-
PDF
- Split View
-
Views
-
Cite
Cite
Jonathan L Golob, Steven A Pergam, Sujatha Srinivasan, Tina L Fiedler, Congzhou Liu, Kristina Garcia, Marco Mielcarek, Daisy Ko, Sarah Aker, Sara Marquis, Tillie Loeffelholz, Anna Plantinga, Michael C Wu, Kevin Celustka, Alex Morrison, Maresa Woodfield, David N Fredricks, Stool Microbiota at Neutrophil Recovery Is Predictive for Severe Acute Graft vs Host Disease After Hematopoietic Cell Transplantation, Clinical Infectious Diseases, Volume 65, Issue 12, 15 December 2017, Pages 1984–1991, https://doi.org/10.1093/cid/cix699
- Share Icon Share
Abstract
Graft-versus-host disease (GVHD) is common after allogeneic hematopoietic cell transplantation (HCT). Risk for death from GVHD has been associated with low bacterial diversity in the stool microbiota early after transplant; however, the specific species associated with GVHD risk remain poorly defined.
We prospectively collected serial weekly stool samples from 66 patients who underwent HCT, starting pre-transplantation and continuing weekly until 100 days post-transplant, a total of 694 observations in HCT recipients. We used 16S rRNA gene polymerase chain reaction with degenerate primers, followed by high-throughput sequencing to assess the relative abundance of sequence reads from bacterial taxa in stool samples over time.
The gut microbiota was highly dynamic in HCT recipients, with loss and appearance of taxa common on short time scales. As in prior studies, GVHD was associated with lower alpha diversity of the stool microbiota. At neutrophil recovery post-HCT, the presence of oral Actinobacteria and oral Firmicutes in stool was positively correlated with subsequent GVHD; Lachnospiraceae were negatively correlated. A gradient of bacterial species (difference of the sum of the relative abundance of positive correlates minus the sum of the relative abundance of negative correlates) was most predictive (receiver operator characteristic area under the curve of 0.83) of subsequent severe acute GVHD.
The stool microbiota around the time of neutrophil recovery post-HCT is predictive of subsequent development of severe acute GVHD in this study.
Acute graft-versus-host disease (GVHD) is a major cause of morbidity and mortality in patients who undergo hematopoietic cell transplantation (HCT). GVHD can present with enteritis, dermatitis, and hepatitis following transplant [1]. An estimated 30%–80% of HCT recipients suffer from GVHD despite matching for HLA and use of prophylactic immunosuppression (eg, cyclosporine) [2, 3]. First-line treatment for GVHD is glucocorticoid steroids, which are associated with an increased risk for cytomegalovirus disease and fungal infections [4, 5].
Death from GVHD in HCT has been previously associated with low bacterial species diversity [6] and the lack of Blautia luti [7] in the stool microbiota of human patients. These data have suggested associations between increased bacterial diversity at the time of engraftment with improved survival [6] but do not focus on development of GVHD as the endpoint. The use of antianaerobe antibiotics (piperacillin–tazobactam and imipenem–cilastatin) was associated with higher rates of GVHD and overgrowth of Akkermansia species in a murine model of HCT [8]. Together these data indicate a need for more detailed assessment of the relationship between specific members of the gut microbiota and GVHD, including assessments at different transplant centers where antibiotic use patterns differ.
Here, we prospectively collected weekly stool samples from patients who underwent HCT, starting before transplant and continuing for 100 days post-transplant. We show an association between lower bacterial species diversity and severe acute GVHD, with a modest predictive strength when tested at recovery of neutrophils post-transplant. We also identify several specific organisms whose relative abundance at neutrophil recovery appears to predict severe acute GVHD of the gut.
METHODS
Microbiome Sample Collection
Weekly kits that contained polyurethane foam swabs (Epicenter Biotechnologies, Madison, Wisconsin) were provided to participants to self-collect stool samples at home; nurses collected swabs from inpatients. Swabs were returned to our laboratory by courier or regular mail at room temperature without special preservative. Upon arrival, samples were tagged and stored at −80oC until retrieved for DNA extraction. The collection date and arrival date were noted; if it was longer than 72 hours between sample collection and storage, the samples were discarded. Several studies have demonstrated that the stool microbiota is not greatly impacted by these storage conditions [9–13]. Related donors who consented to the study collected 1 stool sample.
Clinical Metadata
All clinical data including GVHD grade [1, 14] onset date, and neutrophil recovery were determined by retrospective review of the clinical charts by individuals blinded to the microbiome results of the participants. Severe GVHD was defined as grade ≥3. All patients had stage IIb to IV gut GVHD to be called severe acute GVHD in our study. The acute GVHD start date is defined as the onset of any symptoms, while the stage is determined by the worst conditions observed in the patient, typically after the onset of symptoms. See the Supplementary Appendix for further details.
Neutrophil recovery was defined as the third day of an absolute neutrophil count >500 neutrophils/mm3. We selected neutrophil recovery as our primary time point because it is present in almost all HCT recipients at or before the onset of GVHD and at a point at which one could consider microbial interventions such as fecal microbiota transplant with less concern for infectious complications.
Patients received prophylactic antibiotics including levofloxacin when their absolute neutrophil count was below 500 cells/mm3. For comprehensive details of the antibiotic protocols, see the supplement Antibiotic Protocols in Hematopoietic Cell Transplant at the Fred Hutch.
DNA Extraction and Quality Control
DNA was extracted using the BiOstic Bacteremia DNA Isolation Kit (MoBio, Carlsbad, California) and eluted into 150 μL of 0.5 mM Tris–0.05 mM EDTA (TE) buffer. Sham swabs were extracted in the same manner to monitor for contamination of the extraction materials. All extracts were assessed for the presence of polymerase chain reaction (PCR) inhibitors via spike-in of jellyfish gDNA [15]. Bacterial DNA concentrations were measured using a quantitative PCR assay that target the V3–V4 region of the 16S rRNA gene [16].
