8) | 38.7 (38.6–38.7) |
Median (SD) | 59.0 (18.6) | 42 (24.4) |
Age group, No. | ||
4–17 | 7465 | 404 510 |
18–64 | 55 104 | 693 014 |
65–75 | 17 554 | 103 741 |
≥ 75 | 12 064 | 60 410 |
Female sex | 53 952 (58.5) | 709 626 (56.2) |
Geographical regiona | ||
Missing, No. | 7 | 275 |
Boston | 8181 (9) | 74 327 (6) |
New York | 659 (1) | 57 081 (5) |
Philadelphia | 9744 (11) | 167 908 (13) |
Atlanta | 53 751 (58) | 325 913 (26) |
Chicago | 7649 (8) | 251 584 (20) |
Dallas | 6302 (7) | 77 201 (6) |
Kansas City | 1637 (2) | 105 975 (8) |
Denver | 99 (< 1) | 17 476 (1) |
San Francisco | 3854 (4) | 149 088 (12) |
Seattle | 305 (< 1) | 34 856 (3) |
Race/ethnicity | ||
Missing, No. | 0 | 0 |
White | 70 283 (76.2) | 812 931 (64.4) |
Black | 7176 (7.8) | 90 351 (7.2) |
Hispanic | 4261 (4.6) | 123 077 (9.8) |
Other | 10 467 (11.4) | 235 316 (18.7) |
Comorbidity category | ||
Myocardial infarctionb | 1443 (1.6) | 9375 (0.7) |
Congestive heart failure | 1309 (1.4) | 12 334 (1) |
Peripheral vascular diseaseb | 3979 (4.3) | 26 727 (2.1) |
Cerebrovascular disease | 819 (0.9) | 5397 (0.4) |
Dementia | 2 (< 1) | 18 (< 1) |
COPDb | 12 190 (13.2) | 95 393 (7.6) |
Rheumatic disease | 1025 (1.1) | 7768 (0.6) |
Peptic ulcer disease | 4 (< 1) | 16 (< 1) |
Mild liver disease | 3642 (4.0) | 33 212 (2.6) |
Diabetes without complicationsb | 16 587 (18.0) | 142 483 (11.3) |
Diabetes with chronic complication | 4 (< 1) | 14 (< 1) |
Hemiplegia or paraplegia | 115 (0.1) | 1551 (0.1) |
Renal diseaseb | 1183 (1.3) | 4310 (0.3) |
Any malignancy, including lymphoma and leukemia, except malignant neoplasm of skin | 1968 (2.1) | 15 669 (1.2) |
Moderate or severe liver disease | 224 (0.2) | 1903 (0.2) |
Metastatic solid tumor | 0 | 0 |
HIV/AIDS | 315 (0.3) | 2983 (0.2) |
Data are presented as no. (%), unless otherwise indicated.
Abbreviations: ccIIV4, quadrivalent, inactivated cell-derived influenza vaccine; CI, confidence interval; COPD, chronic obstructive pulmonary disease; HIV, human immunodeficiency virus; IIV4, quadrivalent inactivated influenza vaccine; SD, standard deviation.
aInformation for geographic region (as defined by the Department of Health and Human Services) was available for all (100%) participants in the ccIIV4 group and for 99.9% (n = 1 261 206) of the egg-derived IIV4 group.
bAt least 1.5-fold difference between exposure groups.
