-
PDF
- Split View
-
Views
-
Cite
Cite
H Hackstein, A Kalina, B Dorn, I S Keil, N Baal, G Michel, C Brendel, A Neubauer, T Jakob, G Bein, CD11c+ dendritic cells mediate antigen-specific suppression in extracorporeal photopheresis, Clinical and Experimental Immunology, Volume 203, Issue 2, February 2021, Pages 329–339, https://doi.org/10.1111/cei.13539
- Share Icon Share
Summary
Extracorporeal photopheresis (ECP) represents one of the most widespread and effective cell therapies for graft-versus-host disease and other T cell-mediated disorders. However, the key factors affecting the therapeutic efficacy of ECP remain unclear. We hypothesized that therapeutic effects are mediated by ECP-treated antigen-presenting dendritic cells (DC). To test this hypothesis, we used the experimental model of contact hypersensitivity (CHS). The ECP's therapeutic activity improved when the total cell dose of the ECP-treated cells was increased. We used different haptens during sensitization to demonstrate that the anti-inflammatory activity of ECP is antigen-specific. This confirmed the hypothesis that professional antigen-presenting cells are involved in the mode of action. Also, the ECP's therapeutic activity was abrogated by the depletion of CD11c+ DC, which represents fewer than 1% of all the ECP-exposed cells. Finally, we confirm the critical importance of CD11c+ DC for ECP activity by showing that only a few purified CD11c+ DC are sufficient to mediate its therapeutic effect. The finding that ECP-treated, physiological antigen-presenting DC alone mediate antigen-specific modulation of a pathological immune response may result in better-targeted interventions when treating patients.
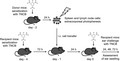
The mechanism of action of extracorporeal photopheresis (ECP) is largely unknown. Here, we demonstrate, using a preclinical in vivo model, that the antigen-specific immunosuppressive effect of ECP is mediated solely by CD11c+ dendritic cells.
Introduction
Extracorporeal photopheresis (ECP) is an immunomodulatory cellular therapy used successfully to treat graft-versus-host disease (GVHD) and other T cell-mediated disorders [1]. Briefly, ECP uses apheresis to collect mononucleated leukocytes that are then incubated with 8-methoxypsoralen (8-MOP), irradiated with ultraviolet A light (UVA) and reinfused to the patient. Initially, ECP was developed for the treatment of cutaneous T cell lymphoma (CTCL) and the response of 73% of patients with otherwise resistant lymphoma was attributed to an immune reaction to the infused damaged cells [2]. Clinical indications include GVHD [3–7], heart transplant rejection [8–10] and bronchiolitis obliterans syndrome in lung transplant patients [11,12]. Initially, the therapeutic effects were attributed to the induction of apoptosis in ECP-treated cells [13]. Recently, we demonstrated that ECP promotes interleukin (IL)-1β production in monocytes and myeloid dendritic cells (DC), indicating that ECP-induced cell death of antigen-presenting cells (APC) is not silent [14].
Interestingly, many clinical studies demonstrated the effectiveness of ECP as per a wide range of T cell-mediated inflammatory diseases and cutaneous and non-cutaneous T cell lymphomas [1,2,15]. A recent consensus report came to the conclusion that ECP delivers two immunotherapeutic vectors: an anti-cancer immunizing mode and a tolerogenic mode [16]. Differentiation of monocytes to DC by the interaction of monocytes with platelets under flow conditions (unexposed to 8-MOP and UVA) and feeding of the resulting DC with ECP-treated tumor cells demonstrated anti-cancer immunizing effects [17]. In contrast, tolerizing effects may be mediated by DC, present in the inoculum, that are exposed to 8-MOP and UVA irradiation [16]. However, the clinical utilization of ECP for antigen-specific immunosuppression is limited due to the rarity of randomized clinical trials and lack of exact knowledge on its mechanism of action [18].
Maeda et al. [19] and Hequet et al. [20] demonstrated that ECP-treated DC partially prevented the sensitization step of contact hypersensitivity (CHS) in antigen-specific preclinical models. Prevention of the challenge stage of CHS by ECP-treated DC has not been demonstrated. Considering that ECP is mainly indicated in the therapeutic setting, rather than in the prophylactic setting, we hypothesized that ECP-treated antigen-presenting DC prevent the challenge stage in a model of CHS. The identification of the critical leukocyte subset and the key parameters for the therapeutic efficacy of ECP could pave the way for further improvement and adaptation of ECP in new clinical indications.
Materials and methods
Mice and reagents: leukocyte phenotyping
Mice, reagents and details of leukocyte phenotyping are presented in the Supporting information.
Tissue preparation
For single-cell suspension, spleen and lymph nodes (axillary, cervical and inguinal lymph nodes) were incubated with digestion buffer (RPMI-1640 with 10% heat-inactivated mouse serum, 8·8 U/ml DNase I and 0·153 U/ml collagenase A) for 15 min at 37°C, mashed through a 70-μm cell strainer and washed twice with RPMI-1640 after erythrocyte lysis at 500 × g for 5 min at 4°C.
