-
PDF
- Split View
-
Views
-
Cite
Cite
Richarda M. de Voer, Fiona R. M. van der Klis, Judith E. Nooitgedagt, Florens G. A. Versteegh, Johannes C. M. van Huisseling, Debbie M. van Rooijen, Elisabeth A. M. Sanders, Guy A. M. Berbers, Seroprevalence and Placental Transportation of Maternal Antibodies Specific for Neisseria meningitidis Serogroup C, Haemophilus influenzae Type B, Diphtheria, Tetanus, and Pertussis, Clinical Infectious Diseases, Volume 49, Issue 1, 1 July 2009, Pages 58–64, https://doi.org/10.1086/599347
- Share Icon Share
Abstract
Background. Maternal antibodies contribute to the protection of neonates from infectious diseases during the first months of life. The seroprevalence of antibodies specific for polysaccharide or protein antigens from vaccine-preventable pathogens was determined in paired maternal delivery and cord blood serum samples.
Methods. Antibody concentrations specific for Neisseria meningitidis serogroup C polysaccharide, Haemophilus influenzae type B polysaccharide, diphtheria toxin, tetanus toxin, and pertussis toxin, filamentous hemagglutinin, and pertactin from Bordetella pertussis were determined by enzyme-linked inmmunosorbent assay (ELISA), fluorescent multiplex immunoassay, or serum bactericidal assay.
Results. We investigated 197 paired maternal delivery and cord blood samples. The mean maternal age was 30.8 years, and the mean gestational age was 39.3 weeks. Cord geometric mean concentrations (GMCs) were 0.23 µg/mL for N. meningitidis serogroup C and 0.53 µg/mL for H. influenzae type B. Cord GMCs to diphtheria and tetanus were 0.16 and 1.06 IU/mL, respectively, and cord GMCs to pertussis toxin, filamentous hemagglutinin, and pertactin were 16.2, 34.8, and 17.7 ELISA U/mL (by ELISA), respectively. Cord GMCs to polysaccharide were, in general, 107% identical to maternal GMCs, whereas cord GMCs to proteins were a mean of 157% of maternal concentrations. In addition, the levels of anti-N. meningitidis serogroup C immunoglobulin G1 and G2 in cord blood were 145% and 109% of maternal concentrations, respectively.
Conclusions. Antibody concentrations directed toward polysaccharide were equal in maternal and cord blood, whereas antibody concentrations to proteins were 1.6 times higher in cord blood than in maternal blood. This is probably attributable to the less-active transportation of immunoglobulin G2 antibodies elicited by polysaccharide. Despite proper placental transfer, cord antibody concentrations are low, possibly placing neonates at risk before they receive their primary vaccinations.
Clinical trials registration. http://www.controlled-trials.com/ISRCTN14204141/
In industrialized countries, many serious infectious diseases that can occur in children are prevented by routine infant vaccination. Newborns, however, remain at risk because of an immature immune defense, particularly in the instance of low herd immunity, which provides indirect protection. Protection by development of specific humoral immunity matures with age and exposure or vaccinations. Therefore, in the first months, newborns also depend on maternal antibodies supplied by placental transfer. The nature and duration of protection relies on the concentration of specific antibodies from the mother and the capacity of the mother to transfer these to the infant [1-3].
Transfer of maternal antibodies is mainly restricted to immunoglobulin G (IgG) antibodies. Antibody transfer from mother to infant is an active process that starts early, around the gestational age of 16 weeks, but an abundance of IgG is acquired later, during the last 4 weeks of full-term pregnancy [4]. The transportation of IgG is likely to depend on placental receptors for the Fc part of the antibody (IgG Fc receptor [FcγR]). FcγR I and III have a preference for IgG1 and IgG3 and low affinity for IgG2 [5]. This has potential impact on the transfer of antibodies directed to different antigens, because polysaccharides are likely to elicit more IgG2 antibodies [6, 7], whereas IgG1 and IgG3 subclasses are directed primarily to proteins [8, 9]. Factors such as gestational age of the infant at birth, placental abnormalities, and the concentration of specific IgG subclasses in the mother also influence the concentrations in the newborn [9-11].
