-
PDF
- Split View
-
Views
-
Cite
Cite
Timothy H. Dellit, Robert C. Owens, John E. McGowan, Dale N. Gerding, Robert A. Weinstein, John P. Burke, W. Charles Huskins, David L. Paterson, Neil O. Fishman, Christopher F. Carpenter, P. J. Brennan, Marianne Billeter, Thomas M. Hooton, Infectious Diseases Society of America and the Society for Healthcare Epidemiology of America Guidelines for Developing an Institutional Program to Enhance Antimicrobial Stewardship, Clinical Infectious Diseases, Volume 44, Issue 2, 15 January 2007, Pages 159–177, https://doi.org/10.1086/510393
- Share Icon Share
Executive Summary
This document presents guidelines for developing institutional programs to enhance antimicrobial stewardship, an activity that includes appropriate selection, dosing, route, and duration of antimicrobial therapy. The multifaceted nature of antimicrobial stewardship has led to collaborative review and support of these recommendations by the following organizations: American Academy of Pediatrics, American Society of Health-System Pharmacists, Infectious Diseases Society for Obstetrics and Gynecology, Pediatric Infectious Diseases Society, Society for Hospital Medicine, and Society of Infectious Diseases Pharmacists. The primary goal of antimicrobial stewardship is to optimize clinical outcomes while minimizing unintended consequences of antimicrobial use, including toxicity, the selection of pathogenic organisms (such as Clostridium difficile), and the emergence of resistance. Thus, the appropriate use of antimicrobials is an essential part of patient safety and deserves careful oversight and guidance. Given the association between antimicrobial use and the selection of resistant pathogens, the frequency of inappropriate antimicrobial use is often used as a surrogate marker for the avoidable impact on antimicrobial resistance. The combination of effective antimicrobial stewardship with a comprehensive infection control program has been shown to limit the emergence and transmission of antimicrobial-resistant bacteria. A secondary goal of antimicrobial stewardship is to reduce health care costs without adversely impacting quality of care.
These guidelines focus on the development of effective hospital-based stewardship programs and do not include specific outpatient recommendations. Although judicious use of antimicrobials is important in outpatient clinics and long-term care facilities, there are very few data regarding effective interventions, and it is unclear which interventions are most responsible for improvement in these settings.
The population targeted by these guidelines includes all patients in acute care hospitals. Most of the evidence supporting the recommendations in these guidelines is derived from studies of interventions to improve antimicrobial use for hospitalized adults. Many of these studies have focused on adults in intensive care units. Only a handful of studies have focused on hospitalized newborns, children, and adolescents. Few studies have included substantial populations of severely immunocompromised patients, such as patients undergoing hematopoetic stem cell transplantation or receiving chemotherapy likely to cause prolonged neutropenia. Nonetheless, the recommendations in these guidelines are likely to be broadly applicable to all hospitalized patients.
The ratings of the practices recommended in this document reflect the likely impact of stewardship practices on improving antimicrobial use and, consequently, minimizing the emergence and spread of antimicrobial resistance. Each recommendation is rated on the basis of the strength of the recommendation and the quality of evidence supporting it, using the rating system of the Infectious Disease Society of America (IDSA), as shown in table 1 [1]. The ratings provided also reflect the likely ability of the recommendation to reduce health care costs. Some strategies to reduce resistance may actually result in an increase in drug acquisition costs as part of a more comprehensive plan to reduce overall costs, including the attributable costs of resistance. In situations in which the likely impact of a recommendation on appropriate use of antimicrobials and health care costs diverge or in which cost data are not available, separate ratings are given.
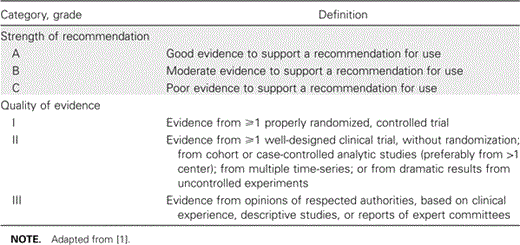
Infectious Diseases Society of America-United States Public Health Service grading system for ranking recommendations in clinical guidelines.
Effective antimicrobial stewardship programs can be financially self-supporting and improve patient care [2–7] (A-II). Comprehensive programs have consistently demonstrated a decrease in antimicrobial use (22%–36%), with annual savings of $200,000–$900,000 in both larger academic hospitals [2, 3, 5, 7, 8] and smaller community hospitals [4, 6]. Thus, health care facilities are encouraged to implement antimicrobial stewardship programs. A comprehensive evidence-based stewardship program to combat antimicrobial resistance includes elements chosen from among the following recommendations based on local antimicrobial use and resistance problems and on available resources that may differ, depending on the size of the institution or clinical setting.
1. Core members of a multidisciplinary antimicrobial stewardship team include an infectious diseases physician and a clinical pharmacist with infectious diseases training (A-II) who should be compensated for their time (A-III), with the inclusion of a clinical microbiologist, an information system specialist, an infection control professional, and hospital epidemiologist being optimal (A-III). Because antimicrobial stewardship, an important component of patient safety, is considered to be a medical staff function, the program is usually directed by an infectious diseases physician or codirected by an infectious diseases physician and a clinical pharmacist with infectious diseases training (A-III).
2. Collaboration between the antimicrobial stewardship team and the hospital infection control and pharmacy and therapeutics committees or their equivalents is essential (A-III).
3. The support and collaboration of hospital administration, medical staff leadership, and local providers in the development and maintenance of antimicrobial stewardship programs is essential (A-III). It is desirable that antimicrobial stewardship programs function under the auspices of quality assurance and patient safety (A-III).
4. The infectious diseases physician and the head of pharmacy, as appropriate, should negotiate with hospital administration to obtain adequate authority, compensation, and expected outcomes for the program (A-III).
5. Hospital administrative support for the necessary infrastructure to measure antimicrobial use and to track use on an ongoing basis is essential (A-III).
6. There are 2 core strategies, both proactive, that provide the foundation for an antimicrobial stewardship program. These strategies are not mutually exclusive.
A. Prospective audit with intervention and feedback. Prospective audit of antimicrobial use with direct interaction and feedback to the prescriber, performed by either an infectious diseases physician or a clinical pharmacist with infectious diseases training, can result in reduced inappropriate use of antimicrobials (A-I).
B. Formulary restriction and preauthorization. Formulary restriction and preauthorization requirements can lead to immediate and significant reductions in antimicrobial use and cost (A-II) and may be beneficial as part of a multifaceted response to a nosocomial outbreak of infection (B-II). The use of preauthorization requirements as a means of controlling antimicrobial resistance is less clear, because a long-term beneficial impact on resistance has not been established, and in some circumstances, use may simply shift to an alternative agent with resulting increased resistance (B-II). In institutions that use preauthorization to limit the use of selected antimicrobials, monitoring overall trends in antimicrobial use is necessary to assess and respond to such shifts in use (B-III).
7. The following elements may be considered and prioritized as supplements to the core active antimicrobial stewardship strategies based on local practice patterns and resources.
A. Education. Education is considered to be an essential element of any program designed to influence prescribing behavior and can provide a foundation of knowledge that will enhance and increase the acceptance of stewardship strategies (A-III). However, education alone, without incorporation of active intervention, is only marginally effective in changing antimicrobial prescribing practices and has not demonstrated a sustained impact (B-II).
B. Guidelines and clinical pathways. Multidisciplinary development of evidence-based practice guidelines incorporating local microbiology and resistance patterns can improve antimicrobial utilization (A-I). Guideline implementation can be facilitated through provider education and feedback on antimicrobial use and patient outcomes (A-III).