Characteristic . | Influenza Vaccination . | . |
---|---|---|
ccIIV4 (n = 92 187) | Egg-derived IIV4 (n = 1 261 675) | |
Age, y | ||
Missing, No. | 1 | 43 |
Mean (95% CI) | 55.6 (55.5–55.8) | 38.7 (38.6–38.7) |
Median (SD) | 59.0 (18.6) | 42 (24.4) |
Age group, No. | ||
4–17 | 7465 | 404 510 |
18–64 | 55 104 | 693 014 |
65–75 | 17 554 | 103 741 |
≥ 75 | 12 064 | 60 410 |
Female sex | 53 952 (58.5) | 709 626 (56.2) |
Geographical regiona | ||
Missing, No. | 7 | 275 |
Boston | 8181 (9) | 74 327 (6) |
New York | 659 (1) | 57 081 (5) |
Philadelphia | 9744 (11) | 167 908 (13) |
Atlanta | 53 751 (58) | 325 913 (26) |
Chicago | 7649 (8) | 251 584 (20) |
Dallas | 6302 (7) | 77 201 (6) |
Kansas City | 1637 (2) | 105 975 (8) |
Denver | 99 (< 1) | 17 476 (1) |
San Francisco | 3854 (4) | 149 088 (12) |
Seattle | 305 (< 1) | 34 856 (3) |
Race/ethnicity | ||
Missing, No. | 0 | 0 |
White | 70 283 (76.2) | 812 931 (64.4) |
Black | 7176 (7.8) | 90 351 (7.2) |
Hispanic | 4261 (4.6) | 123 077 (9.8) |
Other | 10 467 (11.4) | 235 316 (18.7) |
Comorbidity category | ||
Myocardial infarctionb | 1443 (1.6) | 9375 (0.7) |
Congestive heart failure | 1309 (1.4) | 12 334 (1) |
Peripheral vascular diseaseb | 3979 (4.3) | 26 727 (2.1) |
Cerebrovascular disease | 819 (0.9) | 5397 (0.4) |
Dementia | 2 (< 1) | 18 (< 1) |
COPDb | 12 190 (13.2) | 95 393 (7.6) |
Rheumatic disease | 1025 (1.1) | 7768 (0.6) |
Peptic ulcer disease | 4 (< 1) | 16 (< 1) |
Mild liver disease | 3642 (4.0) | 33 212 (2.6) |
Diabetes without complicationsb | 16 587 (18.0) | 142 483 (11.3) |
Diabetes with chronic complication | 4 (< 1) | 14 (< 1) |
Hemiplegia or paraplegia | 115 (0.1) | 1551 (0.1) |
Renal diseaseb | 1183 (1.3) | 4310 (0.3) |
Any malignancy, including lymphoma and leukemia, except malignant neoplasm of skin | 1968 (2.1) | 15 669 (1.2) |
Moderate or severe liver disease | 224 (0.2) | 1903 (0.2) |
Metastatic solid tumor | 0 | 0 |
HIV/AIDS | 315 (0.3) | 2983 (0.2) |
Characteristic . | Influenza Vaccination . | . |
---|---|---|
ccIIV4 (n = 92 187) | Egg-derived IIV4 (n = 1 261 675) | |
Age, y | ||
Missing, No. | 1 | 43 |
Mean (95% CI) | 55.6 (55.5–55.8) | 38.7 (38.6–38.7) |
Median (SD) | 59.0 (18.6) | 42 (24.4) |
Age group, No. | ||
4–17 | 7465 | 404 510 |
18–64 | 55 104 | 693 014 |
65–75 | 17 554 | 103 741 |
≥ 75 | 12 064 | 60 410 |
Female sex | 53 952 (58.5) | 709 626 (56.2) |
Geographical regiona | ||
Missing, No. | 7 | 275 |
Boston | 8181 (9) | 74 327 (6) |
New York | 659 (1) | 57 081 (5) |
Philadelphia | 9744 (11) | 167 908 (13) |
Atlanta | 53 751 (58) | 325 913 (26) |
Chicago | 7649 (8) | 251 584 (20) |
Dallas | 6302 (7) | 77 201 (6) |
Kansas City | 1637 (2) | 105 975 (8) |
Denver | 99 (< 1) | 17 476 (1) |
San Francisco | 3854 (4) | 149 088 (12) |
Seattle | 305 (< 1) | 34 856 (3) |
Race/ethnicity | ||
Missing, No. | 0 | 0 |
White | 70 283 (76.2) | 812 931 (64.4) |
Black | 7176 (7.8) | 90 351 (7.2) |
Hispanic | 4261 (4.6) | 123 077 (9.8) |
Other | 10 467 (11.4) | 235 316 (18.7) |
Comorbidity category | ||
Myocardial infarctionb | 1443 (1.6) | 9375 (0.7) |
Congestive heart failure | 1309 (1.4) | 12 334 (1) |
Peripheral vascular diseaseb | 3979 (4.3) | 26 727 (2.1) |
Cerebrovascular disease | 819 (0.9) | 5397 (0.4) |
Dementia | 2 (< 1) | 18 (< 1) |
COPDb | 12 190 (13.2) | 95 393 (7.6) |
Rheumatic disease | 1025 (1.1) | 7768 (0.6) |
Peptic ulcer disease | 4 (< 1) | 16 (< 1) |
Mild liver disease | 3642 (4.0) | 33 212 (2.6) |
Diabetes without complicationsb | 16 587 (18.0) | 142 483 (11.3) |
Diabetes with chronic complication | 4 (< 1) | 14 (< 1) |
Hemiplegia or paraplegia | 115 (0.1) | 1551 (0.1) |
Renal diseaseb | 1183 (1.3) | 4310 (0.3) |
Any malignancy, including lymphoma and leukemia, except malignant neoplasm of skin | 1968 (2.1) | 15 669 (1.2) |
Moderate or severe liver disease | 224 (0.2) | 1903 (0.2) |
Metastatic solid tumor | 0 | 0 |
HIV/AIDS | 315 (0.3) | 2983 (0.2) |
Data are presented as no. (%), unless otherwise indicated.