Contact hypersensitivity
The mice were sensitized by painting 100 µl of a 3% 2-chloro-1,3,5-trinitrobenzene (TNCB) solution [in acetone/olive oil (AOO) 3 : 1] on the shaved abdominal skin. Four days after sensitization, the mice were challenged by applying a 1% TNCB solution (in acetone) on both ears. As a measure of the degree of CHS, mean ear thickness immediately before, and 24, 48 and 72 h after the challenge was assessed using a micrometer (Käfer Messuhrenfabrik GmbH, Villingen-Schwenningen, Germany). The intensity of CHS was expressed as delta ear thickness in µm.
Experimental ECP
The donor mice were sensitized with TNCB or carrier or 4-ethoxymethylene-2-phenyl-2-oxazoline-5-one (OXA) 24 h before harvest of spleen and lymph nodes. Single-cell suspensions of splenocytes and lymph node cells were exposed to UVA light (2, 4 or 6 J/cm2) in an irradiation chamber (BIO-Link BLX-365; Vilber Lourmat, Eberhardzell, Germany) in the presence of 8-MOP (200 ng/ml). The UVA dose was controlled independently using a calibrated UVA dosimeter (RM-21; Dr Gröbel UV-Elektronik GmbH, Ettlingen, Germany). For this purpose, 5 × 106 cells/ml were taken up in RPMI-1640 with 10% heat-inactivated mouse serum. For irradiation, a volume of 30 ml of cell suspension was transferred into a 100-mm tissue culture dish (Sarstedt, Nümbrecht, Germany) or, in the case of sorted cells, into a 100-mm glass dish (Duran Wheaton Kimble Life Science GmbH, Wertheim, Germany). Corresponding 60-mm dishes were used for smaller volumes. After washing with 0·9% sterile NaCl solution (Braun, Melsungen, Germany), we injected a volume of 100–200 µl cell suspension containing different cell doses per treatment into the tail vein of syngeneic recipient mice. The recipient mice were sensitized with TNCB 3 days before treatment. The ECP-treated mice were challenged by painting the ears with a TNCB solution 24 h later. The experimental protocol is shown in Fig. 1.
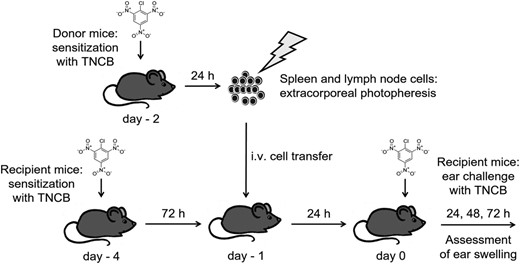
Scheme of experimentalin-vivomodel for contact hypersensitivity (CHS) and extracorporeal photopheresis (ECP). The spleen and lymph node cells were obtained from 2-chloro-1,3,5-trinitrobenzene (TNCB)-sensitized donor mice and irradiated with ultraviolet A (UVA) light in the presence of 8-methoxypsoralen (8-MOP) (extracorporeal photopheresis). The ECP-treated cells were injected intravenously (i.v.) into recipient animals which had been TNCB-sensitized 3 days previously. Twenty-four h after cell transfer, the sensitized mice were challenged by application of TNCB on both ears. As a measure of the degree of CHS, mean ear thickness immediately before and 24, 48 and 72 h after challenge was assessed.
Enrichment and depletion of dendritic cells
The spleen and lymph node cells were incubated with microbeads coated with anti-CD11c and anti-mPDCA-1 antibody (PAN DC MicroBeads Mouse, Miltenyi Biotec, Bergisch Gladbach, Germany). The cells were separated via immunomagnetic cell sorting using the magnetic-activated cell sorting (MACS) system with LS Columns (Miltenyi Biotec). The flow-through fraction was washed with Hanks's balanced salt solution (HBSS) and adjusted to 5 × 106 cells/ml. The eluted fraction was further enriched by fluorescence-activated cell sorting (FACS) using a BD FACSAria III cell sorter (Becton Dickinson Biosciences, San Jose, CA, USA). To this end, the cells were Fc-blocked with heat-inactivated mouse serum and subsequently stained for surface markers with fluorochrome-conjugated monoclonal antibodies to CD45 (30-F11; Biolegend, San Diego, USA), CD3ε (145-2C11; BD Pharmingen, San Diego, USA), CD19 (6D5; Biolegend), GR-1 (RB6-8C5; Biolegend), CD11b (M1-70; Biolegend) and CD11c (N418; Biolegend). The CD11c-positive cells were enriched by FACS. After washing with HBSS, the CD11c+/mPDCA-1+-depleted and the CD11c+-enriched fractions were then ECP-treated as described above. The purity of the CD11c+-depleted and CD11c+-enriched fraction was determined by FACS analysis (BD FACSAria III; Becton Dickinson Biosciences). To determine the dendritic cell content of the enriched fraction, CD11c+/major histocompatibility complex (MHC) class II+ (M5/114.15.2; Biolegend) cells were counted.