C. Antimicrobial cycling. There are insufficient data to recommend the routine use of antimicrobial cycling as a means of preventing or reducing antimicrobial resistance over a prolonged period of time (C-II). Substituting one antimicrobial for another may transiently decrease selection pressure and reduce resistance to the restricted agent. Unless the resistance determinant has been eliminated from the bacterial population, however, reintroduction of the original antimicrobial is again likely to select for the expression of the resistance determinant in the exposed bacterial population.
D. Antimicrobial order forms. Antimicrobial order forms can be an effective component of antimicrobial stewardship (B-II) and can facilitate implementation of practice guidelines.
E. Combination therapy. There are insufficient data to recommend the routine use of combination therapy to prevent the emergence of resistance (C-II). Combination therapy does have a role in certain clinical contexts, including use for empirical therapy for critically ill patients at risk of infection with multidrug-resistant pathogens, to increase the breadth of coverage and the likelihood of adequate initial therapy (A-II).
F. Streamlining or de-escalation of therapy. Streamlining or de-escalation of empirical antimicrobial therapy on the basis of culture results and elimination of redundant combination therapy can more effectively target the causative pathogen, resulting in decreased antimicrobial exposure and substantial cost savings (A-II).
G. Dose optimization. Optimization of antimicrobial dosing based on individual patient characteristics, causative organism, site of infection, and pharmacokinetic and pharmacodynamic characteristics of the drug is an important part of antimicrobial stewardship (A-II).
H. Parenteral to oral conversion. A systematic plan for parenteral to oral conversion of antimicrobials with excellent bioavailability, when the patient's condition allows, can decrease the length of hospital stay and health care costs (A-I). Development of clinical criteria and guidelines allowing switch to use of oral agents can facilitate implementation at the institutional level (A-III).
8. Health care information technology in the form of electronic medical records (A-III), computer physician order entry (B-II), and clinical decision support (B-II) can improve antimicrobial decisions through the incorporation of data on patient-specific microbiology cultures and susceptibilities, hepatic and renal function, drug-drug interactions, allergies, and cost. However, implementation of these features has been slow, and conformation of the technology to the clinical environment remains a challenge.
9. Computer-based surveillance can facilitate good stewardship by more efficient targeting of antimicrobial interventions, tracking of antimicrobial resistance patterns, and identification of nosocomial infections and adverse drug events (B-II).
10. The clinical microbiology laboratory plays a critical role in antimicrobial stewardship by providing patient-specific culture and susceptibility data to optimize individual antimicrobial management and by assisting infection control efforts in the surveillance of resistant organisms and in the molecular epidemiologic investigation of outbreaks (A-III).
11. Both process measures (did the intervention result in the desired change in antimicrobial use?) and outcome measures (did the process implemented reduce or prevent resistance or other unintended consequences of antimicrobial use?) are useful in determining the impact of antimicrobial stewardship on antimicrobial use and resistance patterns (B-III).
Introduction
Purpose. In recognition that antimicrobial resistance results in increased morbidity, mortality, and cost of health care, the IDSA initially published guidelines for improving the use of antimicrobial agents in hospitals in 1988 [9] and then jointly published guidelines with the Society for Healthcare Epidemiology of America in 1997 for the prevention of antimicrobial resistance in hospitals [10]. However, subsequent surveys of hospitals have found that practices to improve antimicrobial use are frequently inadequate and not routinely implemented [11–13]. The purpose of these guidelines is to build on the previous position statements, as well as to provide evidence-based recommendations for developing a program to enhance antimicrobial stewardship in the hospital setting to improve the quality of care. These guidelines are not a substitute for clinical judgment, and clinical discretion is required in the application of guidelines to individual patients.
Effective antimicrobial stewardship programs, also known as antimicrobial management programs, can be financially self-supporting and can improve patient care [2–7] (A-II). Antimicrobial stewardship includes not only limiting inappropriate use but also optimizing antimicrobial selection, dosing, route, and duration of therapy to maximize clinical cure or prevention of infection while limiting the unintended consequences, such as the emergence of resistance, adverse drug events, and cost. Given the emergence of multidrug-resistant pathogens and their impact on clinical care, appropriate use of antimicrobial agents has become a focus of patient safety and quality assurance along with medication errors, allergy identification, and drug-drug interactions [14]. The ultimate goal of antimicrobial stewardship is to improve patient care and health care outcomes.
From the institutional perspective, antimicrobials account for upwards of 30% of hospital pharmacy budgets [15]. It has been recognized for several decades that up to 50% of antimicrobial use is inappropriate, adding considerable cost to patient care [8, 9, 15–18]. In addition to direct pharmacy acquisition costs, numerous reports suggest that inappropriate and unnecessary antimicrobial use leads to increased selection of resistant pathogens (table 2). Once antimicrobial resistance emerges, it can have a significant impact on patient morbidity and mortality, as well as increased health care costs [32, 33]. Bacteremia [34, 35] and surgical site infections [36] due to methicillin-resistant Staphylococcus aureus (MRSA) have been associated with a higher mortality rate than similar infections due to methicillin-susceptible S. aureus, with the mean attributable cost of an MRSA infection ranging from $9275 to $13,901 [36, 37]. Similarly, compared with vancomycin-susceptible Enterococcus faecium infections, bloodstream infections due to vancomycin-resistant E. faecium (VRE) were associated with decreased survival (24% vs. 59%), increased length of hospital stay (34.8 vs. 16.7 days), and an attributable cost of $27,190 per episode [38, 39]. A meta-analysis of 9 studies of VRE bloodstream infections found an attributable excess mortality of 30%, compared with vancomycin-susceptible Enterococcus bloodstream infections [40]. Similar adverse outcomes have also been reported for infections with resistant gram-negative organisms, including Pseudomonas, Acinetobacter, and Enterobacter species and extended-spectrum β-lactamase-producing organisms [41]. A case-control study found that third-generation cephalosporin-resistant Enterobacter infections were associated with increased mortality (relative risk, 5.02), length of hospital stay (1.5-fold increase), and an attributable cost of $29,379 [42]. The emergence of infections with multidrug-resistant gram-negative organisms, combined with a paucity of new drug development, has unfortunately led to the resurgent use of colistin, a polymyxin antimicrobial previously abandoned because of its high rates of nephrotoxicity and neurotoxicity [43]. In 1998, the Institute of Medicine estimated that the annual cost of infections caused by antimicrobial-resistant bacteria was $4–$5 billion [44].
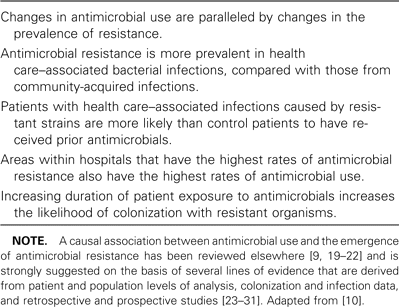
Causal associations between antimicrobial use and the emergence of antimicrobial resistance.
Methods. The recommendations in this guideline are based on a review of published studies identified through a search of the PubMed database (search terms used alone and in combination included “antimicrobial,” “antibiotic,” “stewardship,” “management,” “resistance,” “cost,” “education,” “guidelines,” “restriction,” “cycling,” “order forms,” and “combination therapy”) supplemented by review of references of relevant articles to identify additional reports. Committee members were also asked to cite additional relevant studies to support the recommendations. Because of the limited number of randomized, controlled trials, results from prospective cohort studies, case-control studies, longitudinal time series, and other descriptive studies are included in the review. The ratings of the practices recommended in this document reflect the likely impact of such practices on improving antimicrobial use and, ultimately, antimicrobial resistance. Given the association between antimicrobial use and the selection of resistant pathogens, rates of inappropriate antimicrobial use are considered as surrogate markers for the avoidable impact on antimicrobial resistance.