At present, vaccination of the mother during pregnancy for protection of the newborn is recommended in particular circumstances [12]. In the Netherlands, this is not current practice, and protection of neonates by transferred maternal antibodies depends on the presence of antibodies in the mother during pregnancy. In the Netherlands, >95% of women have received childhood immunizations, but antibody concentrations decrease over time. Furthermore, adolescent immunizations are not routine in the Netherlands. This may lead to antibody titers below protective concentrations in both the mother and the infant [1, 2, 13, 14]
In the Dutch National Immunization Programme, primary vaccinations are currently administrated at 2, 3, and 4 months of age, with a booster vaccination at 11 months (e.g., diphtheria [Dtx], tetanus [Ttx], pertussis, and Haemophilus influenzae type b [Hib; implemented since 1993]). Some vaccines are administered at a later age (e.g., the Neisseria meningitidis serogroup C [MenC] conjugate vaccine is offered once, at the age of 14 months [since 2002]). In this study, we evaluated the antibody concentrations in paired maternal and cord serum samples from a large cohort of mothers and newborns. We focused on antibodies directed toward polysaccharides from the conjugate vaccines against MenC and Hib and toward several protein-derived vaccines: Dtx, Ttx, and 3 antigens from Bordetella pertussis (pertussis toxin [Ptx], filamentous hemagglutinin [FHA], and pertactin [Prn]).
Patients, Materials, and Methods
Study population. All mothers and neonates participated in a study to evaluate the incidence of B. pertussis infection during and shortly after pregnancy that was performed during 2002–2006. Participants eligible for enrollment were pregnant women who delivered a newborn at the Groene Hart Hospital in Gouda, the Netherlands. Blood samples were obtained from mothers immediately after delivery (maternal serum) and from the umbilical cord (cord serum). In the current study, we included 197 maternal and cord blood pairs. All participants had provided informed consent at the time of enrollment for their anonymized samples to be used for future research on maternal and/or infant infectious diseases.
Laboratory methods. MenC-specific IgG, IgG1, and IgG2 antibodies were quantified using a fluorescent bead-based multiplex immunoassay [15]. Standardized reference serum sample CDC 1992 was used in this assay (National Institute for Biological Standards and Control).
Hib-specific antibodies were quantified by an enzyme-linked immunosorbent assay (ELISA) described in detail by Mariani et al. [16], with minor modifications. Standardized reference serum lot 1983 was used in this assay (Center for Biologics Evaluation and Research, Food and Drug Administration [CBER-FDA]), and a different secondary conjugated antibody was used: alkaline phosphatase conjugated goat antihuman IgG (Sigma).
Dtx-, Ttx-, and pertussis (Ptx, FHA, and Prn)-specific antibodies were quantified by ELISA, as described elsewhere [17-19]. In-house reference serum samples were calibrated against international standards Di-03 (Dtx), TE-03 (Ttx; National Institute for Biological Standards and Control), lot 3 (Ptx and FHA; CBER-FDA), and lot 4 (PRN; CBER-FDA).
The concentration of MenC-specific functional antibodies was determined by a serum bactericidal antibody (SBA) assay [20] with use of baby rabbit complement (Pel-Freeze). The target strain for the assay was C11. SBA titers are expressed as the reciprocal of the final serum dilution yielding 50% killing at 60 min.
Data analysis. For statistical analysis, titers below the lower limit of quantitation were assigned half the lower limit of quantitation (0.05 µg/mL for Hib, 0.005 IU/mL for Dtx and Ttx, 1 ELISA U/mL for Ptx and FHA, and 2 ELISA U/mL for Prn). The lower limit of quantitation for MenC antibodies was assigned at 0.01 µg/mL. Antibody concentrations of MenC, Hib, Dtx, Ttx, and pertussis in serum samples were calculated as geometric mean concentrations (GMCs) with 95% confidence intervals (CIs). For each antigen, comparisons of the specific antibody concentrations to MenC, Hib, Dtx, Ttx and pertussis in maternal serum and cord serum samples were performed using Student's t test. Placental transfer of antibodies to the antigens was defined by calculating individual ratios for each paired maternal and cord serum sample. In addition, the total ratio for each antigen was defined as the ratio of cord GMC to maternal GMC in paired samples.