Broad-Range Polymerase Chain Reaction and High-Throughput Sequencing of 16S rRNA Gene Amplicons
Broad-range PCR targeting of the V3–V4 hypervariable region of the 16S rRNA gene was performed (see Supplementary Materials for primer details and PCR conditions). Amplicons were cleaned using Agencourt AMPure XP beads (Beckman Coulter, Indianapolis, Indiana) per the 16S Metagenomic Sequencing Library Preparation [17]. Cleaned amplicons were subjected to Index PCR using NexteraXT index kits v2 set A, set B, set C, and set D to multiplex 384 samples per sequencing run. Following index PCR, the DNA was cleaned using Agencourt AMPure XP beads (Beckman Coulter) per the 16S Metagenomic Sequencing Library Preparation [17], air dried for 8 minutes, and eluted in 30 µL 1× TE buffer. DNA concentration of each sample was determined using the Quant-iT dsDNA assay kit-high sensitivity (Thermo Fisher Scientific, Waltham, Massachusetts), and equimolar quantities of samples were pooled.
Sequencing was performed on the Illumina MiSeq instrument (Illumina, San Diego, California) with the MiSeq reagent kit v3-600 cycle to capture paired-end reads (2 × 300). PhiX Control Library v3 (Illumina) was combined with the amplicon library at 15%. The combined library was denatured with sodium hydroxide, diluted with hybridization buffer, and heat denatured. The library was sequenced as PE300 reads on the MiSeq using standard Illumina primers. Raw sequence reads were demultiplexed using Illumina’s bcl2fastq conversion software, v1.8.4, with zero mismatches to forward and reverse indices [18].
Bacterial Species Classification Reference Set Creation
DADA2 [19] was used to quality control (QC) filter, pair, and cluster the amplicon reads. The filtered and paired 16S rRNA gene amplicons were used to recruit full-length 16S rRNA gene sequences from Ribosomal Database Project release 11.4 [20]. The collected reference sequences were assembled into a phylogenetic tree using FastTree; leaves with only 1 representative sequence were used to recruit additional sequences.
Classification of Sequences into Taxonomic Profiles
QC-filtered amplicon sequences were placed onto the classification reference tree using the pplacer tool, as previously described [21], and validated in a separate study from our group [22].
Statistical Analyses
The PANDAS [23] package (version 0.19.2) of Python was used for data organization and normalization. The Python statsmodels package [24] (version 0.8.0) was used for all statistical analyses. The correlation between alpha diversity and severe acute GVHD was as follows: when all time points considered for an individual were used, generalized estimating equations (GEEs) using an independence working correlation and a binomial family with a logit link function were used. When 1 measurement was used per individual (ie, the sample closest to neutrophil recovery), logistic regression was used. For the correlation between organisms and severe (grade 3–4) acute GVHD: similar to analysis of the composition of microbiomes (ANCOM) [25], for each observed organism identified to the genus or species level and each genus identified, a log ratio of the species count plus 1 over the genus count plus 1 was calculated. Logistic regression was performed, with these ratios as the independent variable and severe acute GVHD as the dependent variable. The estimated coefficient (β) was normalized to its standard error of the mean to generate the Wald statistic. The distribution of the Wald statistic for each species was considered, with the Student T distribution used to determine a P value, which underwent the Bonferroni correction.
RESULTS
Participant Characteristics
Participants had a wide variety of indications for transplant, as well as transplant protocols (Table 1). For each participant an average of 11 weekly samples were assessed, spanning from before transplant through 100 days post-transplant, for a total of 694 stool microbiota profiles. Additionally, 36 HCT donors’ stool microbiota was assessed.
. | Acute GVHD . | Total . | |||
---|---|---|---|---|---|
None . | Grade 1–2 . | Grade 3–4 . | |||
Number | 14 | 38 | 14 | 66 | |
Age range, y (mean) | 38–71 (62) | 30–69 (50) | 22–73 (54) | ||
Disease | Acute lymphoblastic leukemia (%) | 0 (0.0) | 3 (7.9) | 1 (7.1) | 4 (6.1) |
Acute myeloid leukemia (%) | 3 (21.4) | 10 (26.3) | 4 (28.6) | 17 (25.8) | |
Chronic lymphoblastic leukemia (%) | 1 (7.1) | 2 (5.3) | 1 (7.1) | 4 (6.0) | |
Chronic myeloid leukemia (%) | 0 (0.0) | 1 (2.6) | 0 (0.0) | 1 (1.5) | |
Hodgkin’s disease (%) | 0 (0.0) | 0 (0.0) | 2 (14.3) | 2 (3.0) | |
Hemophagocytic lymphohistiocytosis (%) | 0 (0.0) | 1 (2.6) | 0 (0.0) | 1 (1.5) | |
Myelodysplastic syndrome (%) | 6 (42.9) | 15 (39.5) | 3 (21.4) | 24 (36.4) | |
Multiple myeloma (%) | 1 (7.1) | 1 (2.6) | 0 (0.0) | 2 (3.0) | |
Non-Hodgkin’s lymphoma (%) | 3 (21.4) | 4 (10.5) | 3 (21.4) | 10 (15.2) | |
Primary nasopharyngeal lymphoma (%) | 0 (0.0) | 1 (2.6) | 0 (0.0) | 1 (1.5) | |
Transplanta | Related (%) | 7 (50.0) | 25 (65.8) | 8 (57.1) | 40 (60.6) |
Mini (%) | 9 (64.3) | 6 (15.8) | 6 (42.9) | 21 (31.8) | |
Matched (%) | 13 (92.9) | 35 (92.1) | 12 (85.7) | 60 (90.9) | |
GVHD diagnosis day post transplant (mean) | 8–85 (39) | 16–61 (32.4) |
. | Acute GVHD . | Total . | |||
---|---|---|---|---|---|
None . | Grade 1–2 . | Grade 3–4 . | |||
Number | 14 | 38 | 14 | 66 | |
Age range, y (mean) | 38–71 (62) | 30–69 (50) | 22–73 (54) | ||
Disease | Acute lymphoblastic leukemia (%) | 0 (0.0) | 3 (7.9) | 1 (7.1) | 4 (6.1) |
Acute myeloid leukemia (%) | 3 (21.4) | 10 (26.3) | 4 (28.6) | 17 (25.8) | |
Chronic lymphoblastic leukemia (%) | 1 (7.1) | 2 (5.3) | 1 (7.1) | 4 (6.0) | |
Chronic myeloid leukemia (%) | 0 (0.0) | 1 (2.6) | 0 (0.0) | 1 (1.5) | |
Hodgkin’s disease (%) | 0 (0.0) | 0 (0.0) | 2 (14.3) | 2 (3.0) | |
Hemophagocytic lymphohistiocytosis (%) | 0 (0.0) | 1 (2.6) | 0 (0.0) | 1 (1.5) | |
Myelodysplastic syndrome (%) | 6 (42.9) | 15 (39.5) | 3 (21.4) | 24 (36.4) | |
Multiple myeloma (%) | 1 (7.1) | 1 (2.6) | 0 (0.0) | 2 (3.0) | |
Non-Hodgkin’s lymphoma (%) | 3 (21.4) | 4 (10.5) | 3 (21.4) | 10 (15.2) | |
Primary nasopharyngeal lymphoma (%) | 0 (0.0) | 1 (2.6) | 0 (0.0) | 1 (1.5) | |
Transplanta | Related (%) | 7 (50.0) | 25 (65.8) | 8 (57.1) | 40 (60.6) |
Mini (%) | 9 (64.3) | 6 (15.8) | 6 (42.9) | 21 (31.8) | |
Matched (%) | 13 (92.9) | 35 (92.1) | 12 (85.7) | 60 (90.9) | |
GVHD diagnosis day post transplant (mean) | 8–85 (39) | 16–61 (32.4) |
Abbreviation: GVHD, graft-versus-host disease.