Ethics
All the animal studies comply with the ARRIVE (Animal Research: Reporting of In Vivo Experiments) guidelines and were approved by the regional animal authority board (GI 20/8 no. 39/2017).
Statistical analysis
The analyses were performed using GraphPad Prism software version 8.2.1 (GraphPad, San Diego, CA, USA). The figures show mean values and standard error of the mean (s.e.m.). The differences between the experimental groups were analyzed with a one-way analysis of variance (anova) test, Tukey's post-test or Kruskal–Wallis test. A P-value < 0·05 was considered significant.
The data sets generated during and/or analysed during the current study are available from the corresponding author on reasonable request.
Results
The immunomodulatory activity of ECP is UVA dose-dependent
As different ECP treatment protocols are using different UVA doses, we questioned whether the therapeutic efficacy of ECP is UVA dose-dependent. Results show that 2 J/cm2 UVA-irradiated cells were most effective at inhibiting ear-swelling up to 3 days after the TNCB challenge. Conversely, higher UVA doses were less effective, and infusion of non-irradiated 8-MOP exposed cells from sensitized animals was unable to modulate CHS (Supporting information, Fig. S1). Accordingly, 2 J/cm2 UVA was used in further experiments.
The therapeutic effect of ECP is cell dose-dependent
A major clinical question is whether the clinical ECP response can be positively affected by increasing the number of UVA-irradiated cells [21]. To investigate this question, the TNCB-sensitized animals were treated identically but received different doses of ECP-treated donor cells (3 × 106, 10 × 106, 30 × 106 cells). Twenty-four h after cell transfer, ear challenge was performed and ear-swelling was measured on days 1 and 2 after challenge. While the therapeutic effect was much weaker with a lower cell concentration, recipients of 30 × 106 cells had a significantly decreased ear-swelling (P < 0·0001; Fig. 2). These experiments indicated that the total number of ECP-treated cells is a positive predictor of ECP efficacy and higher cell doses are more efficient in this model.
![The therapeutic effect of extracorporeal photopheresis (ECP) depends upon the cell concentration. The spleen and lymph node cells were obtained from 2-chloro-1,3,5-trinitrobenzene (TNCB)-sensitized mice and exposed extracorporeally to 8-methoxypsoralen (8-MOP) plus ultraviolet A (UVA) (2 J/cm2) radiation. The cell number was adjusted to three different concentrations (3, 10, 30 × 106per injection dose). The ECP-treated cells were injected intravenously (i.v.) into recipients which had been sensitized with TNCB 3 days previously. Twenty-four h after cell transfer, recipients were ear-challenged by the application of TNCB. Ear-swelling was measured on days 1 and 2 after challenge. The positive control mice were TNCB-sensitized and TNCB-challenged without ECP treatment. The ear-swelling response is expressed as the difference [µm, mean ± standard error of the mean (s.e.m.)] between thickness of ears before and after challenge. Positive control:n = 7; 30 × 106:n = 7; 10 × 106:n = 8; 3 × 106:n = 8; two independent experiments; statistical tests: one-way analysis of variance (anova) test, Tukey's post-test; *P < 0·05, ***P < 0·001, ****P < 0·0001.](https://oup.silverchair-cdn.com/oup/backfile/Content_public/Journal/cei/203/2/10.1111_cei.13539/4/m_cei13539-fig-0002.jpeg?Expires=1748251889&Signature=vUN~crNSUBrshWkADfjdWHWEKObgyOWnMAsW7IlUv2GjGXL~FwFIk~Q5A8Nz2ecQ7oalbdgG~Q9FGYfrqVdysKHcyuQ8rKUCJiKDuWkigTc78wQ2Nla8ByvodxkCIzfLghZG0t1QTCWL-JraiHZHMSVPY6EmQsqkAlak~rMQx05bOvyyk1xBR1CF8HJNdrqZbo4QaU0iktKXv4gwXvh4TkX9bla~OxSny9u~OWJHkOd9AAFiaEaPqfuu~gp9KDLhSAnbYrOF1PFj9F2mmzProRdqlL569Fhd9hog8SAOUCfABcoSIVBeN7DngPrVWK6a7Gk1h0TXlPg0ucRIDRikLQ__&Key-Pair-Id=APKAIE5G5CRDK6RD3PGA)
The therapeutic effect of extracorporeal photopheresis (ECP) depends upon the cell concentration. The spleen and lymph node cells were obtained from 2-chloro-1,3,5-trinitrobenzene (TNCB)-sensitized mice and exposed extracorporeally to 8-methoxypsoralen (8-MOP) plus ultraviolet A (UVA) (2 J/cm2) radiation. The cell number was adjusted to three different concentrations (3, 10, 30 × 106per injection dose). The ECP-treated cells were injected intravenously (i.v.) into recipients which had been sensitized with TNCB 3 days previously. Twenty-four h after cell transfer, recipients were ear-challenged by the application of TNCB. Ear-swelling was measured on days 1 and 2 after challenge. The positive control mice were TNCB-sensitized and TNCB-challenged without ECP treatment. The ear-swelling response is expressed as the difference [µm, mean ± standard error of the mean (s.e.m.)] between thickness of ears before and after challenge. Positive control:n = 7; 30 × 106:n = 7; 10 × 106:n = 8; 3 × 106:n = 8; two independent experiments; statistical tests: one-way analysis of variance (anova) test, Tukey's post-test; *P < 0·05, ***P < 0·001, ****P < 0·0001.