Results
Study participants. In the original pertussis study, 315 mothers were enrolled from January 2004 through February 2006. Among these mothers, 197 mother and neonate pairs were available for the present study. The numbers of maternal and cord serum samples that were tested for each of the antigens are shown in table 1(numbers depend on the quantity of available serum). The mean maternal age was 30.8 years (range, 17–44 years), and the mean gestational age was 39.3 weeks (range, 33–42.5 weeks). Approximately 36% of the women who delivered a neonate were ⩾30 years of age. The distribution of female and male newborns was 48.7% and 51.3%, respectively.
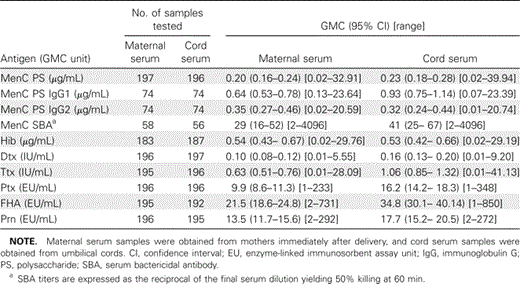
Number of samples tested and geometric mean concentrations (GMCs) of antibodies to Neisseria meningitidis serogroup C (MenC), Haemophilus influenzae type b (Hib), diphtheria (Dtx), tetanus (Ttx), pertussis toxin (Ptx), filamentous hemagglutinin (FHA), and pertactin (Prn) in maternal and cord serum samples.
Seroprevalence of MenC and Hib polysaccharide-specific IgG in maternal and cord serum samples. Table 1presents the GMCs, 95% CIs, and ranges of anti-MenC and anti-Hib capsular polysaccharide antibodies in maternal and cord serum samples. The percentage of transfer of maternal anti-MenC and anti-Hib polysaccharide antibodies was 115% and 98% (mean, 107%), respectively. Individual maternal and cord antibody concentrations to MenC and Hib polysaccharides are shown in figure 1B. Individual ratios of cord-to-maternal antibody concentrations are shown in figure 2.
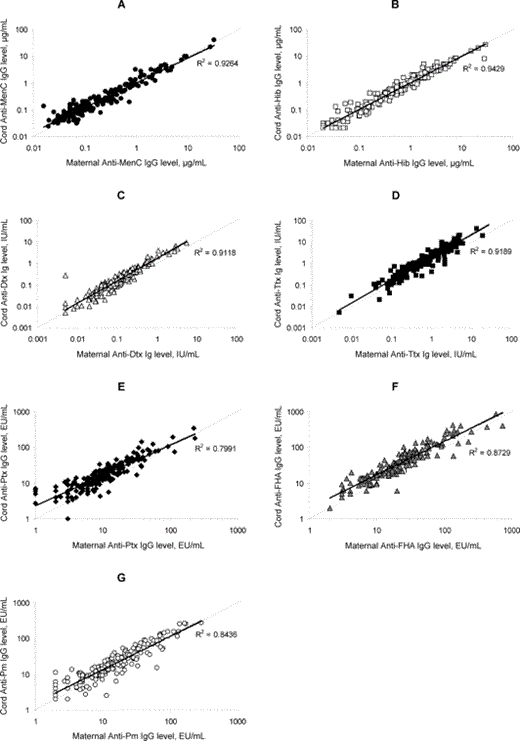
Individual maternal-to-cord antibody concentrations of anti-Neisseria meningitidis serogroup C (MenC) immunoglobulin G (IgG; A ), anti-Haemophilus influenzae type b (Hib) IgG (B), anti-diphtheria (Dtx) Ig (C), anti-tetanus (Ttx) Ig (D), anti-pertussis toxin (Ptx) IgG (E), anti-filamentous hemagglutinin (FHA) IgG (F), and anti-pertactin IgG (G). EU, enzyme-linked immunosorbent assay unit.