aMini: nonmyeloablative conditioning.
. | Acute GVHD . | Total . | |||
---|---|---|---|---|---|
None . | Grade 1–2 . | Grade 3–4 . | |||
Number | 14 | 38 | 14 | 66 | |
Age range, y (mean) | 38–71 (62) | 30–69 (50) | 22–73 (54) | ||
Disease | Acute lymphoblastic leukemia (%) | 0 (0.0) | 3 (7.9) | 1 (7.1) | 4 (6.1) |
Acute myeloid leukemia (%) | 3 (21.4) | 10 (26.3) | 4 (28.6) | 17 (25.8) | |
Chronic lymphoblastic leukemia (%) | 1 (7.1) | 2 (5.3) | 1 (7.1) | 4 (6.0) | |
Chronic myeloid leukemia (%) | 0 (0.0) | 1 (2.6) | 0 (0.0) | 1 (1.5) | |
Hodgkin’s disease (%) | 0 (0.0) | 0 (0.0) | 2 (14.3) | 2 (3.0) | |
Hemophagocytic lymphohistiocytosis (%) | 0 (0.0) | 1 (2.6) | 0 (0.0) | 1 (1.5) | |
Myelodysplastic syndrome (%) | 6 (42.9) | 15 (39.5) | 3 (21.4) | 24 (36.4) | |
Multiple myeloma (%) | 1 (7.1) | 1 (2.6) | 0 (0.0) | 2 (3.0) | |
Non-Hodgkin’s lymphoma (%) | 3 (21.4) | 4 (10.5) | 3 (21.4) | 10 (15.2) | |
Primary nasopharyngeal lymphoma (%) | 0 (0.0) | 1 (2.6) | 0 (0.0) | 1 (1.5) | |
Transplanta | Related (%) | 7 (50.0) | 25 (65.8) | 8 (57.1) | 40 (60.6) |
Mini (%) | 9 (64.3) | 6 (15.8) | 6 (42.9) | 21 (31.8) | |
Matched (%) | 13 (92.9) | 35 (92.1) | 12 (85.7) | 60 (90.9) | |
GVHD diagnosis day post transplant (mean) | 8–85 (39) | 16–61 (32.4) |
. | Acute GVHD . | Total . | |||
---|---|---|---|---|---|
None . | Grade 1–2 . | Grade 3–4 . | |||
Number | 14 | 38 | 14 | 66 | |
Age range, y (mean) | 38–71 (62) | 30–69 (50) | 22–73 (54) | ||
Disease | Acute lymphoblastic leukemia (%) | 0 (0.0) | 3 (7.9) | 1 (7.1) | 4 (6.1) |
Acute myeloid leukemia (%) | 3 (21.4) | 10 (26.3) | 4 (28.6) | 17 (25.8) | |
Chronic lymphoblastic leukemia (%) | 1 (7.1) | 2 (5.3) | 1 (7.1) | 4 (6.0) | |
Chronic myeloid leukemia (%) | 0 (0.0) | 1 (2.6) | 0 (0.0) | 1 (1.5) | |
Hodgkin’s disease (%) | 0 (0.0) | 0 (0.0) | 2 (14.3) | 2 (3.0) | |
Hemophagocytic lymphohistiocytosis (%) | 0 (0.0) | 1 (2.6) | 0 (0.0) | 1 (1.5) | |
Myelodysplastic syndrome (%) | 6 (42.9) | 15 (39.5) | 3 (21.4) | 24 (36.4) | |
Multiple myeloma (%) | 1 (7.1) | 1 (2.6) | 0 (0.0) | 2 (3.0) | |
Non-Hodgkin’s lymphoma (%) | 3 (21.4) | 4 (10.5) | 3 (21.4) | 10 (15.2) | |
Primary nasopharyngeal lymphoma (%) | 0 (0.0) | 1 (2.6) | 0 (0.0) | 1 (1.5) | |
Transplanta | Related (%) | 7 (50.0) | 25 (65.8) | 8 (57.1) | 40 (60.6) |
Mini (%) | 9 (64.3) | 6 (15.8) | 6 (42.9) | 21 (31.8) | |
Matched (%) | 13 (92.9) | 35 (92.1) | 12 (85.7) | 60 (90.9) | |
GVHD diagnosis day post transplant (mean) | 8–85 (39) | 16–61 (32.4) |
Abbreviation: GVHD, graft-versus-host disease.
aMini: nonmyeloablative conditioning.
Stool Microbiota of Healthy Donors
Healthy human stool microbiota varies from individual to individual, with only about one third of bacterial species being common between any 2 individuals. Members of the Bacteroidaceae and Lachnospiracae families are dominant in most individuals (Figure 1A).