The strength of the recommendations and quality of evidence are rated using IDSA criteria (table 1) [1]. Individual studies were evaluated both for their impact on the targeted antimicrobial(s) or resistance problem and for any secondary impact on local antimicrobial use and resistance patterns. The ratings also reflect the likely ability of the recommendation to reduce health care costs. In situations in which the likely impact of a recommendation on appropriate use of antimicrobials and health care costs diverge or cost data are not available, separate ratings are given. Recommendations reflect a compilation of the studies in each section, as well as the opinions of the committee members.
Guidelines For Developing An Institutional Program To Enhance Antimicrobial Stewardship
The Antimicrobial Stewardship Team And Administrative Support
It is essential that the antimicrobial stewardship team includes an infectious diseases physician and a clinical pharmacist with infectious diseases training and that both of these individuals are compensated appropriately for their time. Optimally, the team should include a clinical microbiologist who can provide surveillance data on antimicrobial resistance, as well as an information system specialist who can provide the computer support necessary for surveillance and implementation of recommendations. In addition, it is optimal that the team includes an infection control professional and hospital epidemiologist to coordinate efforts on improving antimicrobial use, because reduction of antimicrobial resistance is a common goal of these persons. Because antimicrobial stewardship, an important component of patient safety, is considered to be a medical staff function, the program is usually directed by an infectious diseases physician or codirected by an infectious diseases physician and a clinical pharmacist with infectious diseases training. The clinical pharmacist should be knowledgeable on the appropriate use of antimicrobials, and appropriate training should be made available to achieve and maintain this expertise. It is essential that there be support and collaboration between the antimicrobial stewardship team and the hospital infection control and pharmacy and therapeutics committees or their equivalents.
The support and collaboration of hospital administration, medical staff leadership, and local providers in the development and maintenance of antimicrobial stewardship programs is essential to success of the program. In this regard, the infectious diseases physician and the head of pharmacy, as appropriate, should negotiate with hospital administration to obtain adequate authority, compensation, and expected outcomes for the program (A-III). It is essential that there be hospital administrative support for the necessary infrastructure, to measure antimicrobial use and to track use on an ongoing basis (A-III). It is desirable that antimicrobial stewardship programs function under the auspices of quality assurance and patient safety. Prior to program implementation, the antimicrobial stewardship strategic plan should be presented to and approved by the chiefs of professional services, hospital medical staff executive committee, and/or other medical staff governing bodies, to ensure their acceptance and support.
Recommendations
Core members of a multidisciplinary antimicrobial stewardship team include an infectious diseases physician and a clinical pharmacist with infectious diseases training (A-II) who should be compensated for their time (A-III), with the inclusion of a clinical microbiologist, an information system specialist, an infection control professional, and hospital epidemiologist being optimal (A-III). Because antimicrobial stewardship, an important component of patient safety, is considered to be a medical staff function, the program is usually directed by an infectious diseases physician or codirected by an infectious diseases physician and a clinical pharmacist with infectious diseases training (A-III).
Collaboration between the antimicrobial stewardship team and the hospital infection control and pharmacy and therapeutics committees, or their equivalents, is essential (A-III).
The support and collaboration of hospital administration, medical staff leadership, and local providers in the development and maintenance of antimicrobial stewardship programs is essential (A-III). It is desirable that antimicrobial stewardship programs function under the auspices of quality assurance and patient safety (A-III).
The infectious diseases physician and the head of pharmacy, as appropriate, should negotiate with hospital administration to obtain adequate authority, compensation, and expected outcomes for the program (A-III).
Hospital administrative support for the necessary infrastructure to measure antimicrobial use and to track use on an ongoing basis is essential (A-III).
Elements Of An Antimicrobial Stewardship Program
The best strategies for the prevention and containment of antimicrobial resistance are not definitively established, because there is a paucity of randomized, controlled trials in this field [45]. Often, multiple interventions have been made simultaneously, making it difficult to assess the benefit attributable to any one specific intervention. However, a comprehensive program that includes active monitoring of resistance, fostering of appropriate antimicrobial use, and collaboration with an effective infection control program to minimize secondary spread of resistance [46, 47] is considered to be optimal [48]. A comprehensive evidence-based stewardship program to combat antimicrobial resistance includes elements chosen from among the following strategies, which are based on local antimicrobial use and resistance problems, and on available resources that may differ depending on the size of the institution or clinical setting.
Active Antimicrobial Stewardship Strategies
Prospective audit with intervention and feedback. Prospective audit of antimicrobial use with intervention and feedback to the prescriber have been demonstrated to improve antimicrobial use. In a large teaching hospital, house staff were randomized by the medical service to receive either no intervention or one-on-one education by a clinical specialist (academic detailing) on a patient-specific basis, emphasizing microbiologic data, local resistance patterns, and clinical literature, when the pharmacy received an order for either levofloxacin or ceftazidime. This resulted in a 37% reduction in the number of days of unnecessary levofloxacin or ceftazidime use by decreasing the duration of therapy, as well as reducing new starts, suggesting that house staff learned not to initiate unnecessary antibiotic treatment regimens [49]. At a 600-bed tertiary teaching hospital, inpatients receiving parenteral antimicrobials chosen by their primary care physician were randomized to an intervention group that received antimicrobial-related suggestions from an infectious diseases fellow and a clinical pharmacist versus no antimicrobial suggestions. Physicians in the intervention group received 74 suggestions for 62 of 127 patients, including suggestions on a more appropriate agent, route of administration, dosing, discontinuation of the drug, or toxicity monitoring. Eighty-five percent of the suggestions were implemented, resulting in 1.6 fewer days of parenteral therapy and a cost savings of $400 per patient, with no adverse impact on clinical response, compared with the control group [50]. There was a trend, however, toward increasing rates of readmission in the intervention group, emphasizing the need to monitor the impact of such interventions designed to decrease length of hospital stay.
Prospective audit and interventions by a clinical pharmacist and infectious diseases physician at a medium-sized community hospital resulted in a 22% decrease in the use of parenteral broad-spectrum antimicrobials, despite a 15% increase in patient acuity over a 7-year period [3]. They also demonstrated a decrease in rates of C. difficile infection and nosocomial infection caused by drug-resistant Enterobacteriaceae, compared with the preintervention period.
In hospitals where daily review of antimicrobial use is not feasible because of limited resources, a scaled-down model can still have a significant impact, as illustrated by a small, 120-bed community hospital that used an infectious diseases physician and clinical pharmacist 3 days per week to review patients receiving multiple, prolonged, or high-cost courses of antimicrobial therapy [4]. Sixty-nine percent of 488 recommendations were implemented, resulting in a 19% reduction in antimicrobial expenditures for an estimated annual savings of $177,000, compared with the preintervention period. In these studies, interventions were communicated to prescribers either verbally or in writing. Written communication was typically accomplished by using special, nonpermanent forms that were placed in the medical record or chart but were subsequently removed after the intervention or at the time of discharge from the hospital. Each intervention provides the opportunity for provider education.
Effective audit with intervention and feedback can be facilitated through computer surveillance of antimicrobial use, allowing the targeting of specific services or units where problems exist, as well as identification of patients receiving particular agents or combinations of agents that might benefit from intervention.
Recommendation
Prospective audit of antimicrobial use with direct interaction and feedback to the prescriber, performed by either an infectious diseases physician or a clinical pharmacist with infectious diseases training, can result in reduced inappropriate use of antimicrobials (A-I).
Formulary restriction and preauthorization requirements for specific agents. Most hospitals have a pharmacy and therapeutics committee or an equivalent group that evaluates drugs for inclusion on the hospital formulary on the basis of considerations of therapeutic efficacy, toxicity, and cost while limiting redundant new agents with no significant additional benefit. Antimicrobial restriction—either through formulary limitation by this method or by the requirement of preauthorization and justification—is the most effective method of achieving the process goal of controlling antimicrobial use. Longitudinal studies implementing restrictive policies have demonstrated significant initial decreases in the use of the targeted antimicrobials, with annual antimicrobial cost savings ranging upwards of $800,000 [14, 51–57]. The achievement of the outcome goal of reducing antimicrobial resistance has not been as clear, as illustrated by the following studies.