The therapeutic effect of ECP is antigen-specific
As per the mechanism of action of ECP, we analyzed the antigen-specificity of ECP. The ECP-treated cells were obtained from different donor groups sensitized with TNCB or OXA. Also, a control donor group was mock-sensitized with the AOO. After ECP treatment, the cells were transferred into the TNCB-sensitized recipients and modulation of ear-swelling was analyzed. Results show that only the TNCB-exposed donor cells markedly inhibited ear-swelling after ECP treatment in the TNCB-sensitized recipients (Fig 3; P < 0·001 at day 1). Conversely, neither the OXA-exposed nor vehicle-only exposed donor cells were effective in the TNCB-sensitized recipients after ECP treatment, confirming the hapten specificity of ECP (Fig. 3).
![The therapeutic effect of extracorporeal photopheresis (ECP) is antigen-specific. The spleen and lymph node cells were obtained from the mice that were sensitized with 2-chloro-1,3,5-trinitrobenzene (TNCB) or oxazolone (OXA). Also, the donor mice were treated with the acetone/olive oil (AOO) vehicle as a control. Twenty-four h after transfer of 30 × 106ECP-treated cells [ultraviolet A (UVA) dose 2 J/cm2], the recipients were ear-challenged by the application of TNCB. As a positive control, the mice were sensitized with TNCB and were ear-challenged with the same hapten without ECP treatment. Ear swelling was measured on days 1 and 2 after challenge. The ear-swelling response is expressed as the difference [µm, mean ± standard error of the mean (s.e.m.)] between thickness of ears before and after challenge. Positive control TNCB:n = 9; AOO:n = 7; OXA:n = 8; TNCB:n = 8; two independent experiments; statistical test: Kruskal–Wallis test; Tukey's post-test; **P < 0·01, ***P < 0·001.](https://oup.silverchair-cdn.com/oup/backfile/Content_public/Journal/cei/203/2/10.1111_cei.13539/4/m_cei13539-fig-0003.jpeg?Expires=1748251889&Signature=mcJrsK4hGa6Mra1DENqiDGhQoFbK8kO7d-JSHqlcXI-2d5RxsGTM49cLz9sNcbUQtBAT9I-GkVr8i336usIR1jNQ63SYw3wzAYiVZxu8abFNs8sCroRM1P6G2MM-HPKI8r2JL8Qge7ccG9KqhdhoBZn5Nymeeks3bHHcIeBfsjlqIlRYq95gUqtVfpQzKuIVOtuIBNukqkfJUeHEsYb64cBPZKOiKbxzSf13m8SakwJpm~-ZRHwFy4oWGavsLXpIz38crQ~fU2CuZc6MO7e31BCRA256FD7WiLp3MagSa~8DFIqz~4z-YAQBDbF4FssV9e5xYoUwlqyI-VbctK4vVQ__&Key-Pair-Id=APKAIE5G5CRDK6RD3PGA)
The therapeutic effect of extracorporeal photopheresis (ECP) is antigen-specific. The spleen and lymph node cells were obtained from the mice that were sensitized with 2-chloro-1,3,5-trinitrobenzene (TNCB) or oxazolone (OXA). Also, the donor mice were treated with the acetone/olive oil (AOO) vehicle as a control. Twenty-four h after transfer of 30 × 106ECP-treated cells [ultraviolet A (UVA) dose 2 J/cm2], the recipients were ear-challenged by the application of TNCB. As a positive control, the mice were sensitized with TNCB and were ear-challenged with the same hapten without ECP treatment. Ear swelling was measured on days 1 and 2 after challenge. The ear-swelling response is expressed as the difference [µm, mean ± standard error of the mean (s.e.m.)] between thickness of ears before and after challenge. Positive control TNCB:n = 9; AOO:n = 7; OXA:n = 8; TNCB:n = 8; two independent experiments; statistical test: Kruskal–Wallis test; Tukey's post-test; **P < 0·01, ***P < 0·001.
