-
PDF
- Split View
-
Views
-
Cite
Cite
Mark G. Kortepeter, Barbara J. Seaworth, Sybil A. Tasker, Timothy H. Burgess, Rodney L. Coldren, Naomi E. Aronson, Health Care Workers and Researchers Traveling to Developing-World Clinical Settings: Disease Transmission Risk and Mitigation, Clinical Infectious Diseases, Volume 51, Issue 11, 1 December 2010, Pages 1298–1305, https://doi.org/10.1086/657115
- Share Icon Share
Abstract
With the recent emphasis on funding and training opportunities for global health and humanitarian aid and the increased interest in the field, many health care workers and medical researchers are traveling from resource-replete to resource-limited settings. This type of travel brings unique disease risks not routinely considered for the business or vacationing traveler. This review provides practical advice for this special population of travelers, targeted to specific health care-related risks (needlestick, hemorrhagic fever viruses, severe viral respiratory disease, and tuberculosis), with suggestions for risk mitigation.
Few data on the epidemiology of infectious diseases occurring among traveling health care workers (HCWs) exist. Surveillance systems do not classify this group separately from business or vacationing travelers but record them instead as tourists, missionaries, or others. Furthermore, HCWs are a diverse group, ranging from short-term travelers to workers in refugee camps; consequently, their individual activities and travel destinations around the globe pose varied risks.
It is important to consider the intersection of the host, agent, vector, and environment. HCWs, because of their occupation, travel to regions of high disease endemicity and then compound the risk by working in the specific microenvironment of the health care setting. High concentrations of potentially infectious human vectors (patients and other staff) and an environment that often does not offer the protections present in the developed world (such as engineering controls and personal protective equipment [PPE]) place traveling HCWs at greater risk for infection. All these factors need to be addressed in determining risks and preparing a mitigation plan before, during, and after travel. Providing prophylaxis and vaccinations, bringing PPE, and having medical countermeasures (such as anti-retrovirals and antibiotics) on hand must all be considered (Table 1).
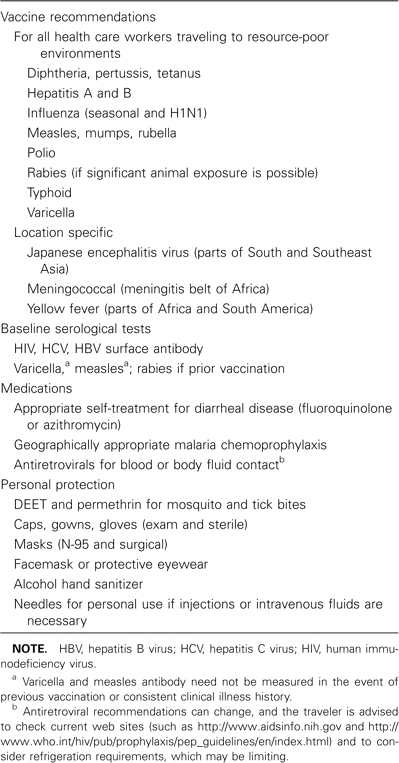
Needlestick Exposure
Pretravel advice for managing needlestick injuries should start with a discussion of prevention. Needlesticks may be more likely to occur in crowded, rushed, and unfamiliar work settings [1]. Advise HCWs to set up a “sharps container” if one is not available. Potential substitutes include an empty container, such as a soda can or plastic laundry detergent bottle.
Awareness of the disease epidemiology of the region and among potential patients will help in risk assessment should a needlestick injury occur. In addition to human immunodeficiency virus (HIV) and hepatitis viruses, other viruses can be transmitted by needlestick, such as dengue virus and other hemorrhagic fever viruses. Other potential disease risks include syphilis, mycobacterial and rickettsial infections, trypanosomiasis, visceral leishmaniasis, and malaria [2]. Malaria is particularly notable, because the risk of disease from needlestick may be higher than that of hepatitis viruses or HIV and because fatal and near-fatal cases have occurred as a result of diagnostic delays [3]. Knowing the prevalence of drug resistance for needlestick-transmissible endemic diseases is also useful.
If a needlestick injury occurs, HCWs should note the source patient's region of origin, presenting complaints, history, and physical findings and perform a rapid HIV test or obtain a blood sample for later testing. Wash the injury site thoroughly with warm water and soap. Caustic antiseptics (eg, bleach and povidone iodide) are not recommended, because they may actually increase the risk of infection as a result of local tissue injury and recruitment of inflammatory cells [4]. A clear plan for follow-up assessments and points of contact is essential. Given that diagnostic testing for hepatitis viruses and HIV may be limited at overseas destinations, HCWs should consider completing baseline tests for them before travel.