Both formulary restriction [58] and preauthorization requirements for use of clindamycin [59] during nosocomial epidemics of C. difficile infection have led to prompt cessation of the outbreaks, whereas preapproval restriction of broad-spectrum antimicrobials has led to short-term increased susceptibilities among gram-negative pathogens, such as Pseudomonas aeruginosa, Klebsiella pneumoniae, and Enterobacter cloacae, during a 6–12-month period [57, 60]. Restriction of vancomycin and third-generation cephalosporins in response to increasing rates of VRE has demonstrated mixed results [61–63]. Fecal VRE colonization rates of 47% (despite barrier precautions) led one center to restrict vancomycin and cefotaxime use while encouraging the replacement of third-generation cephalosporins with β-lactam/β-lactamase inhibitor combinations. This led to a reduction in the rates of monthly use of vancomycin, cefotaxime, and ceftazidime by 34%, 84%, and 55%, respectively, and rates of ampicillin-sulbactam and piperacillin-tazobactam use increased. This was accompanied by a decrease in the fecal VRE point prevalence from 47% to 15% during 6 months [59]. In contrast, in another study, the prevalence of VRE increased from 17% to 30%, despite the restriction on the use of vancomycin and third-generation cephalosporins during a 10-year period [64]. The interpretation of these study results is often confounded by concomitant changes in infection control practices and by the influence of nonrestricted antimicrobial agents on gut flora.
Studies of antibiotic-restriction policies among pediatric patients have demonstrated inconsistent results. A crossover study of 2 neonatal intensive care units (ICUs) compared 2 approaches for empirical treatment of early- and late-onset suspected sepsis—a “broad-spectrum” regimen consisting of ampicillin and cefotaxime versus a “narrow-spectrum” regimen consisting of penicillin and tobramycin—on the prevalence of colonization with bacteria resistant to each of the regimens [65]. The narrow-spectrum regimen was associated with a markedly lower prevalence of colonization with resistant gram-negative bacilli. In contrast, a quasi experimental study from a pediatric ICU of a policy to restrict ceftazidime use (piperacillin-tazobactam was the preferred regimen) found no change in the incidence of colonization with ceftazidime-resistant gram-negative bacilli, although there was a decrease in the prevalence of colonization with specific species of gram-negative bacilli that commonly harbor inducible AmpC β-lactamases (e.g., E. cloacae, Serratia marcesens, Citrobacter freundii, and P. aeruginosa) [66].
The effectiveness of a preauthorization program depends on who is making the recommendations. Restriction of cefotaxime use through a program requiring approval from a chief resident or attending physician had no impact on its use [67]. Recommendations from an antimicrobial management team consisting of a pharmacist and an infectious diseases physician resulted in increased antimicrobial appropriateness, increased clinical cure, and a trend towards improved economic outcome, compared with recommendations made by infectious diseases fellows [68].
The challenge of antimicrobial restriction and its effect on antimicrobial resistance is exemplified in a study by Rahal et al. [27]. In response to an increasing incidence of cephalosporin-resistant Klebsiella, a preapproval policy was implemented for cephalosporins. This resulted in an 80% reduction in hospital-wide cephalosporin use and a subsequent 44% reduction in the incidence of ceftazidime-resistant Klebsiella throughout the medical center, as well as a 71% reduction in the ICUs. Concomitantly, however, imipenem use increased 141%, accompanied by a 69% increase in the incidence of imipenem-resistant P. aeruginosa. This untoward restrictive effect of “squeezing the balloon” may counteract the originally sought benefits [69]. Furthermore, restricting use of a single drug to prevent or reverse antimicrobial resistance may be ineffective, because multiple antimicrobials may be associated with changes in susceptibility to other drugs for a given pathogen [70].
Recommendation
Formulary restriction and preauthorization requirements can lead to immediate and significant reductions in antimicrobial use and cost (A-II) and may be beneficial as part of a multifaceted response to a nosocomial outbreak of infection (B-II). The use of preauthorization requirements as a means of controlling antimicrobial resistance is less clear, because a long-term beneficial impact on resistance has not been established, and in some circumstances, use may simply shift to an alternative agent with resulting increased resistance (B-II). In institutions that use preauthorization to limit the use of selected antimicrobials, monitoring overall trends in antimicrobial use is necessary to assess and respond to such shifts in use (B-III).
Supplemental Antimicrobial Stewardship Strategies
Education. Education is the most frequently employed intervention and is considered to be an essential element of any program designed to influence prescribing behavior. Educational efforts include passive activities, such as conference presentations, student and house staff teaching sessions, and provision of written guidelines or e-mail alerts. However, education alone, without incorporation of active intervention, is only marginally effective and has not demonstrated a sustained impact [71–73].
Step-wise implementation of an antimicrobial stewardship program initially with passive strategies, such as education and order forms, followed by an active strategy with prospective audit and intervention demonstrated progressive decreases in antimicrobial consumption, resulting in a savings of $913,236 over 18 months. During the period of active intervention, 25% of antimicrobial orders were modified (86% resulted in less expensive therapy, and 47% resulted in use of a drug with a narrower spectrum of activity), resulting in a significant increase in microbiologically based prescribing (63% vs. 27%) [71].
In an attempt to improve adherence to recommendations for perioperative antimicrobial prophylaxis, a before-and-after study compared prescribing practices after distribution of an educational handbook with those after the introduction of an order form. The educational handbook led to a marginal improvement in compliance (from 11% to 18%), whereas introduction of the order form led to significantly improved compliance (from 17% to 78%) [73].
Recommendation
Education is considered to be an essential element of any program designed to influence prescribing behavior and can provide a foundation of knowledge that will enhance and increase the acceptance of stewardship strategies (A-III). However, education alone, without incorporation of active intervention, is only marginally effective in changing antimicrobial prescribing practices and has not demonstrated a sustained impact (B-II).
Guidelines and clinical pathways. Clinical practice guidelines are being produced with increasing frequency, with the goal of ensuring high-quality care. However, the impact on provider behavior and improved clinical outcomes has been difficult to measure. Although physicians usually agree, in principle, with national guidelines, the absence of accompanying strategies for local implementation often presents a formidable barrier [74]. Antimicrobial stewardship programs can facilitate multidisciplinary development of evidence-based practice guidelines that incorporate local microbiology and resistance patterns.
Randomized implementation of a clinical pathway, compared with conventional management of community-acquired pneumonia, among 20 hospitals led to a 1.7-day decrease in the median length of hospital stay, an 18% decrease in the rate of admissions of low-risk patients, and 1.7 fewer mean days of intravenous therapy in the intervention group, without an increase in complications, readmissions, or mortality [75]. In another study, multidisciplinary development of practice guidelines based on evidence in the literature and local microbiology and resistance patterns and implementation in a surgical ICU led to a 77% reduction in antimicrobial use and cost, a 30% reduction in overall cost of care, decreased mortality among patients with infection, and a trend towards reduced length of ICU stay, compared with the preimplementation time period [76]. Importantly, both of these studies demonstrate that antimicrobial selection is only 1 component in improving the management of infectious diseases and cannot be done without recommendations for diagnosis and testing, admission criteria, nursing care, conversion to oral medication, and discharge planning. Whether the use of guidelines will lead to a long-term impact on antimicrobial resistance remains to be determined, but the following studies of hospital-acquired pneumonia (HAP) and ventilator-associated pneumonia (VAP) suggest that improving antimicrobial use through the use of guidelines may decrease the emergence of resistant pathogens.