Abbreviations: ccIIV4, quadrivalent, inactivated cell-derived influenza vaccine; CI, confidence interval; COPD, chronic obstructive pulmonary disease; HIV, human immunodeficiency virus; IIV4, quadrivalent inactivated influenza vaccine; SD, standard deviation.
aInformation for geographic region (as defined by the Department of Health and Human Services) was available for all (100%) participants in the ccIIV4 group and for 99.9% (n = 1 261 206) of the egg-derived IIV4 group.
bAt least 1.5-fold difference between exposure groups.
Prior to adjustment, the patients in the ccIIV4 exposure group were substantially older than the egg-derived IIV4 group (median age, 59 vs 42 years, respectively), with both groups having a similar sex distribution (Table 1). The 2 study groups were generally comparable with respect to race/ethnicity and geographic distribution with some differences observed between the 2 vaccination groups for the regions of Atlanta, Chicago, and San Francisco. With regards to comorbid status, the ccIIV4 group had at least 1.5 times the incidence of the following Charlson comorbidity categories [39, 40]: myocardial infarction, peripheral vascular disease, chronic obstructive pulmonary disease (inclusive of asthma and other chronic respiratory diseases), diabetes without complications, and renal disease.
Overall, 27 350 ILI events were observed (2.0%). (Results seen in broadly defined ILI [Code Set A] can be found in the Supplementary Data.) The observed incidence of ILI was highest in the youngest children and steadily declined as age increased to approximately 18 years of age. After 18 years of age, incidence increased steadily with each year of age until the eldest age group (85–89 years of age), which is likely an artifact of small numbers of participants in this age group.
There were 1705 (1.88%) ILI events in the ccIIV4 group, which had a median age of 59 years, compared to 25 645 (2.07%) events in the egg-derived IIV4 group, which had a median age of 42. The estimated, crude rVE of ccIIV4 vs egg-derived IIV4 was 9.2% (95% CI, 4.6%–13.6%). When adjusted for age, sex, health status, and geographic region, the estimated rVE changed to 36.2% (95% CI, 26.1%–44.9%; P < .001). Results from the PS sensitivity analysis (Supplementary Table 6) were directionally consistent with the primary analysis (Figure 1 and Supplementary Figure 1).
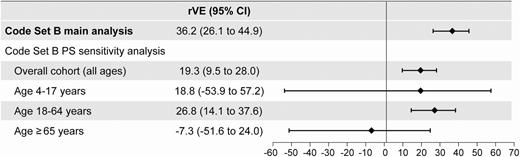
Results from the propensity score (PS)–matched and adjusted models. Main analyses are multivariable logistic regressions including age, sex, race/ethnicity, and geographic region, with age modeled as age (continuous) with interaction terms: age (continuous) × vaccine group. PS sensitivity analyses are conditional logistic regression models on matched pairs (matched by PS for exposure) with logit (PS) included only as covariate in the model (doubly robust adjustment methodology). Abbreviations: CI, confidence interval; PS, propensity score; rVE, relative vaccine effectiveness.
DISCUSSION
In this analysis of 1 353 862 vaccinations in persons ≥ 4 years of age, ccIIV4 was significantly more effective in preventing ILI captured within primary care visits in the 2017–2018 influenza season in the US. For the narrowly defined ILI, the PS-matched conditional logistic regression model confirmed findings from the main analysis. This finding is noteworthy given the context: the 2017–2018 influenza season in the US was predominated by circulating A(H3N2) influenza viruses. These results are directionally similar and consistent with other published findings [29–32]. The production of vaccines using cell-propagated influenza viruses eliminates opportunities for viral mutations to occur and maintains viral antigenicity, which supports the improved effectiveness of ccIV4 observed in this study [19]. However, VE depends on multiple factors, including those at the individual level (such as repeat vaccination, immune system immaturity, and immunosenescence), vaccine level (such as vaccine-strain virus propagation in eggs and alternative production platforms), and virus level (natural antigenic drift and shift) [21].
The majority of vaccinations in the dataset were egg-derived IIV4 (93%), which is not unexpected as ccIIV4 was approved in the US market in May 2016 and is not yet in widespread clinical use. The observed differences in baseline covariates between exposure groups—particularly in terms of age, health status, and geographic region—appear to be a statistical artifact present in the dataset as the ACIP did not preferentially indicate ccIIV4 for older or sicker patients in the 2017–2018 season [41] and, therefore, it is not expected that ccIIV4 be systematically administered to a specific patient profile as part of routine clinical practice. Subjects with a record of ccIIV4 were older and had more comorbid conditions than the egg-derived IIV4 group, which may have resulted in differences between crude and adjusted estimates. This covariate imbalance was addressed by adjusting the logistic regression models in both the main analysis and in the sensitivity analysis. From the PS analyses, it appears as though the overall effectiveness estimate is driven by the statistically significant rVE estimated in adults 18–64 years of age. The small number of cases in the pediatric (4–17 years) and older adult (≥65 years) cohorts resulted in point estimates with large standard errors and wide CIs—crossing the null for narrowly defined ILI—precluding any definitive conclusions about the relative effectiveness of ccIIV4 in these age groups. VE is typically lower in older adults compared to younger populations due to a phenomenon known as immunosenescence—the gradual and natural deterioration of the immune system due to aging [42]. Enhanced influenza vaccines (such as the adjuvanted or high-dose trivalent vaccines) are specifically indicated for adults ≥ 65 years of age to address suboptimal flu vaccine responses in older adults.