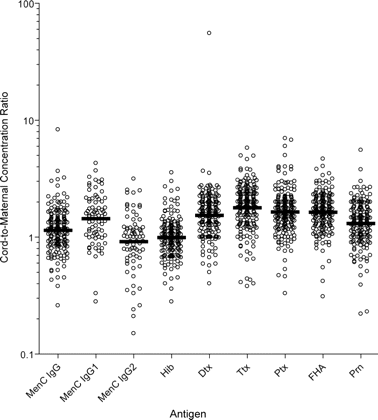
Individual cord-to-maternal ratios of anti-Neisseria meningitidis serogroup C (MenC) immunoglobulin G (IgG), IgG1, and IgG2; anti-Haemophilus influenzae type b (Hib); anti-diphtheria (Dtx); anti-tetanus (Ttx); anti-pertussis toxin (Ptx); anti-filamentous hemagglutinin (FHA); and anti-pertactin (Prn) antibodies. Horizontal bars indicate geometric mean cord-to-maternal ratios.
MenC-specific SBA titers, 95% CIs, and ranges are shown in table 1. The percentage of placental transfer of MenC-specific serum bactericidal antibodies was higher than that of MenC polysaccharide antibodies alone (141% vs. 115%).
In addition, anti-MenC polysaccharides IgG1 and IgG2 subclasses were determined in a subset of paired maternal and cord serum samples. MenC polysaccharide-specific IgG1 and IgG2 GMCs, 95% CIs, and ranges are presented in table 2.
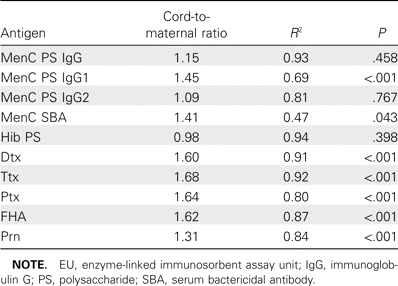
Individual cord-to-maternal concentration ratios and correlations among anti-Neisseria meningitidis serogroup C (MenC), anti-Haemophilus influenzae type b (Hib), anti-diphtheria (Dtx), anti-tetanus (Ttx), anti-pertussis toxin (Ptx), anti-filamentous hemagglutinin (FHA), and anti-pertactin (Prn) antibodies.
Seroprevalence of Dtx- and Ttx-specific Ig and Ptx-, FHA-, and Prn-specific IgG in maternal and cord serum samples. GMCs, 95% CIs, and ranges of anti-Dtx, anti-Ttx, anti-Ptx, anti-FHA, and anti-Prn antibodies in maternal and cord serum samples are summarized in table 1. The percentage of placental transfer of anti-Dtx, anti-Ttx, anti-Ptx, anti-FHA, and anti-Prn antibodies was 160%, 168%, 164%, 162%, and 131%, respectively. Individual cord and maternal antibody concentrations to Dtx, Ttx, Ptx, FHA, and Prn are shown in figure 1C-1G. The mean percentage of placental transfer of antibodies elicited by these proteins was 157%. Individual cord-to-maternal ratios are shown in figure 2. Mean ratios, correlations, and P values among maternal and cord serum samples that are specific for anti-Dtx, anti-Ttx, and anti-pertussis antibodies are shown in table 2.
Protective antibody concentrations in maternal and cord serum samples. Protective concentrations of anti-MenC polysaccharide antibodies (⩾2 µg/mL) [21] were seen in 11% of the cord serum samples. However, 80% of a subset of cord serum samples (table 1) revealed a protective MenC SBA level (⩾8). Protective concentrations of anti-Hib polysaccharide antibodies (⩾0.15 µg/mL) [22] were seen in 76% of cord serum samples. Protective concentrations of anti-Dtx (⩾0.01 IU/mL) [23] and anti-Ttx (⩾0.01 IU/mL) [24] were seen in 96% and 97% of cord serum samples, respectively. Protective concentrations for anti-Ptx, anti-FHA, and anti-Prn are not internationally assigned, but when an arbitrary cutoff value of ⩾20 ELISA U/mL was used [25], protective concentrations were seen in 37%, 66%, and 38% of cord serum samples, respectively.