The increasing incidence of multidrug-resistant organisms in cases of HAP and VAP, the diagnostic challenge of these entities, and the mortality benefit associated with initial appropriate therapy [77] have led to an increased use of broad-spectrum antimicrobials, which must be balanced against further selection of resistant pathogens. Invasive diagnosis of VAP with quantitative bronchoscopy for diagnosis and antimicrobial guidance led to reduced mortality at 14 days and a decrease in antimicrobial use [78]. Another strategy to address the inappropriate use of antimicrobials in the ICU setting used an algorithm incorporating the clinical pulmonary infection score to identify patients with a low likelihood of pneumonia. Patients randomized to the intervention group who continued to have a low clinical pulmonary infection score (⩽6) had their antimicrobial therapy discontinued at day 3, and the control group received the standard 10–21 days of therapy. This led to a significant decrease in duration of therapy (3 vs. 9.8 days) and antimicrobial cost ($400 per patient), with no difference in mortality. In addition, the development of antimicrobial resistance and/or superinfections was less common in the group receiving the short-course antimicrobial therapy (15% vs. 35%) [79]. A prospective before-and-after study of a clinical guideline for the management of VAP incorporated broad empirical therapy based on local microbiology with culture-driven de-escalation and a standard 7-day course of therapy. Implementation of the protocol led to increased initial administration of adequate antimicrobial therapy (94% vs. 48%), decreased duration of therapy (8.6 vs. 14.8 days), and decreased VAP recurrence (8% vs. 24%), without affecting patient mortality [80]. The efficacy of short-course therapy for VAP was subsequently confirmed in a randomized study of 8 versus 15 days of antimicrobial therapy in patients with VAP documented by quantitative culture of samples obtained by bronchoscopy. There was no difference in mortality or recurrent infection in patients who received the shorter course of therapy, but the short-course group did have more antimicrobial-free days (13.1 vs. 8.7) and a decreased rate of emergence of multidrug-resistant pathogens among those patients with recurrences of pulmonary infection (42% vs. 62%) [81]. These studies support the development and implementation of evidence-based guidelines for diagnosis and antimicrobial therapy for HAP and VAP.
A quasi experimental study in a pediatric hospital in Australia demonstrated similar results regarding the use of guidelines to improve therapy for common infections [82]. The investigators provided recommendations for the treatment of childhood infections on a laminated card that could be clipped to a hospital badge. When the 6-month period during implementation of the intervention (intervention period) was compared with the prior 6 months (baseline period), the intervention was associated with substantial increases in the percentage of prescriptions with the correct choice and dose of antimicrobial agents for 2 of 3 indicator infections (pneumonia and orbital/periorbital cellulitis). The cost of third-generation cephalosporins was reduced by more than one-half in the intervention period.
Recommendation
Multidisciplinary development of evidence-based practice guidelines incorporating local microbiology and resistance patterns can improve antimicrobial utilization (A-I). Guideline implementation can be facilitated through provider education and feedback on antimicrobial use and patient outcomes (A-III).
Antimicrobial cycling and scheduled antimicrobial switch. “Antimicrobial cycling” refers to the scheduled removal and substitution of a specific antimicrobial or antimicrobial class to prevent or reverse the development of antimicrobial resistance within an institution or specific unit. In true cycling, there is a return to the original antimicrobial after a defined time, as opposed to a simple switch of antimicrobials [83–86]. In many respects, cycling is an attempt at controlled heterogeneity of antimicrobial use to minimize antimicrobial selection pressures. Studies of true antimicrobial cycling are limited and vary in terms of antimicrobial class selection, duration of cycling, therapeutic options offered during cycling periods, and cycling by time period versus by patient. Concerns about allergies, adverse drug events, and conflicts with national guidelines have led to 10%–50% of patients in cycling programs to receive “off-cycle” antimicrobials, resulting in poor implementation of the intended process change, with multiple antimicrobials being used at the same time by different patients [85].
Driven by both increasing resistance among Enterobacteriaceae and pricing changes, the largest cycling experience has been reported for changes in aminoglycoside use—particularly, substituting amikacin for gentamicin. Such a switch in aminoglycoside use has been associated with a significant reduction in gentamicin resistance [87–93]; however, rapid reintroduction of gentamicin was accompanied by a rapid return of gentamicin resistance [89, 92]. In one institution with 10 years of experience, this led to an additional cycle of amikacin followed by a more gradual return of gentamicin, without an associated increase in resistance once the original gentamicin resistance plasmids could no longer be detected [92]. This last example highlights the importance of understanding and monitoring mechanisms of resistance over the long term when developing protocols for antimicrobial cycling. Once antimicrobial resistance emerges, it will often persist even in the absence of direct antimicrobial selection pressure, potentially minimizing the impact of antimicrobial removal strategies [21].
A switch from the empirical use of ceftazidime to ciprofloxacin for suspected gram-negative bacterial infection in a cardiothoracic ICU led to a decreased incidence of VAP due to multidrug-resistant, gram-negative bacteria (1% vs. 4%) [94]. Restriction of ceftazidime and ciprofloxacin in a medical ICU, combined with cycling of the preferred β-lactam agent at monthly intervals, led to a decreased incidence of VAP and improved susceptibilities for P. aeruginosa. Because these maneuvers, as well as de-escalation of therapy based on culture results, led to a 50% reduction in overall antimicrobial use, the benefit of cycling alone cannot be ascertained [95]. Quarterly rotation of empirical antimicrobial regimens in a surgical ICU for pneumonia and peritonitis/sepsis led to a decreased incidence of resistant bacterial infections and mortality due to infection [96]. However, significant patient population differences and the simultaneous changes in infection control, including institution of an antibiotic surveillance team and the introduction of alcohol gel dispensers, confounded interpretation of the results. In addition, only 62%–83% of patients received the “on-cycle” antimicrobial intended in the process change, resulting in antimicrobial mixing as opposed to time period-based cycling.
It should be noted that mathematical modeling suggests that true cycling is unlikely to reduce the evolution or spread of antimicrobial resistance. Rather, such modeling suggests that the simultaneous mixed use of different antimicrobial classes in a heterogeneous fashion may slow the spread of resistance [97, 98].
In an attempt to examine this hypothesis, a prospective crossover study compared the effects of monthly cycling of antipseudomonal agents (cefepime or ceftazidime, ciprofloxacin, imipenem or meropenem, or piperacillin-tazobactam) with the use of these agents in the same order by consecutive patients (i.e., mixing) [99]. During mixing, a significantly higher proportion of patients acquired a strain of P. aeruginosa that was resistant to cefepime (9% vs. 3%; P = .01). As in previous cycling studies, however, adherence to the cycling regimen was problematic, with scheduled antimicrobials never accounting for more than 45% of all antipseudomonal antimicrobials. Additional clinical studies to examine optimal cycling parameters and the role of antimicrobial diversity are needed.
Recommendation
There are insufficient data to recommend the routine use of antimicrobial cycling as a means of preventing or reducing antimicrobial resistance over a prolonged period of time (C-II). Substituting one antimicrobial for another may transiently decrease selection pressure and reduce resistance to the restricted agent. Unless the resistance determinant has been eliminated from the bacterial population, however, reintroduction of the original antimicrobial is again likely to select for the expression of the resistance determinant in the exposed bacterial population.
Antimicrobial order forms. Antimicrobial order forms decrease antimicrobial consumption in longitudinal studies through the use of automatic stop orders and the requirement of physician justification [100, 101]. Prior to more recent studies further defining the optimal timing and duration of perioperative antimicrobial prophylaxis [102, 103], use of perioperative prophylactic order forms with automatic discontinuation at 2 days resulted in a decrease in the mean duration of antimicrobial prophylaxis (from 4.9 to 2.4 days) and a decrease in the percentage of patients receiving perioperative prophylaxis for >2 days (from 85% to 44%) [100]. The rate of inappropriate initiation of antimicrobial prophylaxis postoperatively decreased from 30% to 11% with use of the order form. The use of an order form for all inpatient antimicrobial orders in an 800-bed hospital that required clinical indication, as well as a defined duration before order renewal, led to a 30% decrease in antibiotic courses and a 2% decrease in the hospital pharmacy budget for parenteral antibiotics over a 25-month period, during which time most hospitals were experiencing an increase in expenditures [101]. Use of an antibiotic order form for vancomycin did not improve appropriate use of vancomycin in a pediatric hospital [104]. Automatic stop orders should not replace clinical judgment, and renewal requirements must be clearly communicated to providers to avoid inappropriate treatment interruptions.