Hepatitis B virus (HBV) is one of the most transmissible viruses related to needlestick and is generally more common in resource-limited settings than in the United States. The risk to unvaccinated persons is 37%–62% if the source is positive for hepatitis B e antigen (HBeAg) and is 23%–37% if the source is negative for HBeAg and positive for hepatitis B surface antigen (HBsAg). Transmission from environmental surfaces can occur even without a cutaneous injury [4]. Fortunately, HBV vaccination is highly effective and eliminates this risk for the 90% who respond to the primary series. For those who do not respond, repeat vaccination may protect 30%–50%. Before repeating the series, check antibody to hepatitis B core antigen and HBsAg to ensure that the patient is not a chronic HBV carrier. Deep intramuscular injection with a longer needle may be needed for larger recipients [4]. If serostatus is negative after 6 immunizations, there is no benefit to additional vaccine. Seronegative HCWs remain at high risk for hepatitis B if exposed, and hepatitis B immunoglobulin (0.06 mL/kg intramuscularly) should be offered after injury and repeated in 1 month [4]. Hepatitis B immunoglobulin started within 1 week of exposure provides ∼75% protection [4]. Tenofovir and lamivudine have activity against HBV, but there is no guidance for prophylactic use in this setting. Consider administration of hepatitis B immunoglobulin before departure for seronegative HCWs when concern exists for HBV exposure in a remote setting. Passive antibody half-life is 3 weeks, and levels are measurable for ∼2 months [5].
Although no prophylaxis for hepatitis C virus (HCV) exposure exists, the needlestick transmission risk is lower (1.8%), and up to 20% of transmitted infections resolve spontaneously. The incubation period is 4–12 weeks. Exposed individuals should be monitored with HCV RNA testing, if available. Immediate treatment is not recommended in light of the significant toxicity and duration of therapy and because some infections will resolve spontaneously, but treatment should be considered if the HCV infection remains established after 12 weeks [6].
Most feared is HIV infection after needlestick, but it has the lowest risk of transmission (0.3%). Time to initiation of postexposure prophylaxis (PEP) is critical. PEP is most effective if started immediately and definitely should be started within 3 days of exposure; PEP must be continued for 4 weeks [4, 7, 8]. Unlike HIV treatment, for which the use of triple-drug combinations is an essential success factor, 3 drugs are unlikely to be significantly better than 2 for PEP and paradoxically may be less effective, given that adherence to 3-drug regimens is poorer because of toxicity of the multidrug regimen. Zidovudine monotherapy is 79% effective in preventing infection [7]. The World Health Organization (WHO) recommends using 2 nucleoside reverse-transcriptase inhibitors from the country's first-line regimen and 3 drugs if the source patient is known to be infected with drug-resistant HIV or if there is >15% resistance in the community [8]. The Centers for Disease Control and Prevention (CDC) recommends a 3-drug PEP regimen if the source patient is known to be infected with HIV and the source device is a hollow-bore needle or has visible blood contamination. The CDC recommends zidovudine, stavudine, or tenofovir plus emtricitabine or lamivudine. When a third drug is included, both the CDC and WHO recommend a ritonavirboosted protease inhibitor, not nonnucleoside reverse-transcriptase inhibitors [8, 9]. HCWs are strongly encouraged to bring postexposure medications to their destination.
Follow up for needlestick injury should include a high level of suspicion for any febrile illness in the weeks after the incident; serological tests for HIV, hepatitis, and syphilis at ∼3 months; and adherence support if antiretrovirals are given. HIV RNA should be checked if there is an acute febrile illness after an HIV-positive needlestick exposure. If HCV RNA measurements are available, they can be monitored at 2, 6, 12, and 24 weeks after a high-risk exposure.
Hemorrhagic Fever Viruses
Viral hemorrhagic fever (VHF) is an acute febrile syndrome, accompanied with a bleeding diathesis, caused by 4 families of single-stranded RNA viruses (filoviruses, arenaviruses, bunyaviruses, and flaviviruses) [10, 11]. Nosocomial spread and infection of HCWs are potential risks but can be mitigated by early recognition and appropriate precautions.
The first step in protection is to know which viruses are endemic to the local area, as shown in Table 2 [12, 14]. Humans are infected via the bite of an arthropod vector, inhalation or ingestion of rodent excretions, or mucosal or percutaneous contact with infected blood or secretions of patients [10]. In underresourced settings, spread of these viruses in the hospital environment is often facilitated by lack of recognition, reuse of equipment (such as contaminated needles), and inconsistent enforcement of basic infection-control practices, frequently because of inadequate supplies.