CD11c+ dendritic cells are critical for the therapeutic efficacy of ECP
As CD11c+ dendritic cells represent key APC modulating innate and adaptive immunity, we investigated their relevance in ECP by preparing additional cell fractions after cell sorting. The ECP cell donors were TNCB-sensitized 24 h before harvesting of splenocytes and lymph node cells. The TNCB-sensitized recipient mice received 30 × 106 ECP-treated cells intravenously (i.v.) that were either CD11c+-depleted or undepleted. Regarding CD45+GR1−CD11b−CD3−CD19− cells, fewer than 1% stained positive for CD11c/MHC class II cells after immunomagnetic depletion (Supporting information, Fig. S2). The results showed that the CD11c+-depleted ECP cells lose their therapeutic effect, indicating that the CD11c+ cells are relevant for the response after ECP treatment (Fig. 4). However, as the loss of therapeutic activity after selective cell depletion is not formal evidence for the positive therapeutic activity of a certain cell type, we included additional experiments with purified CD11c+ cells; 95% of sorted cells were CD11c+/MHC class II+ (Supporting information, Fig. S2). Interestingly, as few as 0·2–0·3 × 106 CD11c+-enriched DC, representing only a minimal fraction of fewer than 1% of unseparated cells, was able to mediate the full therapeutic activity of ECP (Fig. 5). These results suggested that UVA-exposed, 8-MOP-treated CD11c+ DC are key cells mediating the therapeutic activity of ECP.
![Dendritic cells (DC) mediate the therapeutic effect of extracorporeal photopheresis (ECP). The spleen and lymph node cells were obtained from the 2-chloro-1,3,5-trinitrobenzene (TNCB)-sensitized mice. Different cell fractions were used for ECP treatment of the recipient mice: CD11c+/mPDCA-1+DC-depleted fraction (DC-) (30 × 106cells), fluorescence-activated cell sorted (FACS) CD11c+dendritic cells (CD11c+; 0·2–0·3 × 106cells) and unseparated splenocytes/lymph node cells (bulk; 30 × 106). The cells were exposed extracorporeally to 8-methoxypsoralen (8-MOP) and ultraviolet A (UVA) radiation (2 J/cm2) and injected intravenously (i.v.) into the recipients which had been sensitized with TNCB 3 days previously. Twenty-four h after cell transfer, the recipients were ear-challenged by the application of TNCB. As a positive control, the mice were sensitized with TNCB and were ear-challenged with the same hapten without ECP treatment. Ear-swelling was measured on days 1 and 2 after challenge. The ear-swelling response is expressed as the difference [µm, mean ± standard error of the mean (s.e.m.)] between ear thickness before and after challenge. Positive control:n = 8; DC-:n = 8; bulk:n = 8; CD11c+:n = 7; two independent experiments; statistical tests: one-way analysis of variance (anova) test, Tukey's post-test; ***P < 0·001, ****P < 0·0001.](https://oup.silverchair-cdn.com/oup/backfile/Content_public/Journal/cei/203/2/10.1111_cei.13539/4/m_cei13539-fig-0004.jpeg?Expires=1748251889&Signature=cLO6M4UPUsUkZszwqoCLUgH1mUd8d4m6RHKss27ThV7f5njBFTsRJXix06GKk4G6D~qulU~LYQ46CCla3qUXB-MwVuCXeJkku7JE9ChNne0Owd7fOVp864cYQ18B2Zgu~gkiT3eB8A006yBEIRD7Avf6V2OYZjiknUve9rsPXgrOGWay3PyugfULCsbEn2JrC4Ue3WxrBZUxMbbG4o8rBn5NaE~~rBPNcz0VDLGsUsOQJDhQwYTyX0h9kBkGgGvhjmzVTpAkfqCrgPvzEaqmuk4myS6BvmXHrpZ8WwbyeOM0s0b5AJb5zUc3mUoDyWQNmFPEF9LYTZufcEu4ywTExA__&Key-Pair-Id=APKAIE5G5CRDK6RD3PGA)
Dendritic cells (DC) mediate the therapeutic effect of extracorporeal photopheresis (ECP). The spleen and lymph node cells were obtained from the 2-chloro-1,3,5-trinitrobenzene (TNCB)-sensitized mice. Different cell fractions were used for ECP treatment of the recipient mice: CD11c+/mPDCA-1+DC-depleted fraction (DC-) (30 × 106cells), fluorescence-activated cell sorted (FACS) CD11c+dendritic cells (CD11c+; 0·2–0·3 × 106cells) and unseparated splenocytes/lymph node cells (bulk; 30 × 106). The cells were exposed extracorporeally to 8-methoxypsoralen (8-MOP) and ultraviolet A (UVA) radiation (2 J/cm2) and injected intravenously (i.v.) into the recipients which had been sensitized with TNCB 3 days previously. Twenty-four h after cell transfer, the recipients were ear-challenged by the application of TNCB. As a positive control, the mice were sensitized with TNCB and were ear-challenged with the same hapten without ECP treatment. Ear-swelling was measured on days 1 and 2 after challenge. The ear-swelling response is expressed as the difference [µm, mean ± standard error of the mean (s.e.m.)] between ear thickness before and after challenge. Positive control:n = 8; DC-:n = 8; bulk:n = 8; CD11c+:n = 7; two independent experiments; statistical tests: one-way analysis of variance (anova) test, Tukey's post-test; ***P < 0·001, ****P < 0·0001.