The stool microbiome of hematopoietic cell transplantation (HCT) donors and HCT recipients over time. The height of each colored bar or strip is proportionate to the relative abundance of 1 specific organism identified to the species or genus level. The legend is simplified to the family level for legibility. The coloration is related to the phylum of the organism: Firmicutes, purple/blue (Lachnospiraceae in purple); Proteobacteria, tan; Bacteroides, orange; and Actinobacteria, teal. A. The stool microbiome of 36 healthy HCT donors, demonstrating a typical dominance of Lachnospiracea or Bacteroides, with occasional codominance with Enterobacteraceae. B–E, HCT recipients’ microbiota over time. The x-axis is days relative to transplant. The vertical dashed line depicts the date of neutrophil recovery. For those with severe acute graft-versus-host disease (GVHD) (D and E), the date of GVHD onset is depicted with a black dot on the timeline. C, A patient who underwent a partial colectomy prior to transplant. E, Three HCT recipients who developed both severe and steroid-refractory acute GVHD. The HCT recipients with the most samples were included in this figure; those with fewer samples were omitted in consideration of space. Abbreviations: GVHD, graft-versus-host disease; HCT, hematopoietic cell transplantation.
Stool Microbiota in Hematopoietic Cell Transplantation Transplant Patients Over Time
In general, individuals without severe acute GVHD of the gut maintained a stool microbiota broadly similar to those we observed in healthy donors (Figure 1B); there was dominance with a combination of Bacteroidaceae species and/or members of the Lachnospiraceae. One exception was a patient who underwent partial colectomy for diverticular disease prior to transplant (Figure 1C). Some of those with severe acute GVHD (Figure 1D) had a deficit of Lachnospiraceae. Two of the 3 individuals with steroid-refractory severe acute GVHD (Figure 1E) had a persistent lack of Bacteroidaceae and Lachnospiracae species after the onset of GVHD. We did not see a consistent trend in the rate of change over time (Supplementary Figure S1) with grade of GVHD. For recipients for whom we had paired stool microbiota samples from their donors, we saw a trend toward higher donor-to-recipient microbiota distance (an indicator of dissimilarity of the bacterial communities) as grade of acute GVHD increased, through grade 3 (Supplementary Figure S2); the one grade 4 patient for whom we had a donor pair was an outlier for this trend. The difference between grade 3 acute GVHD and no acute GVHD was significant (P < 0.05) as per GEE regression.
Diversity of the Stool Microbiota of Hematopoietic Cell Transplantation Recipients and Donors
Figure 2A shows the alpha diversity of HCT donors and HCT recipients stratified by acute GVHD grade. We used the following 2 measures of diversity: balanced weighted phylogenetic diversity (BWPD) [26], which is a measure that accounts for the phylogenetic relationships between organisms in the community when calculating diversity, and Inverse Shannon [27] (InvSh), which is a commonly used measure of species diversity. When using all samples for a given patient, or just the sample closest to neutrophil recovery, there was a trend to lower alpha diversity (InvSh or BWPD) at higher grades of acute GVHD. By GEE regression with an independence correlation structure, both BWPD and InvSh alpha diversity were statistically significantly lower in patients with grade 3 or 4 GVHD when compared to those with no acute GVHD or grade 1–2 acute GVHD (P < 0.05). Figure 2B shows the receiver operator characteristic (ROC) curve for BWPD (area under the curve [AUC] of 0.56) and InvSh diversity (AUC of 0.63) at neutrophil recovery as a predictor for eventual development of severe acute GVHD (grade 3–4).
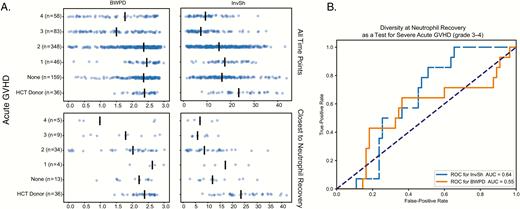
Alpha diversity and graft-versus-host disease (GVHD). A, Balanced weighted phylogenetic diversity (BWPD) and inverse Shannon (InvSh) species diversity. The top row is for all observed time points; the bottom row is only for samples closest to neutrophil recovery. Alpha diversity was statistically significantly lower in those with severe GVHD (P < 0.05) as calculated by general estimating equations with independence as a correlation structure when all time points are considered. B, A receiver operator characteristic for BWPD (dashed blue) and InvSh (solid orange) at neutrophil engraftment as a test for subsequent development of severe (grade 3–4) acute GVHD. Abbreviations: AUC, area under the curve; BWPD, balanced weighted phylogenetic diversity; GVHD, graft-versus-host disease; HCT, hematopoietic cell transplantation; InvSh, inverse Shannon; ROC, receiver operator characteristic.
Specific Bacteria and Development of Severe Acute Graft-versus-Host Disease
To test for correlations between specific bacteria (identified to species or genus level) at neutrophil recovery and the subsequent development of severe acute GVHD, we used an ANCOM-like analysis in which we calculated the log of the ratio of counts of each species or genus to each other genus and then performed logistic regression for each ratio to the endpoint (acute severe GVHD or not) [25]. We then selected the organisms that were consistently significantly positively or negatively correlated with subsequent severe acute GVHD (Table 2). Members of the Bacteroides genus (B. thetaiotaomicron, B. ovatus, and B. caccae) were negatively correlated with subsequent acute severe GVHD, while others (B. dorei) were positively correlated. Among the gram-positive bacteria, oral bacteria such as Rothia mucilaginosa, Solobacterium moorei, and Veillonella parvula were positively correlated; several Lachnospiraceae (including B. luti) and a Butyricicoccus species were negatively correlated.