Recommendation
Antimicrobial order forms can be an effective component of antimicrobial stewardship (B-II) and can facilitate implementation of practice guidelines.
Combination therapy: prevention of resistance versus redundant antimicrobial coverage. The rationale for combination antimicrobial therapy includes broad-spectrum empirical therapy for serious infections, improved clinical outcomes, and the prevention of resistance. Inadequate initial antimicrobial therapy was found to be an independent risk factor for mortality in nonurinary infections due to extended-spectrum β-lactamase-producing Escherichia coli and Klebsiella species [105]. Similarly, inadequate early antimicrobial coverage has been associated with increased mortality in patients with microbiologically confirmed severe sepsis (39% vs. 24%) [106] and critically ill ICU patients (42% vs. 18%) [55], leading to incorporation of empirical combination therapy for late-onset VAP in the recent IDSA-American Thoracic Society guidelines [107]. These studies highlight the need to assess risk factors for multidrug-resistant pathogens when selecting empirical antimicrobial therapy for critically ill patients.
However, in many situations, combination therapy is redundant and unnecessary. Evidence supporting the role of combination antimicrobial therapy for the prevention of resistance is limited to those situations in which there is a high organism load combined with a high frequency of mutational resistance during therapy. Classic examples are tuberculosis or HIV infection. There is often debate about the role of combination therapy in serious infections due to gram-negative organisms, such as Pseudomonas species, but clear evidence supporting a clinical benefit or resistance benefit is lacking [108–118]. A meta-analysis of randomized, controlled trials comparing a β-lactam plus an aminoglycoside as combination therapy with β-lactam monotherapy for the treatment of hospitalized patients with serious infections found no difference in the emergence of antimicrobial resistance. In fact, β-lactam monotherapy was associated with fewer superinfections [119].
Recommendation
There are insufficient data to recommend the routine use of combination therapy to prevent the emergence of resistance (C-II). Combination therapy does have a role in certain clinical contexts, including use for empirical therapy for critically ill patients at risk of infection with multidrug-resistant pathogens, to increase the breadth of coverage and the likelihood of adequate initial therapy (A-II).
Streamlining or de-escalation of therapy. Good stewardship to optimize empirical initial antimicrobial therapy may conflict with good stewardship to promote judicious use, because continuing excessively broad therapy contributes to the selection of antimicrobial resistant pathogens [120]. This conflict can be resolved when culture results become available by streamlining or de-escalating antimicrobial therapy to more targeted therapy that decreases antimicrobial exposure and contains cost. De-escalation may also include discontinuation of empirical antimicrobial therapy based on clinical criteria and negative culture results as demonstrated in the management of suspected VAP [79, 107, 121]. Review by a pharmacist and an infectious diseases physician of 625 patients receiving combination antimicrobial therapy led to streamlining recommendations in 54% of antimicrobial courses over 7 months, resulting in a projected annual savings of $107,637 [122].
In another study, a computer query to mine the hospital pharmacy database followed by targeted review by an infectious diseases clinical pharmacist facilitated the identification of potentially redundant antimicrobial combinations in 16% of patients receiving ⩾2 antimicrobials. Even after accepting the debatable “double gram-negative coverage,” 71% of the combinations were deemed to be inappropriate. Interestingly, half of the redundancy was due to physician prescribing error, whereas the other half was due to medication ordering and distribution system errors. The annualized potential savings from this intervention was estimated to be $60,000, and ∼3500 redundant inpatient antibiotic-days were avoided [123].
Recommendation
Streamlining or de-escalation of empirical antimicrobial therapy on the basis of culture results and elimination of redundant combination therapy can more effectively target the causative pathogen, resulting in decreased antimicrobial exposure and substantial cost savings (A-II).
Dose optimization. Optimization of antimicrobial dosing that accounts for individual patient characteristics (e.g., age, renal function, and weight), causative organism and site of infection (e.g., endocarditis, meningitis, and osteomyelitis), and pharmacokinetic and pharmacodynamic characteristics of the drug is an important part of antimicrobial stewardship. For instance, the bactericidal activity of β-lactams correlates with the percentage of time that the drug concentration remains greater than the MIC, whereas fluoroquinolones and aminoglycosides are concentration-dependent agents, with the ratio of the maximum concentration to the MIC or the ratio of the area under the curve to the MIC being important predictors of activity. Examples of these principles in practice include prolonged or continuous infusion of β-lactams [124], extended-interval dosing of aminoglycosides [125], and dosing of fluoroquinolones for Streptococcus pneumoniae in community-acquired pneumonia [126, 127] and for Pseudomonas in HAP and VAP [107]. The use of pharmacokinetic and pharmacodynamic principles is more likely to be in development of antimicrobial use guidelines than in individual patients' care.
Recommendation
Optimization of antimicrobial dosing based on individual patient characteristics, causative organism, site of infection, and pharmacokinetic and pharmacodynamic characteristics of the drug is an important part of antimicrobial stewardship (A-II).
Conversion from parenteral to oral therapy. Antimicrobial therapy for patients with serious infections requiring hospitalization is generally initiated with parenteral therapy. Enhanced oral bioavailability among certain antimicrobials—such as fluoroquinolones, oxazolidinones, metronidazole, clindamycin, trimethoprim-sulfamethoxazole, fluconazole, and voriconazole—allows conversion to oral therapy once a patient meets defined clinical criteria. This can result in reduced length of hospital stay, health care costs, and potential complications due to intravenous access.
Randomized studies evaluating early transition from parenteral to oral therapy in the management of adults with community-acquired pneumonia have demonstrated significant reductions in length of hospital stay and cost of care with no adverse effect on clinical outcomes [128–130]. A similar decrease in length of hospital stay, with a 52% reduction in total health care costs, was noted in the treatment of lower respiratory tract infections in children, compared with historical control subjects [131]. A pharmacist-initiated program utilizing predetermined clinical criteria for general conversion from parenteral to oral therapy decreased length of hospital stay by 1.53 days, with cost savings for drug acquisition and reduced length of hospital stay of $15,149 and $161,072, respectively, over 12 months [132].
A randomized study of oral linezolid versus intravenous vancomycin in patients with complicated skin and soft-tissue infections due to MRSA demonstrated a decreased mean length of hospital stay of 5 days for the linezolid group [133], and a switch from vancomycin to oral linezolid for early discharge from the hospital resulted in an annual savings of $294,750 [134]. The use of new agents, such as linezolid, in this manner must be done judiciously and with the direct oversight of an antimicrobial-management program to balance concerns about the development of resistance and added antimicrobial acquisition costs.
A systematic plan for switching from parenteral to oral treatment may have an added benefit of aiding in early hospital discharge planning, if needed, to provide surge capacity during local or national problems (e.g., epidemic influenza).
Recommendation
A systematic plan for parenteral to oral conversion of antimicrobials with excellent bioavailability, when the patient's condition allows, can decrease length of hospital stay and health care costs (A-I). Development of clinical criteria and guidelines allowing conversion to use of oral agents can facilitate implementation at the institutional level (A-III).