![The therapeutic effect of extracorporeal photopheresis (ECP) mediated by dendritic cells is antigen-specific. The spleen and lymph node cells were obtained from the mice that were sensitized with 2-chloro-1,3,5-trinitrobenzene (TNCB) or oxazolone (OXA). The CD11c+dendritic cells were enriched by fluorescence-activated cell sorting (FACS). The cells were exposed extracorporeally to 8-methoxypsoralen (8-MOP) and ultraviolet A (UVA) radiation (2 J/cm2). The ECP-treated CD11c+dendritic cells (0·2–0·3 × 106) were injected intravenously (i.v.) into the recipients which had been sensitized with TNCB 3 days previously. For comparison, the ECP-treated unseparated splenocytes/lymph node cells that were obtained from the TNCB-sensitized mice were infused (bulk; 30 × 106). Twenty-four h after cell transfer, the recipients were ear-challenged by the application of TNCB. As a positive CHS control, the mice were sensitized with TNCB and were ear-challenged with the same hapten without ECP treatment. Ear swelling was measured on days 1 and 2 after challenge. The ear-swelling response is expressed as the difference [µm, mean ± standard error of the mean (s.e.m.)] between mean ear thickness before and after challenge. Positive control TNCB:n = 6; CD11c+OXA:n = 8; CD11c+TNCB:n = 7; two independent experiments; statistical test: Kruskal–Wallis test; Tukey's post-test; *P < 0·05, **P < 0·01, ***P < 0·001.](https://oup.silverchair-cdn.com/oup/backfile/Content_public/Journal/cei/203/2/10.1111_cei.13539/4/m_cei13539-fig-0005.jpeg?Expires=1748251889&Signature=Mdkmvcssm-7Viw2-OjHx44DzIOhMlnOsL5jIM5IaBzS~mk6Y5WRZyAh4x6pthy6z5h-oa2nc9E0PeBdowJQB9PGL7Ci4uW8C8WYiAt39JlQcZQbSlWJLfgWWE7O4YXTutDwg6A4dP3lfthjvYh5yz~qeSNxtuhq3wPb-aTiAihrJDH10Cd0FZkhxYAbN~5AVxkhnYDYQCOmStte7-wA2AImPcozKsWYdpZZ74dtH7TsbqiC3NeoEG3A52ayYvOSyutgx46yBVdJu8H7jgXQTSjPqRDowY2~1KmDXjIEOhieoAm3zxtaeoGO8yTXGmHodjll3RoumggfOGJH7aMj5yg__&Key-Pair-Id=APKAIE5G5CRDK6RD3PGA)
The therapeutic effect of extracorporeal photopheresis (ECP) mediated by dendritic cells is antigen-specific. The spleen and lymph node cells were obtained from the mice that were sensitized with 2-chloro-1,3,5-trinitrobenzene (TNCB) or oxazolone (OXA). The CD11c+dendritic cells were enriched by fluorescence-activated cell sorting (FACS). The cells were exposed extracorporeally to 8-methoxypsoralen (8-MOP) and ultraviolet A (UVA) radiation (2 J/cm2). The ECP-treated CD11c+dendritic cells (0·2–0·3 × 106) were injected intravenously (i.v.) into the recipients which had been sensitized with TNCB 3 days previously. For comparison, the ECP-treated unseparated splenocytes/lymph node cells that were obtained from the TNCB-sensitized mice were infused (bulk; 30 × 106). Twenty-four h after cell transfer, the recipients were ear-challenged by the application of TNCB. As a positive CHS control, the mice were sensitized with TNCB and were ear-challenged with the same hapten without ECP treatment. Ear swelling was measured on days 1 and 2 after challenge. The ear-swelling response is expressed as the difference [µm, mean ± standard error of the mean (s.e.m.)] between mean ear thickness before and after challenge. Positive control TNCB:n = 6; CD11c+OXA:n = 8; CD11c+TNCB:n = 7; two independent experiments; statistical test: Kruskal–Wallis test; Tukey's post-test; *P < 0·05, **P < 0·01, ***P < 0·001.
The therapeutic effect of ECP-treated dendritic cells is antigen-specific
To confirm the antigen-specificity of purified CD11c+ dendritic cell fractions, we prepared these fractions from animals sensitized with different haptens (TNCB, OXA). After ECP treatment, the cells were transferred into the TNCB-sensitized recipient mice and ear-swelling was monitored after the hapten challenge. Only the ECP-treated CD11c+ DC from the TNCB-sensitized donor animals were able to inhibit ear-swelling in the TNCB-sensitized recipients. Conversely, the ECP-treated CD11c+ cells from OXA-sensitized animals were inactive, indicating that the therapeutic activity of the ECP-treated CD11c+ DC is antigen-specific (Fig. 5).