Serum samples obtained from mothers at the time of delivery showed very low concentrations of specific antibodies to MenC polysaccharides; only 10% revealed protective concentrations of antibodies. In contrast, 64% of all mothers had a protective MenC SBA titer. Maternal antibodies directed toward pertussis were at a low concentration; only 20%, 48%, and 32% contained protective concentrations for Ptx, FHA, and Prn, respectively. Maternal antibody concentrations to Hib, Dtx, and Ttx were also low; however, 99% of the maternal serum samples revealed titers above the supposed protective concentration for Dtx and Ttx, and 79% of these serum samples contained protective IgG concentrations of antibodies to Hib polysaccharides.
Discussion
In the present study, we investigated the placental transfer of antibodies and the concentrations of antibodies specific for several vaccine components implemented in our Dutch National Immunization Programme. To our knowledge, this is the first study in which a birth cohort of ∼200 paired samples was used to investigate the placental transfer of maternal antibodies to a variety of vaccine-preventable diseases. We found that, in general, circulating maternal antibody concentrations were low. Active transportation of maternal antibodies, leading to increased concentrations in the infant, is probably restricted to IgG1 antibodies elicited by proteins; cord antibody concentrations increased to 160% of the concentration of maternal antibodies. In contrast, concentrations of polysaccharide-specific antibodies in cord blood were equal to concentrations in maternal serum, indicating that transportation is less effective than for antibodies directed toward proteins and does not result in higher concentrations in the infant. The likely reason for this phenomenon is the less effective transfer of IgG2 antibodies (the main subclass for antipolysaccharide antibodies in adults), compared with the other IgG antibodies.
The concentration of antibodies placentally transferred from mother to infant depends on the type of antigen being either protein or polysaccharide. This was observed for Hib and Ttx in other studies [26, 27]. We found similar antibody concentrations in mother and cord blood not only for MenC and Hib polysaccharides but also for meningococcal serogroups A, W-135, and Y polysaccharides (data not shown). Other studies found that antibody transfer is subclass dependent [8, 26]. The results for the IgG subclasses that are specific for MenC poly-saccharides seem to indicate that active transportation is limited to MenC-specific IgG1 and that IgG2 is placentally transferred to a lesser extent, only attaining the concentrations of maternal IgG2, as was shown elsewhere for Hib [26].
The fact that antiprotein antibodies are more effectively and actively transported across the placenta, compared with antipolysaccharide antibodies, is also revealed by MenC SBA titers. Bactericidal activity is higher in cord blood than in maternal serum; this suggests that such activity not only depends on MenC polysaccharide antibodies, the percentage of which was equal in maternal serum and cord blood, but might also depend on antibodies directed to proteins. These MenC-specific bactericidal antibodies are expected to be elicited by natural exposure to meningococci, also inducing antibody responses toward outer-membrane proteins. Therefore, on the basis of the MenC polysaccharide antibody titer alone, the percentage of protected infants seems to be underestimated. However, bactericidal killing in vivo might be less effective in neonates than in adults [28]. Furthermore, the MenC vaccine is implemented at 14 months of age; therefore, neonates and infants might be at risk before they receive their MenC vaccination, because maternal antibodies will probably not persist for such a long period [29, 30]. This risk is emphasized by the current cases of MenC disease that have sporadically occurred in un-vaccinated age groups after the mass immunization campaign of 2002 [31, 32].
Concentrations of Hib polysaccharide antibodies were found above the estimated protective level in ∼70% of the cord serum samples. These maternally derived antibodies were elicited by natural exposure of the mothers to the pathogen, possibly inducing a wide variety of anti-Hib antibodies. Although the problem of waning maternal antibody is of a less concern for Hib than for MenC, because the first vaccination to prevent Hib disease is given at 2 months of age, future monitoring is required.