Computer Surveillance and Decision Support
Increased focus on medical errors and patient safety led to a series of reports by the Institute of Medicine's National Roundtable on Health Care Quality to emphasize the role of information technology in the delivery of health care [135–137]. The Leapfrog Group has identified computer physician order entry (CPOE) as 1 of the 3 most important “leaps” that organizations can take to substantially improve patient safety. CPOE has the potential to incorporate clinical decision support and to facilitate quality monitoring [138]. Progress to this end, however, remains slow, with only 13% of US hospitals converting to electronic medical records and 5% implementing CPOE as of 2002 [139, 140].
The most well-described computer surveillance and decision-support system related to antimicrobial prescribing linked to electronic medical records is from LDS Hospital in Salt Lake City, Utah [141]. This program presents epidemiologic information with detailed recommendations and warnings regarding antimicrobial regimens and courses of therapy. Even if a physician overrides the recommendation for the antimicrobial and selects his or her own treatment plan, the computer still automatically reviews the patient's allergies and potential drug-drug interactions, recommending a dosage and interval based on the patient's renal and hepatic function. A prospective study of the use of this program in an ICU demonstrated significant reductions in orders for drugs to which the patients had reported allergies, excess drug dosages based on renal function, adverse drug events, antimicrobial-susceptibility mismatches, antimicrobial costs, and length of hospital stay [142]. Implementation of a computer-assisted antibiotic-dose monitor throughout the same hospital over a 12-month period identified 1974 (44%) of 4483 patients receiving excessive antimicrobial dosages (based on renal function), leading to more-appropriate dosing and fewer adverse drug events [143]. Incorporation of practice guidelines into the system increased the percentage of surgical patients who received their preoperative prophylactic antimicrobials within 2 h of incision from 40% to 99.1% [144, 145].
In addition to improving antimicrobial use and care of the individual patient, their system has facilitated the electronic surveillance of hospital-acquired infections and adverse drug events. Computer surveillance identified 90% of confirmed nosocomial infections, compared with 76% of such infections identified by manual surveillance, allowing infection control practitioners to reduce the time required for such activities by 65% [146]. Automated surveillance of 36,653 patients over 18 months using defined triggers identified 731 adverse drug events, whereas only 9 were reported through traditional voluntary incident reports [147].
This computer decision-support system was adapted for use in pediatric patients by Mullett et al. [148], and its effect was evaluated in a quasi experimental study. Dosing guidelines were adjusted for pediatric and neonatal populations, to ensure that treatment recommendations were appropriate for infections common in the pediatric population (e.g., bacterial meningitis), local antimicrobial resistance patterns (e.g., the prevalence of S. pneumoniae with reduced susceptibility to penicillin), special populations (e.g., children with cystic fibrosis), and children with renal insufficiency. Comparing a 6-month period after implementation (intervention period) with the prior 6 months (baseline period), the decision-support system was associated with a 59% decrease in the rate of pharmacy interventions for erroneous drug doses and 36% and 28% decreases in the rates of subtherapeutic and excessive antimicrobial dosing days, respectively. There was a 9% decrease in the cost of antimicrobial agents during the intervention period. The frequency of adverse drug events and antimicrobial-bacterial susceptibility mismatches were not significantly different during the intervention period—a finding likely attributable to the low frequency of these events in the baseline period. Clinicians using the system reported that they felt that the program improved their selection of antimicrobial agents, increased their awareness of impairments in renal function that affected drug dosing, and reduced the likelihood of adverse drug events. The lead investigator has subsequently developed a separate decision-support system for treatment of bloodstream infection in hospitalized children, although the system has not yet been evaluated prospectively [149].
A randomized study incorporating guidelines for vancomycin use into a hospital's CPOE at the time of initial ordering and after 72 h of therapy led to 32% fewer vancomycin orders and a 36% reduction in the duration of vancomycin therapy. This resulted in a projected savings of $90,000 [150]. Simply adding antimicrobial cost information to antimicrobial susceptibility data resulted in decreased average monthly antimicrobial expenditures by $7636 (17%) in another hospital [151].
Despite these initial promising studies, matching the linear technology of CPOE with complex clinical management that can be subjective, interpretive, and reactive has been a challenge at other institutions [152]. Implementation of CPOE at a 750-bed teaching hospital to reduce medical errors was actually found to frequently facilitate medication errors [140]. Errors included inappropriate dose selection, double-dosing caused by separate order and discontinuation functions, and gaps in antimicrobial therapy resulting from automatic discontinuation orders. In large part, these errors reflected the difficulty of implementation rather than the concept of CPOE.
The Veterans Administration health care system has been a leader in the use of an electronic medical records and CPOE. Despite being a model for implementing CPOE, one Veterans Administration hospital found a continuing high rate of adverse drug events in the absence of decision support for drug selection, dosing, and monitoring [153]. Twenty-six percent of hospital admissions were associated with at least 1 adverse drug event, with medication errors contributing to 27% of these adverse events.
The lofty goal of merging the electronic records with CPOE and clinical decision support to optimize antimicrobial use is currently not attainable for most institutions on the basis of current technology. Depending on available resources, however, automated targeting of interventions to facilitate antimicrobial stewardship can be obtained through varying levels of complexity. Such targeting may include using pharmacy records to identify patients who are receiving broad-spectrum or expensive antimicrobials, use of simple computer programs that merge hospital pharmacy and microbiology databases, and use of more-complex, commercially available software to identify antimicrobial interventions.
Recommendations
Health care information technology in the form of electronic medical records (A-III), CPOE (B-II), and clinical decision support (B-II) can improve antimicrobial decisions through the incorporation of data on patient-specific microbiology cultures and susceptibilities, hepatic and renal function, drug-drug interactions, allergies, and cost. However, implementation of these features has been slow, and conformation of the technology to the clinical environment remains a challenge.
Computer-based surveillance can facilitate good stewardship by more efficient targeting of antimicrobial interventions, tracking of antimicrobial resistance patterns, and identification of nosocomial infections and adverse drug events (B-II).
Microbiology Laboratory
The clinical microbiology laboratory plays a critical role in the timely identification of microbial pathogens and the performance of susceptibility testing [154, 155]. Susceptibility testing and reporting should be based on the guidelines developed by the Clinical and Laboratory Standards Institute (CLSI; formerly the NCCLS) [156]. Prioritization of tested antimicrobials and selective reporting of susceptibility profiles (e.g., not routinely reporting susceptibility of S. aureus to rifampin to prevent inadvertent monotherapy with rifampin) can aid in the prudent use of antimicrobials and direct appropriate therapy based on local guidelines. The advance of molecular diagnostics allows the identification of difficult-to-culture pathogens, potentially avoiding the need for extended courses of broad-spectrum empirical therapy.
In addition to routine susceptibility testing, the clinical microbiology laboratory should be actively involved in resistance surveillance. Local antibiograms with pathogen-specific susceptibility data should be updated at least annually, to optimize expert-based recommendations for empirical therapy [157]. Computerized surveillance can facilitate more-frequent monitoring of antimicrobial resistance trends, as well as provide ICU- or ward-specific data and inpatient versus outpatient data, recognizing that different parts of a health care institution can have very different patterns of antimicrobial use and resistance [157]. Besides qualitative determination of antimicrobial resistance or susceptibility, periodic review of MICs or zone diameters in disk-diffusion techniques can detect early trends of emerging resistance, even within the “susceptibility” cut-offs. Kirby-Bauer disk-diffusion methods can also be used to perform the D test for inducible clindamycin resistance for S. aureus [158], as well as provide quick screenings for extended-spectrum β-lactamase- and AmpC β-lactamase-containing organisms. Finally, the laboratory is an important partner with infection control in the identification and molecular epidemiologic investigation of local outbreaks of infection. The development of rapid resistance testing will facilitate the surveillance of organisms such as MRSA and VRE, allowing the more rapid implementation of infection control measures to prevent secondary spread [159, 160]. Clonal characterization of resistant strains through molecular typing can help focus appropriate interventions, leading to a reduction in nosocomial infections with associated cost savings [161]. If antimicrobial resistance is due to a clonal outbreak, antimicrobial interventions may be of limited value, compared with infection control interventions. If resistant strains are diverse, antimicrobial interventions may be required.