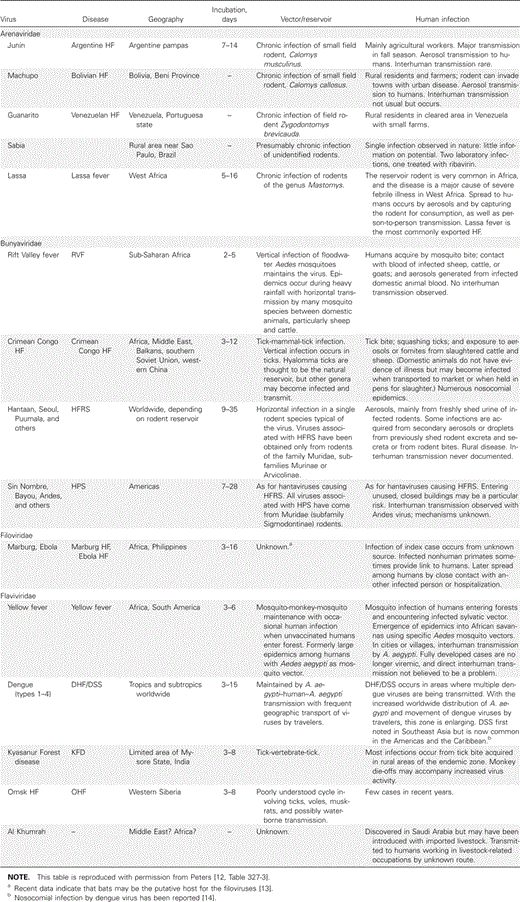
Clinical diagnosis of VHF requires an index of suspicion and is challenging, especially in underdeveloped regions, where the broad differential diagnosis of acute febrile illness includes malaria, typhoid, rickettsial illness, and leptospirosis. Common presenting symptoms include acute onset of fever, malaise, headache, myalgias, and prostration, frequently accompanied by gastrointestinal complaints. Early signs may include pharyngitis, conjunctivitis, cutaneous flushing, or a maculopapular rash. Hemorrhagic manifestations may include oozing at intravenous puncture sites, petechiae, purpura, large ecchymoses, or frank hemorrhage; however, manifestations, frequency, and severity of bleeding varies depending on the agent [11, 12]. Case fatality rates can range from <1% for Rift Valley fever to nearly 90% with Ebola and Marburg fever [15].
Specific diagnosis can be made on the basis of detection of virus or viral antigens in serum, plasma, or whole blood by antigen-capture or antibody-detection enzyme-linked immunosorbent assay and reverse-transcription polymerase chain reaction. Viral isolation should be attempted only in a biosafety level 4 containment laboratory, such as those at the CDC or the US Army Medical Research Institute of Infectious Diseases [11].
All hemorrhagic fever viruses except dengue virus are known to infect via artificially produced aerosols in a laboratory environment. Potential spread between laboratory animals has raised concern about potential person-to-person airborne transmission [10, 16]. However, the epidemiology of VHF outbreaks indicates that if person-to-person airborne transmission does occur, it is rare [15]. In the 1995 Zaire Ebola epidemic, infections of HCWs declined dramatically after the institution of isolation precautions [17]. However, achieving universal adherence to strict isolation precautions in hot, humid conditions in such environments is a challenge, as demonstrated in a 2000 Ebola outbreak in Uganda [18].
Specific guidance for infection control is available [19]. Standard, contact, and droplet precautions should be used in a private room for both inpatient and outpatient settings. Although airborne precautions are not required in earlier stages of illness, they should be considered early (1) to eliminate the need for later transfer to an airborne isolation room, (2) for severe pulmonary involvement, and (3) for certain activities that might stimulate coughing or generate aerosols (eg, bronchoscopy and endotracheal intubation) [19, 20].
The WHO and CDC have developed VHF infection-control recommendations for African health care settings that are applicable to other austere environments [21]. Their handbook recommends practical measures for establishing standard precautions for all patients, routine hand washing, and safe handling and disposal of used needles and syringes. Upgrading to VHF precautions includes isolating the patient and wearing protective clothing (scrub suit, gown, apron, gloves, mask, headcover, eyewear, and rubber boots). HCW training should occur in advance, and access to patient care areas should be limited to those with a definite need. The CDC identifies 3 levels of risk (casual, close, and high-risk contacts) for potential exposure, along with follow-up recommendations [22].