The difference in effect estimates between the primary analysis and the matched PS sensitivity analysis (36.2% vs 19.3% for narrowly defined ILI) may be attributed to 2 key differences between the analyses. First, the multivariable logit model used in the primary analysis was compiled using model-building methods based on statistical significance. As such, not all categories of the 5 variables were included in the final model, whereas all of the categories of all variables were forced into the PS model. Second, the study populations differed between the 2 analyses: all ccIIV4 and egg-derived IIV4 recipients were included in the main analysis, while only matched pairs were included in the PS analysis. Despite these differences, both the primary analysis and the more conservative matched PS approach demonstrated significantly better effectiveness of ccIIV4 vs egg-derived IIV4.
A key strength of the study is the large sample size available to conduct robust statistical analyses. Furthermore, exposure ascertainment was derived from patient EMRs using specific product codes, limiting exposure misclassification and minimizing biases often observed when comparing a vaccinated to unvaccinated cohort. Nonetheless, results from this study must be interpreted in light of several limitations. First, the effectiveness outcome was not PCR-confirmed influenza, the gold standard for influenza VE studies. However, as previously above, the diagnostic ICD code set used to define ILI was validated by the AFHSC in a study population primarily composed of active military personnel dependents, supporting the generalizability of the validation study results to the nonmilitary individuals [43]. Additionally, a descriptive evaluation of the overlap between CDC-reported laboratory-confirmed influenza and the incidence of ILI (defined both broadly and narrowly) was conducted within this specific study cohort. Concordance was observed between the AFHSC diagnostic code sets for influenza and the incidence of laboratory-confirmed influenza (Supplementary Table 7), supporting the use of these code sets in real-world evidence—particularly for ILI defined narrowly, which was defined a priori as the primary outcome for this study. Another study limitation is the inability to reliably estimate absolute influenza VE from the ambulatory EMR dataset. This is because a patient may not have a record of an influenza vaccination record in their primary care EMR but have obtained the vaccine in another setting (such as a workplace or pharmacy). Importantly, this issue of exposure status misclassification (ie, false-negative vaccine status) does not apply when comparing 2 vaccinated cohorts, as was done in this analysis. Last, the main analysis did not specifically adjust for functional status (a variable identified as a potential confounder of influenza VE in older adults) and receipt of an influenza vaccine in the previous season, given that this information was not readily, reliably, and/or systematically available from all subject EMRs [44]. The analysis also did not specifically adjust for timing of influenza vaccinations throughout the course of the season as no systematic differences were observed in the timing of vaccine administration (by month) between both vaccine groups (Supplementary Figure 2).
To conclude, the rVE estimates for ccIIV4 vs egg-derived IIV4 in preventing ILI were derived from EMR data evaluated as a retrospective cohort study. Results from this study demonstrate that ccIIV4 vaccine produced using an A(H3N2) seed virus propagated exclusively in cell culture, and thus lacking egg adaptive changes, was significantly more effective than conventional IIVs in preventing ILI as captured within US primary care visits in the 2017–2018 influenza season. This study contributes to the body of evidence supporting the theoretical basis for better relative effectiveness of the cell-derived influenza vaccine compared to standard, egg-derived vaccines. We expect these results to be generalizable to the US population ≥ 4 years of age.
Supplementary Data
Supplementary materials are available at Clinical Infectious Diseases online. Consisting of data provided by the authors to benefit the reader, the posted materials are not copyedited and are the sole responsibility of the authors, so questions or comments should be addressed to the corresponding author.
Notes
Acknowledgments. C. Gordon Beck and Amanda M. Justice provided editorial support (formatting, reference management) in the preparation of this manuscript, which was funded by Seqirus Inc.
Financial support. This work was supported by Seqirus Inc.
Potential conflicts of interest. C. B. and J. A. M. are employees of Seqirus Inc. G. C. S. is an employee of Seqirus USA Inc. J. S. S. was contracted by Seqirus and received fees for data management and statistical analyses. All authors have submitted the ICMJE Form for Disclosure of Potential Conflicts of Interest. Conflicts that the editors consider relevant to the content of the manuscript have been disclosed.