Organisms at Neutrophil Recovery Negatively or Positively Correlated With Subsequent Development of Severe Acute Graft-Versus-Host Disease
Organism . | Correlation With Severe Acute Graft-Versus-Host Diseasea . | ||
---|---|---|---|
β/SEM(β) . | P (corr) . | Lineage . | |
Erysipelatoclostridium ramosum | –1.7 | 2.92E-84 | Firmicutes; Erysipelotrichia; Erysipelotrichales; Erysipelotrichaceae; Erysipelatoclostridium |
Bacteroides thetaiotaomicron | –1.5 | 3.68E-82 | Bacteroidetes/Chlorobi group; Bacteroidetes; Bacteroidia; Bacteroidales; Bacteroidaceae; Bacteroides |
Blautia luti | –1.4 | 2.92E-83 | Firmicutes; Clostridia; Clostridiales; Lachnospiraceae; Blautia |
Candidatus soleaferrea | –1.4 | 9.82E-66 | Firmicutes; Clostridia; Clostridiales; Ruminococcaceae |
Butyricicoccus spp. | –1.4 | 1.35E-64 | Firmicutes; Clostridia; Clostridiales; Clostridiaceae |
Blautia spp. | –1.3 | 7.26E-76 | Firmicutes; Clostridia; Clostridiales; Lachnospiraceae; Blautia |
Dorea spp. | –1.2 | 2.29E-64 | Firmicutes; Clostridia; Clostridiales; Lachnospiraceae |
Ruminococcus spp. | –1.2 | 1.08E-44 | Firmicutes; Clostridia; Clostridiales; Ruminococcaceae |
Clostridium clostridioforme | –1.2 | 2.17E-54 | Firmicutes; Clostridia; Clostridiales; Lachnospiraceae; Fusicatenibacter |
Bacteroides ovatus | –1.2 | 8.73E-69 | Bacteroidetes/Chlorobi group; Bacteroidetes; Bacteroidia; Bacteroidales; Bacteroidaceae; Bacteroides |
Bacteroides caccae | –1.0 | 3.33E-51 | Bacteroidetes/Chlorobi group; Bacteroidetes; Bacteroidia; Bacteroidales; Bacteroidaceae; Bacteroides |
Roseburia hominis | 1.0 | 1.82E-39 | Firmicutes; Clostridia; Clostridiales; Lachnospiraceae; Roseburia |
Eisenbergiella spp. | 1.0 | 3.96E-45 | Firmicutes; Clostridia; Clostridiales; Lachnospiraceae |
Veillonella parvula | 1.0 | 2.32E-38 | Firmicutes; Negativicutes; Selenomonadales; Veillonellaceae; Veillonella |
Enterococcus faecium or hirae–like | 1.1 | 2.56E-61 | Firmicutes; Bacilli; Lactobacillales; Enterococcaceae; Enterococcus |
Lactobacillus salivarius | 1.1 | 3.94E-48 | Firmicutes; Bacilli; Lactobacillales; Lactobacillaceae; Lactobacillus |
Lactobacillus fermentum | 1.2 | 1.02E-66 | Firmicutes; Bacilli; Lactobacillales; Lactobacillaceae; Lactobacillus |
Bacteroides dorei | 1.3 | 1.59E-82 | Bacteroidetes/Chlorobi group; Bacteroidetes; Bacteroidia; Bacteroidales; Bacteroidaceae; Bacteroides |
Solobacterium moorei | 1.3 | 1.93E-40 | Firmicutes; Erysipelotrichia; Erysipelotrichales; Erysipelotrichaceae; Solobacterium |
Rothia mucilaginosa | 1.4 | 1.05E-57 | Actinobacteria; Actinobacteria; Actinobacteridae; Actinomycetales; Micrococcineae; Micrococcaceae; Rothia |
Organism . | Correlation With Severe Acute Graft-Versus-Host Diseasea . | ||
---|---|---|---|
β/SEM(β) . | P (corr) . | Lineage . | |
Erysipelatoclostridium ramosum | –1.7 | 2.92E-84 | Firmicutes; Erysipelotrichia; Erysipelotrichales; Erysipelotrichaceae; Erysipelatoclostridium |
Bacteroides thetaiotaomicron | –1.5 | 3.68E-82 | Bacteroidetes/Chlorobi group; Bacteroidetes; Bacteroidia; Bacteroidales; Bacteroidaceae; Bacteroides |
Blautia luti | –1.4 | 2.92E-83 | Firmicutes; Clostridia; Clostridiales; Lachnospiraceae; Blautia |
Candidatus soleaferrea | –1.4 | 9.82E-66 | Firmicutes; Clostridia; Clostridiales; Ruminococcaceae |
Butyricicoccus spp. | –1.4 | 1.35E-64 | Firmicutes; Clostridia; Clostridiales; Clostridiaceae |
Blautia spp. | –1.3 | 7.26E-76 | Firmicutes; Clostridia; Clostridiales; Lachnospiraceae; Blautia |
Dorea spp. | –1.2 | 2.29E-64 | Firmicutes; Clostridia; Clostridiales; Lachnospiraceae |
Ruminococcus spp. | –1.2 | 1.08E-44 | Firmicutes; Clostridia; Clostridiales; Ruminococcaceae |
Clostridium clostridioforme | –1.2 | 2.17E-54 | Firmicutes; Clostridia; Clostridiales; Lachnospiraceae; Fusicatenibacter |
Bacteroides ovatus | –1.2 | 8.73E-69 | Bacteroidetes/Chlorobi group; Bacteroidetes; Bacteroidia; Bacteroidales; Bacteroidaceae; Bacteroides |
Bacteroides caccae | –1.0 | 3.33E-51 | Bacteroidetes/Chlorobi group; Bacteroidetes; Bacteroidia; Bacteroidales; Bacteroidaceae; Bacteroides |
Roseburia hominis | 1.0 | 1.82E-39 | Firmicutes; Clostridia; Clostridiales; Lachnospiraceae; Roseburia |
Eisenbergiella spp. | 1.0 | 3.96E-45 | Firmicutes; Clostridia; Clostridiales; Lachnospiraceae |
Veillonella parvula | 1.0 | 2.32E-38 | Firmicutes; Negativicutes; Selenomonadales; Veillonellaceae; Veillonella |
Enterococcus faecium or hirae–like | 1.1 | 2.56E-61 | Firmicutes; Bacilli; Lactobacillales; Enterococcaceae; Enterococcus |
Lactobacillus salivarius | 1.1 | 3.94E-48 | Firmicutes; Bacilli; Lactobacillales; Lactobacillaceae; Lactobacillus |
Lactobacillus fermentum | 1.2 | 1.02E-66 | Firmicutes; Bacilli; Lactobacillales; Lactobacillaceae; Lactobacillus |
Bacteroides dorei | 1.3 | 1.59E-82 | Bacteroidetes/Chlorobi group; Bacteroidetes; Bacteroidia; Bacteroidales; Bacteroidaceae; Bacteroides |
Solobacterium moorei | 1.3 | 1.93E-40 | Firmicutes; Erysipelotrichia; Erysipelotrichales; Erysipelotrichaceae; Solobacterium |
Rothia mucilaginosa | 1.4 | 1.05E-57 | Actinobacteria; Actinobacteria; Actinobacteridae; Actinomycetales; Micrococcineae; Micrococcaceae; Rothia |
Severe graft-versus-host disease is grade 3–4.