Recommendation
The clinical microbiology laboratory plays a critical role in antimicrobial stewardship by providing patient-specific culture and susceptibility data to optimize individual antimicrobial management and by assisting infection control efforts in the surveillance of resistant organisms and in the molecular epidemiologic investigation of outbreaks (A-III).
Monitoring of Process and Outcome Measurements
In conjunction with developing local strategies for improving antimicrobial stewardship, programs must establish process and outcome measures to determine the impact of antimicrobial stewardship on antimicrobial use and resistance patterns. Furthermore, health care systems must invest in data systems to allow the evaluation of antimicrobial stewardship as a routine measure of quality improvement [162]. With antimicrobial stewardship, the “process goal” is often to change use of a specific antimicrobial or class of antimicrobials. The related “process measure” for this goal would determine the degree to which the intervention to change the use of an antimicrobial or class of antimicrobials has been successfully implemented, compared with baseline levels. The desired “outcome goal” of these process changes is to reduce or prevent resistance or other unintended consequences of antimicrobial use. “Outcome measurements” define the degree to which these outcomes are achieved, such as reduced antimicrobial resistance, adverse drug events, and cost, as well as unintended consequences, such as rates of C. difficile infection and the use of nontargeted antimicrobials as a result of the process change.
Antimicrobial use data based on pharmacy expenditure or dispensing reports often do not account for drug wastage, unused doses returned to pharmacy, or fluctuations in institutional price structures and discounts [163]. Drug use data can be standardized using the defined daily dose, calculated as the total number of grams of an antimicrobial agent used divided by the number of grams in an average adult daily dose of the agent [164]. The World Health Organization publishes defined daily dose values for nearly all antimicrobials (http://www.whocc.no/atcddd/). The use of defined daily doses is recommended so that hospitals may compare their antimicrobial use with that of other similar hospitals, recognizing the challenges of interhospital comparisons and the potential need for “risk adjustment.” However, in populations with renal compromise (e.g., the elderly population) and for drugs that require renal dose adjustment, the defined daily dose may be less accurate than measures of antimicrobial-days of therapy [165].
Recommendation
Both process measures (did the intervention result in the desired change in antimicrobial use?) and outcome measures (did the process implemented reduce or prevent resistance or other unintended consequences of antimicrobial use?) are useful in determining the impact of antimicrobial stewardship on antimicrobial use and resistance patterns (B-III).
Comprehensive Multidisciplinary Antimicrobial Management Programs
Through the previous review of individual interventions directed at improving antimicrobial use, it is clear that effective antimicrobial stewardship requires a multidisciplinary team approach that incorporates many of these elements simultaneously. The core members of a comprehensive antimicrobial management program include an infectious diseases physician and a clinical pharmacist with infectious diseases training, with the inclusion of infection control professionals, the hospital epidemiologist, a clinical microbiologist, and an information system specialist, when possible [166–174]. The latter is critical for linking the patient's medical record to the pharmacy and microbiology databases, to identify interventions and to perform surveillance activities. Program personnel should be included as active members on the hospital infection control and pharmacy and therapeutics committees or their equivalents.
Central to an effective program is a proactive strategy incorporating prospective audit with direct intervention and feedback to the provider and/or preauthorization requirements for antimicrobial use. On the basis of an understanding of local antimicrobial use and resistance problems and of available resources that may differ depending on the size of the institution, the core active strategies may be supplemented by education, guidelines and clinical pathways, antimicrobial order forms, adequate empirical therapy followed by de-escalation based on culture results, dose optimization, and a systematic plan for conversion from parenteral to oral therapy. Consensus building with the support of administration and local providers is essential, with the focus on collaborating in the safety and care of their patients rather than a policing role. Although reports describing the clinical and economic impacts of multidisciplinary antimicrobial management programs are limited to single-center longitudinal studies, they consistently demonstrate a decrease in antimicrobial use (22%–36%) and annual savings of $200,000–$900,000, which more than pays for the program in both larger academic hospitals [2, 3, 5, 7, 8, 69] and smaller community hospitals [4, 6]. Quantifying a long-term impact on antimicrobial resistance has been more challenging, and further studies are needed to determine the optimal processes by which the goals of improved clinical outcomes and containment of antimicrobial resistance can be achieved. However, given the strong association between antimicrobial use and antimicrobial resistance (table 2), improving antimicrobial stewardship is an important first step.
Research Priorities And Future Directions
Because of the limited number of randomized clinical studies addressing antimicrobial stewardship strategies, many of the recommendations in this guideline are based on level III evidence. Further research and evaluation through appropriately conducted clinical trials are necessary to determine the best strategies for the prevention and containment of antimicrobial resistance. Recommended topics for investigation are as follows:
1. Antimicrobial cycling at the patient, unit, and institutional level to determine whether cycling is effective and, if so, the optimal antimicrobials to be cycled, the optimal duration of the cycles, and the preferred order in which agents should be cycled.
2. Clinical validation of mathematical models suggesting that heterogeneous antimicrobial use slows the spread of resistance.
3. The long-term impact of formulary restriction and preauthorization requirements on antimicrobial use and resistance.
4. Evaluation of “bundled” approaches that incorporate many or all of the most effective strategies.
5. Examination of the effectiveness of these strategies in more detail in subpopulations of hospitalized patients, including neonates, infants, and children; elderly patients; and severely immunocompromised patients.
6. The ability of antimicrobials to cause “collateral damage” or unintended ecological resistance, to focus interventions.
7. The incremental role of antimicrobial stewardship combined with infection control practices, such as hand hygiene and isolation, designed to prevent secondary spread of resistant organisms.
8. Understanding the resistance gene pool through molecular epidemiology, to determine the relative impact of antimicrobial stewardship and infection control practices on specific resistant bacteria, to tailor an approach to local resistance issues.
9. Development and validation of automated surveillance strategies for nosocomial infections and real-time monitoring of resistance trends.
10. Development of decision-support systems incorporating antimicrobial stewardship into CPOE.
11. Development and cost-effectiveness of more rapid and sensitive diagnostic tests, to identify patients with bacterial versus viral infections and to identify resistant bacterial organisms earlier.
12. Strategies to stimulate research and development of novel antimicrobials as outlined in the IDSA “Bad Bugs, No Drugs” campaign.
13. Education and training of infectious diseases fellows and pharmacists in the area of antimicrobial stewardship, including program implementation and management.
14. The influence of pharmaceutical industry and representatives on antimicrobial prescribing within the health care setting and effective strategies to counteract inappropriate detailing.
acknowledgments
We wish to express our gratitude to Ronald E. Polk, Christopher A. Ohl, and Joseph F. John, Jr., for their thoughtful reviews of this guideline.
Potential conflicts of interest. J.E.M. has received research support for Project ICARE from AstraZeneca, bioMérieux, Elan, Pfizer, and 3M Health Care and has served as a consultant for Cubist, Dade Microscan, Merck, Replidyne, and Wyeth. D.N.G. has patents licensed to ViroPharma; has received research grants from Genzyme, Massachusetts Biological Laboratories, and ViroPharma; and has served as consultant for AstraZeneca, Genzyme, Optimer, Romark, GOJO, Salix, and ViroPharma. W.C.H. has received research support from Elan and Merck and has served as consultant to Roche Diagnostics. D.L.P. has received research grants from AstraZeneca, Elan, Merck, and Pfizer and has served as a consultant or on speakers' bureaus for Merck, Cubist, Pfizer, Elan, and Genzyme. M.B. has served on speakers' bureaus for Merck and Pfizer. All other authors: no conflicts.
references
These guidelines were developed and issued on behalf of the Infectious Diseases Society of America and the Society for Healthcare Epidemiology of America.