The only VHF vaccine currently licensed in the United States is for yellow fever. Investigational vaccines exist for several other VHFs; however, they are undergoing animal or clinical testing, are only for laboratory workers, or are not generally available [10]. Medical countermeasures are limited. In a postexposure situation, expert consultation for treatment or prophylaxis measures is recommended.
Severe Respiratory Viral Infections
Recently, emergent severe respiratory viral diseases have prompted intensified research and public health concern. A coronavirus causing severe acute respiratory syndrome (SARS-CoV) spread rapidly in 2003, resulting in a pandemic with frequent nosocomial spread [23]. The reemergence in the human population of avian influenza A(H5N1) in 2003 raised international alarm over a potential novel influenza pandemic. Widespread infection in poultry and wild birds continues to cause occasional human cases in Southeast Asia and the Middle East. Although human-to-human transmission is limited, the case fatality rate exceeds 60% [24]. Most recently, influenza A(H1N1), a reassortment zoonotic influenza virus, emerged in 2009 in North America and spread throughout the world, resulting in a pandemic declaration by June 2009. As of April 2010, >300,000 confirmed cases (producing at least 17,700 deaths) have been reported from >200 countries, and the pandemic virus has effectively replaced previously circulating seasonal influenza strains [25].
Researchers and HCWs face obvious risks for occupational acquisition of respiratory diseases. Limited data to quantify the actual risk exist, although compelling anecdotes document the potential danger [26]. Protective measures to mitigate risk for those with exposure include contact and respiratory precautions. Optimal strategies depend on the nature of the pathogen, its known or suspected transmission routes, and exposure circumstances.
Transmission of SARS-CoV appears to occur predominantly through close interactions with infected persons. During the pandemic, transmission to HCWs occurred after close, unprotected contact with symptomatic persons and was significantly mitigated once infection-control precautions were implemented; the degree of risk was related to the type and intensity of exposure (endotracheal intubation was significantly associated with contracting SARS) [27, 28]. Airborne transmission of SARS-CoV is clearly suggested by modeling studies, retrospective evaluation of nosocomial outbreaks, and anecdotal reports of transmission aboard commercial aircraft [29–31].
Transmission of human influenza viruses occurs via large droplets, direct and indirect contact, and droplet nuclei. The relative contribution of each route is not known and likely varies with the setting and circumstances. There are conflicting opinions regarding the importance of airborne transmission of influenza viruses in humans [32–34]. Direct contact may be more important than inhalation in conditions of high humidity and temperature [35–37].
Frequent and diligent hand hygiene is likely the most important intervention for the interruption of transmission for many respiratory viruses, particularly influenza virus. When clean water is not available in field settings, alcohol-based hand gels should be used. These gels and/or washing with soap and water have demonstrated effectiveness [38]. Personnel should be trained in and use protective clothing, gloves, shoe covers, goggles, and masks.
The principal question with respect to respiratory protection is whether the mask should be a fitted, high-efficiency, particlefiltering N95 respirator. The answer depends on the relative contribution of aerosols versus large droplets to disease transmission. The WHO cites evidence from observational studies in hospitals suggesting that droplet transmission is responsible for the majority of nosocomially acquired cases to recommend standard plus droplet precautions for persons with seasonal influenza [39]. Recent results suggest that surgical masks are effective for preventing seasonal influenza, although some controversy exists [40]. During the 2009 H1N1 pandemic, an Institute of Medicine panel recommended that HCWs use N95 masks, given the uncertainty regarding risks of transmission [41]. In resource-limited settings, priority should be given to risk-reduction interventions focusing on hand hygiene, contact precautions, expedient respiratory protection, and the use of PPE that is effective for aerosol respiratory protection, particularly during exposures likely to generate aerosols (eg, intubation).
Although heterotypic protection against novel strains and pathogens cannot be expected, influenza vaccination is recommended for individuals involved in respiratory disease research and for HCWs caring for such patients, unless contraindicated. Vaccination is recommended to decrease the risk of illness due to seasonal influenza virus, reducing the frequency of diagnostic and management dilemmas for those occupationally involved in close contact with individuals with severe respiratory disease, such as that caused by influenza A(H5N1) or SARS. Prevention of influenza by means of chemoprophylaxis with neuraminidase inhibitors is a potential strategy and may be indicated in certain settings, such as direct contact with sources of avian influenza [39].
Drug-Resistant Tuberculosis
In general, tuberculosis is a potential threat to HCWs and researchers who travel to work in high-burden countries [42]. Approximately 500,000 new cases of multidrug-resistant (MDR) tuberculosis, defined as tuberculosis resistant to isoniazid and rifampin, were reported in 2008, and 1% were treated according to WHO standards [43]. Extensively drug-resistant (XDR) tuberculosis, defined as MDR tuberculosis plus resistance to fluoroquinolones and a second-line injectable (kanamycin, amikacin, or capreomycin), has been reported in 58 countries [44].