Discussion
We developed a robust hapten-specific experimental ECP protocol that suppressed the effector phase of a pathological T cell response based on previous work of van Iperen [22] and Maeda [19,23]. The UVA dose was a vital variable for therapeutic success. UVA dosing above 2 J/cm2 reduced the suppressive effect of ECP on the CHS response. Moreover, we report here that the therapeutic effects of the experimental ECP strongly depend upon the quantity of the ECP-treated cells that are infused to the recipient. The impact of cell dose on ECP responsiveness is seen in observational clinical studies (for review see Worel et al. [24]), suggesting that escalating the cell dose in clinical ECP protocols may improve the response rates. Also, we report that the purified ECP-treated CD11c+ DC (harvested from antigen-experienced donors) mediated suppression of CHS in sensitized recipients. The therapeutic effect was hapten-specific; pretreatment of the ECP cell donor with an unrelated hapten did not lead to the suppression of TNCB-mediated CHS. The number of ECP-treated CD11c+ cells mediating a therapeutic effect was 100 times lower (0·2–0·3 × 106) compared to the number of ECP-treated unseparated splenocytes that mediated a similar effect (30 × 106). Thus, the vast majority of other apoptotic splenocytes were not essential for the hapten-specific immunosuppressive effect (induction of apoptosis in splenocytes and expression of MHC class II on ECP-treated DC are presented in Supporting information, Figs. S3 and S4).
The results of the experiments presented in Fig. 4 suggest that isolated CD11c+ cells mediate stronger therapeutic effects compared to bulk cells. Figure 5 suggests the opposite result, i.e. bulk cells may be more effective compared to isolated CD11c+ cells. These results may represent day-to-day variation and are not directly comparable.
Other preclinical in-vivo models showed that ECP suppressed allogeneic skin graft rejection [25–27], cardiac allograft rejection [28–30], GVHD [31–35], CHS [19,20,22,23,36], experimental allergic encephalomyelitis [37,38], pathology in lupus-prone mice [39,40], type 1 diabetes in non-obese mice [41] and collagen-induced arthritis (CIA) [42]. The experimental design of these studies involved a donor of ECP-treated cells, incubation of donor cells with 8-MOP (range = 100–250 ng/ml), UVA irradiation (range = 1–5 J/cm2) of donor cells and i.v. infusion of the ECP inoculum (1 × 106 – 1 × 107 donor cells) to a recipient. Most studies used unseparated splenocytes of the ECP cell donor. Photopheresis-treated cells traffic to spleen, liver and, to a much lesser extent, to lymph nodes and small intestines [19]. All preclinical studies showed that ECP may suppress pathological T cell responses directed to defined alloantigens, haptens or autoantigens in the recipient. However, the experimental designs of these studies used fundamentally different concepts depending on different hypotheses about the mechanism of action of ECP.
The first concept relates to the question of whether the cognate antigen of the pathological T cell response is present in the ECP-treated inoculum or not. If a naive ECP cell donor is syngeneic to the recipient of an allogeneic skin transplant [27] or organ transplant [29], the cognate alloantigen (i.e. minor or major histocompatibility antigen of the graft donor) is not present in the ECP-treated inoculum and immunosuppressive effects are not donor-specific. The same holds true in experimental ECP treatment of GVHD if a naive ECP cell donor is syngeneic to the bone marrow donor [32,34,35], as pathological T cell responses in the setting of active GVHD are directed to the recipient but not donor, minor or major histocompatibility antigens. In these protocols, even third-party ECP cell donors [32,35] mediated immunosuppressive effects in the recipient. The antigen-independent effects of ECP treatment (that are obvious in these experimental protocols) may mirror immunomodulating effects that are also seen in other apoptotic cell-based therapies (for review see [43]). Conclusively, in some experimental systems, the infusion of any apoptotic cell type may induce non-specific immunosuppression.
In other experimental ECP protocols, the ECP cell donor is antigen-experienced; that is, skin-transplanted [25,26], bone marrow-transplanted [31,34], sensitized to a hapten [19,22,23,36] or autoantigen [37,42]. In these models, spleen APC of the ECP cell donor may present cognate antigens against which the pathological T cell response of the recipient is directed. The immunosuppressive effect of ECP treatment using an antigen-experienced ECP cell donor was antigen-specific, as exhibited by the unaltered response to unrelated antigens [19]. Arguably, the cognate antigen is not present within the ECP inoculum if there is a long-term lag between the sensitization of the ECP cell donor and the harvest of spleen cells. In a model of CIA, this time-frame exceeded 3 weeks [42]. However, immunization via subcutaneous injection of an emulsion of antigen (in this case, bovine collagen type II in complete Freund's adjuvant) is known as a ‘depot’ effect, leading to localizing and slowly releasing intact antigen for many weeks [44]. In this CIA model, ECP-treated splenocytes from non-immunized donors induced no therapeutic effect. Conclusively, in experimental models where the ECP cell donor is antigen-experienced, the cognate antigen is presumably presented by APC within the spleen or lymph node compartment, and ECP treatment leads to antigen-specific suppression of T cell responses that are directed to cognate antigens within the ECP inoculum.