aAn ANCOM (analysis of composition of microbiomes)–style [25] analysis was performed. β/SEM(β) is the Wald statistic. The Bonferroni correction was used to correct P. Neutrophil recovery is the third consecutive day of an absolute neutrophil count >500 cells per mm3.
Organisms at Neutrophil Recovery Negatively or Positively Correlated With Subsequent Development of Severe Acute Graft-Versus-Host Disease
Organism . | Correlation With Severe Acute Graft-Versus-Host Diseasea . | ||
---|---|---|---|
β/SEM(β) . | P (corr) . | Lineage . | |
Erysipelatoclostridium ramosum | –1.7 | 2.92E-84 | Firmicutes; Erysipelotrichia; Erysipelotrichales; Erysipelotrichaceae; Erysipelatoclostridium |
Bacteroides thetaiotaomicron | –1.5 | 3.68E-82 | Bacteroidetes/Chlorobi group; Bacteroidetes; Bacteroidia; Bacteroidales; Bacteroidaceae; Bacteroides |
Blautia luti | –1.4 | 2.92E-83 | Firmicutes; Clostridia; Clostridiales; Lachnospiraceae; Blautia |
Candidatus soleaferrea | –1.4 | 9.82E-66 | Firmicutes; Clostridia; Clostridiales; Ruminococcaceae |
Butyricicoccus spp. | –1.4 | 1.35E-64 | Firmicutes; Clostridia; Clostridiales; Clostridiaceae |
Blautia spp. | –1.3 | 7.26E-76 | Firmicutes; Clostridia; Clostridiales; Lachnospiraceae; Blautia |
Dorea spp. | –1.2 | 2.29E-64 | Firmicutes; Clostridia; Clostridiales; Lachnospiraceae |
Ruminococcus spp. | –1.2 | 1.08E-44 | Firmicutes; Clostridia; Clostridiales; Ruminococcaceae |
Clostridium clostridioforme | –1.2 | 2.17E-54 | Firmicutes; Clostridia; Clostridiales; Lachnospiraceae; Fusicatenibacter |
Bacteroides ovatus | –1.2 | 8.73E-69 | Bacteroidetes/Chlorobi group; Bacteroidetes; Bacteroidia; Bacteroidales; Bacteroidaceae; Bacteroides |
Bacteroides caccae | –1.0 | 3.33E-51 | Bacteroidetes/Chlorobi group; Bacteroidetes; Bacteroidia; Bacteroidales; Bacteroidaceae; Bacteroides |
Roseburia hominis | 1.0 | 1.82E-39 | Firmicutes; Clostridia; Clostridiales; Lachnospiraceae; Roseburia |
Eisenbergiella spp. | 1.0 | 3.96E-45 | Firmicutes; Clostridia; Clostridiales; Lachnospiraceae |
Veillonella parvula | 1.0 | 2.32E-38 | Firmicutes; Negativicutes; Selenomonadales; Veillonellaceae; Veillonella |
Enterococcus faecium or hirae–like | 1.1 | 2.56E-61 | Firmicutes; Bacilli; Lactobacillales; Enterococcaceae; Enterococcus |
Lactobacillus salivarius | 1.1 | 3.94E-48 | Firmicutes; Bacilli; Lactobacillales; Lactobacillaceae; Lactobacillus |
Lactobacillus fermentum | 1.2 | 1.02E-66 | Firmicutes; Bacilli; Lactobacillales; Lactobacillaceae; Lactobacillus |
Bacteroides dorei | 1.3 | 1.59E-82 | Bacteroidetes/Chlorobi group; Bacteroidetes; Bacteroidia; Bacteroidales; Bacteroidaceae; Bacteroides |
Solobacterium moorei | 1.3 | 1.93E-40 | Firmicutes; Erysipelotrichia; Erysipelotrichales; Erysipelotrichaceae; Solobacterium |
Rothia mucilaginosa | 1.4 | 1.05E-57 | Actinobacteria; Actinobacteria; Actinobacteridae; Actinomycetales; Micrococcineae; Micrococcaceae; Rothia |
Organism . | Correlation With Severe Acute Graft-Versus-Host Diseasea . | ||
---|---|---|---|
β/SEM(β) . | P (corr) . | Lineage . | |
Erysipelatoclostridium ramosum | –1.7 | 2.92E-84 | Firmicutes; Erysipelotrichia; Erysipelotrichales; Erysipelotrichaceae; Erysipelatoclostridium |
Bacteroides thetaiotaomicron | –1.5 | 3.68E-82 | Bacteroidetes/Chlorobi group; Bacteroidetes; Bacteroidia; Bacteroidales; Bacteroidaceae; Bacteroides |
Blautia luti | –1.4 | 2.92E-83 | Firmicutes; Clostridia; Clostridiales; Lachnospiraceae; Blautia |
Candidatus soleaferrea | –1.4 | 9.82E-66 | Firmicutes; Clostridia; Clostridiales; Ruminococcaceae |
Butyricicoccus spp. | –1.4 | 1.35E-64 | Firmicutes; Clostridia; Clostridiales; Clostridiaceae |
Blautia spp. | –1.3 | 7.26E-76 | Firmicutes; Clostridia; Clostridiales; Lachnospiraceae; Blautia |
Dorea spp. | –1.2 | 2.29E-64 | Firmicutes; Clostridia; Clostridiales; Lachnospiraceae |
Ruminococcus spp. | –1.2 | 1.08E-44 | Firmicutes; Clostridia; Clostridiales; Ruminococcaceae |