MDR and XDR tuberculosis pose special risks. Recognition of infectious individuals is difficult because of the lack of laboratory infrastructure for culture and susceptibility testing. Treatment of MDR and XDR tuberculosis is prolonged, with significant toxicity and poor outcomes. Treatment completion or cure is reported for 46%–87% of MDR cases and for 29%71% of XDR cases [45–49].
The epidemiology of drug-resistant tuberculosis is described in the WHO fourth global report [50]. Information is incomplete for some regions, especially Africa, but medical literature identifies a serious problem with MDR and XDR tuberculosis there [51, 52]. China represents 25% of the global burden, and India represents >20%. Countries of the former Soviet Union have the highest proportion of drug resistance; 20% of all cases are MDR tuberculosis. Other countries with a high proportion of MDR tuberculosis (rates of >3%) are Vietnam, Thailand, Korea, the Philippines, Peru, and Guatemala [50].
Because of good infection control, at-risk screening, and treatment of latent tuberculosis, nosocomial transmission is rare in the United States. Exposure overseas may occur in locations with uncontrolled transmission and inadequate infection-control practices. Exposure is likely not only in hospitals but also in clinics, hospices, prisons, factories, mines, orphanages, and offices [51]. Most HCWs are not counseled regarding tuberculosis risks before departure, nor are they evaluated on their return. Many are unaware of the risk, and if symptoms develop the diagnosis is often delayed, allowing potential transmission to vulnerable populations in US medical facilities.
Before departure, the risk of tuberculosis at the destination should be assessed. HCWs should be screened for latent tuberculosis by an interferon γ release assay (IGRA) or the tuberculin skin test (TST). IGRAs are more specific and are preferred for those with a history of bacille Calmette-Guérin (BCG) vaccination. If the results of TST and IGRA are negative, vaccination with BCG may be considered 2–6 months before departure (B.J.S., personal communication). Two months after returning, rescreen with an IGRA. Fit testing with either a disposable filtering facepiece respirator or a reusable elastomeric respirator is advised. For those with facial hair, an adequate seal is not possible and a powered air purifying respirator is needed. Respiratory protection should be brought to the destination.
WHO infection-control guidelines for resource-limited countries suggest measures to limit transmission, including patient cohorting, individual respiratory protection, and natural ventilation [53]. Engineering controls (such as negative air pressure) are usually not available; therefore, HCWs should minimize the time spent in high-risk areas with poor ventilation when possible. Personal respiratory protection is the main option for limiting exposure [54].
Treatment of latent tuberculosis is strongly recommended for US HCWs. If latent tuberculosis is detected before departure, it is often best to delay treatment until return. The CDC recommends treatment of latent tuberculosis with 9 months of isoniazid or 4 months of rifampin [55]. When latent tuberculosis is diagnosed after travel to high-risk areas, infection with MDR or XDR tuberculosis must be considered. If exposure to MDR or XDR tuberculosis is not reported or is unlikely, standard treatment is indicated. When the risk is significant or definite exposure occurs, treatment based on the suspected or known susceptibility of MDR and XDR tuberculosis exposure can be considered. This approach is often taken in the United States but is based on expert opinion and is controversial. Treatment may include 6–12 months of either ethambutol or pyrazinamide with levofloxacin or moxifloxacin [56]. The CDC recommends clinical and radiographic follow-up for 24 months for those with latent tuberculosis likely due to MDR or XDR pathogens, regardless of whether treatment of latent tuberculosis occurs. If tuberculosis develops, rapid identification of resistance is critical. Molecular detection of drug resistance is available at the CDC and at some state health departments. Consultation with an expert is strongly advised. Assistance is available from health departments or the CDC's Regional Training and Medical Consultation Centers [57].
Conclusion
Medical researchers and health care workers who travel to resource-limited settings to conduct patient care or research compose an understudied travel population. The nature of their work, restricted provision of supplies, and potentially limited use of infection-control practices puts them at unique risk for acquiring specific infections. This review provides practical guidance to mitigate the risk of potential occupational infectious diseases transmission in such settings and aspires to raise interest in further study of how to best to protect these individuals.
Acknowledgments
Potential conflicts of interest. All authors: no conflicts.
References
Disclaimer: The views expressed in this article are those of the authors and do not reflect the official policy of the Department of the Army, the Department of the Navy, the Department of Defense, or the US government.