Almost all Dtx and Ttx titers in cord serum samples were above the generally excepted protection level of 0.01 IU/mL. Maternal antibody concentrations were probably high because of the 6 vaccinations that are administrated during childhood, with the last routine vaccinations at 9 years of age; re-vaccinations to Ttx and/or Dtx later in life are not exceptional. Dtx and Ttx antibodies remain above protective levels for a long time [33, 34], and with an estimated half-life of 5–6 weeks [29, 30], antibody concentrations would still be well above the protective level in most infants until their primary vaccinations. In addition to the transferred maternal antibodies, the large herd effect should provide sufficient protection against diphtheria during the first 2 months [34].
The observed antibody concentrations specific for pertussis in cord serum samples were low. Based on titers directed against Ptx, FHA, and Prn, only a small proportion of all neonates would probably be protected against pertussis infection, according to the arbitrary cutoff value [35-37]. The higher maternal antibody concentration to FHA might also be caused by cross-reactive antigens. Moreover, considering the scepticism about the protective properties of FHA antibodies, the high number of infants considered to be protected is most likely an overestimation. The few observed high pertussis-specific antibody concentrations in mothers were probably attributable to (recent) natural infection. Nevertheless, for a large majority of the neonates, the overall antibody concentrations are too low to provide sufficient protection until the neonates receive their first vaccination at 2 months. This is of great concern, because the incidence of pertussis is still high and epidemical episodes occur every 3 years in the Netherlands [38]. This is especially threatening for infants who are too young to be (fully) vaccinated [39-41].
A few premature neonates (n=5) were included in our neonatal study group. Their individual antibody titers did not deviate from those of full-term infants, and therefore, the premature neonates were not excluded from our study group. Furthermore, limited demographic data on the mothers were available (e.g., ethnicity). However, our cohort of mother and neonate pairs was large and, therefore, may be representative of the Dutch population as a whole. Demographic statistics from the Dutch statistics center indicate that our study population resembled the overall Dutch birth cohort on the basis of maternal age and sex of the newborn [42].
In conclusion, antibodies directed to polysaccharides, such as MenC and Hib, are transferred less effectively than are antibodies elicited by proteins, such as Dtx, Ttx, and the 3 pertussis antigens. This difference is likely to be subclass dependent, because IgG2 is placentally transported less efficiently, compared with IgG1. In addition, even in cases of effective maternal antibody transfer to the neonate, antibody concentrations in the mothers were low, resulting in low concentrations of maternal antibodies in cord serum samples and leaving the newborns at increased risk in the first months of life before they received primary vaccinations. These data indicate that close clinical and serological surveillance is necessary to prevent MenC and Hib disease and pertussis in neonates and infants. Furthermore, these data support maternal vaccination to prevent neonates from infectious diseases or vaccination of close contacts of the neonate to decrease the reservoir of infectious agents before neonates receive their primary vaccinations.
Acknowledgments
We thank Femke van Nunen and Corine Nellestijn, for technical assistance; Drs. Ger Rijkers, Annemarie Buisman, and Leo Schouls, for useful discussions; and the nurses and secretary from the Department of Obstetrics and Gynaecology at Groene Hart Ziekenhuis.
Financial support. National Institute of Public Health and the Environment, Bilthoven, the Netherlands.
Potential conflicts of interest. All authors: no conflicts.
References
- enzyme-linked immunosorbent assay
- antigens
- communicable diseases
- diphtheria toxin
- gestational age
- hemagglutinins
- immunoassay
- newborn
- maternal age
- mothers
- neisseria meningitidis
- polysaccharides
- tetanus toxin
- toxins
- vaccination
- vaccines
- immunoglobulins
- antibodies
- bordetella pertussis
- diphtheria
- enzymes
- haemophilus influenzae type b
- placenta
- tetanus
- pertussis
- pathogenic organism
- placental transport
- umbilical cord blood
- serum specimen
- transportation
- meningococcal conjugate vaccines
- maternal antibody
- seroprevalence
- serogroup