Clostridium clostridioforme | –1.2 | 2.17E-54 | Firmicutes; Clostridia; Clostridiales; Lachnospiraceae; Fusicatenibacter |
Bacteroides ovatus | –1.2 | 8.73E-69 | Bacteroidetes/Chlorobi group; Bacteroidetes; Bacteroidia; Bacteroidales; Bacteroidaceae; Bacteroides |
Bacteroides caccae | –1.0 | 3.33E-51 | Bacteroidetes/Chlorobi group; Bacteroidetes; Bacteroidia; Bacteroidales; Bacteroidaceae; Bacteroides |
Roseburia hominis | 1.0 | 1.82E-39 | Firmicutes; Clostridia; Clostridiales; Lachnospiraceae; Roseburia |
Eisenbergiella spp. | 1.0 | 3.96E-45 | Firmicutes; Clostridia; Clostridiales; Lachnospiraceae |
Veillonella parvula | 1.0 | 2.32E-38 | Firmicutes; Negativicutes; Selenomonadales; Veillonellaceae; Veillonella |
Enterococcus faecium or hirae–like | 1.1 | 2.56E-61 | Firmicutes; Bacilli; Lactobacillales; Enterococcaceae; Enterococcus |
Lactobacillus salivarius | 1.1 | 3.94E-48 | Firmicutes; Bacilli; Lactobacillales; Lactobacillaceae; Lactobacillus |
Lactobacillus fermentum | 1.2 | 1.02E-66 | Firmicutes; Bacilli; Lactobacillales; Lactobacillaceae; Lactobacillus |
Bacteroides dorei | 1.3 | 1.59E-82 | Bacteroidetes/Chlorobi group; Bacteroidetes; Bacteroidia; Bacteroidales; Bacteroidaceae; Bacteroides |
Solobacterium moorei | 1.3 | 1.93E-40 | Firmicutes; Erysipelotrichia; Erysipelotrichales; Erysipelotrichaceae; Solobacterium |
Rothia mucilaginosa | 1.4 | 1.05E-57 | Actinobacteria; Actinobacteria; Actinobacteridae; Actinomycetales; Micrococcineae; Micrococcaceae; Rothia |
Severe graft-versus-host disease is grade 3–4.
aAn ANCOM (analysis of composition of microbiomes)–style [25] analysis was performed. β/SEM(β) is the Wald statistic. The Bonferroni correction was used to correct P. Neutrophil recovery is the third consecutive day of an absolute neutrophil count >500 cells per mm3.
A Gradient of Positively to Negatively Correlated Organisms at Neutrophil Recovery Can be Used as a Metric to Assess Risk for Subsequent Severe Acute GVHD
For each microbial community we calculated a gradient of the sum of relative abundance of the positively correlated bacteria minus the sum of the relative abundance of the negative correlates. Figure 3A shows the gradient for all samples for our recipients stratified by acute GVHD grade; there is a slight trend to a higher gradient in those with higher grades of acute GVHD. Figure 3B shows the gradient for the microbial communities closest to neutrophil recovery; the trend is clearer toward a higher gradient being associated with higher grade of acute GVHD. Figure 3C is the ROC for this gradient as a test for development of severe acute GVHD (grade 3 or 4); the AUC of the ROC for this test is 0.83 (significantly better than the null hypothesis value of 0.5, P < 0.05 by permutation over 1000 iterations). Figure 3D shows the relationship between specificity and sensitivity for this metric at various cutoff values.
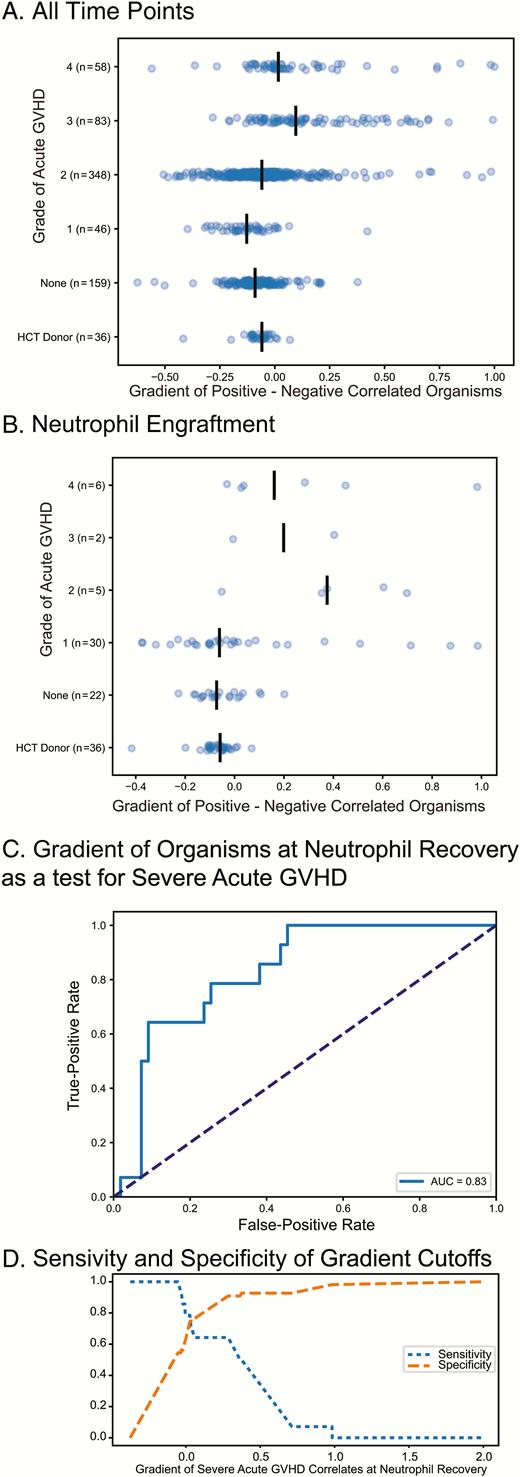
Positively and negatively correlated organisms at neutrophil recovery and graft-versus-host disease (GVHD). A gradient was calculated by taking the sum of the relative abundance of positive correlated organism (with GVHD) minus the sum of the relative abundance of the negative correlates. The value for this gradient is shown for all time points in panel A and for the time point closest to engraftment in panel B for hematopoietic cell transplant (HCT) recipients. C, The receiver operator characteristic for this gradient at neutrophil recovery as a predictor for development of severe (grade 3–4) acute GVHD. D, Sensitivity or specificity at various cutoff values for the gradient, allowing one to select a cutoff optimized for either specificity (ie, for a higher-risk intervention) or sensitivity (ie, for inclusion in a GVHD prophylaxis trial). Abbreviations: AUC, area under the curve; GVHD, graft-versus-host disease; HCT, hematopoietic cell transplantation.
DISCUSSION
In this study, we were able to directly correlate characteristics of the stool microbiome with acute GVHD, further establishing the microbiome as a predictor of GVHD. We noted a trend toward greater phylogenetic distance between donor and recipient stool microbiota with higher grades of acute GVHD. We found a trend toward lower alpha diversity in patients with severe acute GVHD. We described several distinct bacterial species positively or negatively correlated with the subsequent development of severe acute GVHD when present around neutrophil recovery and used these organisms in a metric to predict subsequent severe acute GVHD. Our results are broadly comparable with the prior published work [6, 7] but point to specific species rather than alpha diversity as a more predictive microbiome factor for eventual development of severe acute GVHD.
We note that the onset of acute GVHD can be hyperacute, occurring right as neutrophil recovery occurs. In 5 of the 14 patients who went on to develop severe acute GVHD, the onset of 14 GVHD symptoms was with the recovery of neutrophils, with the more severe GVHD symptoms occurring later. Thus, our metric remains useful for predicting the subsequent development of severe GVHD.
While this study is focused on correlations between the stool microbiota and acute GVHD and their potential to be used as a biomarker of disease risk, our results and those of other groups [6, 7] point to several possible mechanisms by which the gut microbiome could play a direct role in modulating GVHD. Typically oral Actinobacteria and oral Firmicutes in the gut were positively correlated with subsequent development of severe GVHD in our study (a novel association with GVHD, not previously published). Others have implicated the same family of organism with gut inflammation in colorectal carcinoma [28, 29] and to gut damage [30] in a model system of HCT. Several studies have shown a correlation between antibiotic exposure and risk for death from severe GVHD [31–33], particularly antibiotics with an anaerobic spectrum [8]. Such agents may open new ecologic niches for these typically oral organisms in the gut.
Different species in the Bacteroides genus were associated with different effects, with B. dorei positively correlated with GVHD and B. ovatus, B. caccae, and B. thetaiotaomicron negatively correlated in our study. These differences could be explained by differences in metabolic capability, virulence, or ability to promote inflammation among these organisms in the same genus. Metabolites have also been considered as a correlate with severe GVHD [34], specifically with changes in mucin metabolism. A better understanding of the metabolic capability and activity of the gut microbiome in HCT may offer insight into how the microbiome can affect immune recovery post-transplant [35–37].
Our findings include a possible relationship between the HCT donor and recipient microbiota, as measured by phylogenetic distance and risk of GVHD [38]. Both the composition of the microbiota and closeness to the HCT donor’s microbiota may be important factors to consider when attempting future interventions such as fecal microbiota transplantation [39, 40]. This observation requires confirmation.
Human clinical trials in the 1980s and early 1990s suggested a benefit from antibiotic decontamination of the gut prior to transplant; decontamination became the standard of care [41, 42]. By the late 1990s, after the introduction of immunosuppressant prophylaxis, clinical studies revealed a more mixed effect from aggressive gut decontamination, even the possibility of increased GVHD [43–45]. Understanding the mechanisms by which the microbiota affect risk of GVHD will be needed to reconcile these results with the current studies on the microbiome and GVHD.
This study is limited by the intrinsic constraints of 16S-SSU profiles of a microbiota where we assess relative abundance of sequence reads, not absolute concentrations of bacteria. Certain clades of organisms (including the Clostridiales and Enterobacteraceae) can be difficult to resolve via 16S rRNA gene amplicon-based profiling [22]. Samples were at room temperature without preservative for up to 72 hours prior to being stored at −80oC. This makes the study conditions easier to reproduce in the clinical setting but could have resulted in shifts in the composition of the microbiota samples due to blooms or loss of organisms in that time window [46]. In future studies, we would like to explore the effect of enteral vs parenteral nutrition, antacid use, and other factors that may correlate with the shift to oral organisms we observed in severe GVHD. As with other published studies, this was a single-center study, and therefore our findings may not be generalized to all centers. We provide a novel metric to assess risk of GVHD so that other centers can validate or refute this approach to risk assessment.
In conclusion, we demonstrate that the stool microbiota can be a useful predictor for subsequent development of acute severe GVHD when measured around the time of neutrophil recovery post-transplant. Determining which HCT patients are most likely to develop severe acute GVHD would allow clinicians to target these patients for more aggressive interventions, including use of preemptive immunomodulatory agents or efforts to modify gut microbial communities.
Supplementary Data
Supplementary materials are available at Clinical Infectious Diseases online. Consisting of data provided by the authors to benefit the reader, the posted materials are not copyedited and are the sole responsibility of the authors, so questions or comments should be addressed to the corresponding author.
Notes
Acknowledgments. The authors thank Noah Hoffman for providing code and tools to refine our classification pipeline and Timothy Randolph for advice on statistical analysis.
Financial support. This work was supported in part by an institutional grant from the Vaccine and Infectious Disease Division at the Fred Hutchinson Cancer Research Center and through a generous donation. J. L. G. is supported by the Joel Meyers Endowment.
Potential conflicts of interest. S. A. P. has served as consultant and has participated in clinical trials with Merck, Chimerix, and Optimer/Cubist. All remaining authors: No reported conflicts. All authors have submitted the ICMJE Form for Disclosure of Potential Conflicts of Interest. Conflicts that the editors consider relevant to the content of the manuscript have been disclosed.
References