In addition to the cognate antigen, spleen cells and lymph node cells of antigen-experienced ECP cell donors may include pathogenic T cells that are uniquely characterized by clonotypical T cell receptors. It has been hypothesized that ECP-induced cell death may be immunogenic and may induce an anti-clonotypical T cell response in the recipient, directed to T cell receptor peptides derived from pathogenic T cell clones [18]. The findings in our experimental model do not support this hypothesis, as the time-frame of 24 h between painting the ECP cell donor and spleen cell and lymph node harvest is too short for the sequential steps of antigen uptake by skin APC, migration of APC to the spleen/lymph node, T cell pairing with APC, T cell ‘blasting’ and clonal expansion of pathogenic T cells (for review see Obst [45]).
The second experimental concept relates to the question of whether ECP treatment can suppress the sensitization of a naive recipient, i.e. in a prophylactic setting, or whether ECP treatment can suppress an established immune response, i.e. in a therapeutic setting. Many experimental protocols investigated the efficacy of ECP treatment in a prophylactic setting [19,20,23,25–27,29,30,32,33,36,38–41]; however, in clinical medicine, ECP treatment is usually indicated in therapeutic settings, e.g. GVHD, graft rejection or autoimmune diseases. Nevertheless, experimental ECP protocols addressing the effector phase of a pathological T cell response showed considerable therapeutic effects [22,23,31,34,37,42].
In addition to ECP treatment effects on clinical end-points, mechanistic effects of ECP-treated cells on the recipient immune response were investigated in several experimental protocols. Suppressive effects were adoptively transferred to naive mice by splenocytes [19,26,30] and were abrogated by depletion of CD4+ [19] or CD25+ [19,31] T cells. Also, it did not occur in IL-10-deficient recipients [23,33], and did not occur if IL-10-deficient bone marrow donors were used in GVHD setting [32]. Moreover, suppressive effects were abrogated by anti-IL-10 administration [23] and depletion of forkhead box protein 3 (FoxP3)+ T cells [33]. Overall, these studies suggested that regulatory T cells (Treg) cells or type 1 regulatory T cells (Tr1) cells are induced by infusion of the ECP-treated cells. Also, it is known that experimental ECP induces IL-10 in circulating B cells [27] and induces regulatory CD8+ T cells [20].
However, the cellular contributors within the ECP-treated inoculum mediating these effects remained unclear. Maeda et al. [19] showed that infusion of ECP-treated splenocytes and lymph node cells from a hapten-experienced donor caused inhibition of a hapten response in naive mice, and that this inhibitory effect was lost upon depletion of CD11c+ cells. However, the possibility remained that donor CD11c+ cells are converted to tolerogenic DC by uptake of apoptotic splenocytes and lymph node cells within the ECP-treated inoculum. Conversely, our findings revealed that ECP-treated donor CD11c+ DCs alone mediated specific inhibition of CHS response. Our results are supported by a recent study by Hequet et al. [20], who showed that infusion of ECP-treated in-vitro haptenized bone marrow-derived DC prevented antigen-specific development of CHS in naive mice.
The question arises of whether ECP-treated DC, presenting the tolerizing antigen, directly interact with recipient CD4+ or CD8+ cells after infusion or whether ECP-treated DC are phagocytosed by recipient APC that are converted consecutively into tolerogenic APC. This may take place in the recipient's spleen, as ECP-mediated immunosuppression did not develop in splenectomized mice [23]. We had already detected annexin V binding of CD11c+ cells 2 h after ECP treatment, indicating early apoptosis within the DC inoculum (data on induction of early and late apoptosis are presented in the Supporting information, Fig. S1). In vitro, uptake of apoptotic DC and not uptake of apoptotic splenocytes converted immature DC into tolerogenic DC [46]. One explanation for different effects of uptake of different apoptotic cell types is provided by Medina et al. [47]. Apoptotic cells release specific metabolites and this is not simply due to passive emptying of cellular contents, but a regulated process. A subset of apoptotic metabolites is cell type-specific and acts as a messenger inducing specific gene programs in healthy neighboring cells [47]. In this context, it would be interesting to analyze the metabolite secretome of ECP-treated DC.
The different capacity of different apoptotic cell types to induce antigen-specific immunosuppression is illustrated in another tolerizing setting by the treatment of donor-derived peripheral blood mononuclear cells (PBMC) with the chemotherapeutic drug mitomycin C in a rat transplantation model [48]. Infusion of mitomycin C-treated PBMC prolonged heart allograft survival and removal of monocytes from the inoculum completely abrogated their suppressive effect, demonstrating in a different model that allogeneic apoptotic APC, and not lymphocytes, induced immunosuppression. This protocol was effective in a clinical Phase I trial in living donor kidney transplantation, and treated patients showed the immune tolerance network operational tolerance signature [49].
We conclude from the results of our study and published trials that the tolerizing effects of ECP are mediated by antigen-presenting DC that are exposed to 8-MOP and UVA irradiation. It has been proposed that ECP is a bidirectional therapy, both tolerizing in the setting of alloreactive and autoreactive T cell pathologies and immunizing in the setting of CTCL [16]. Edelson et al. recently developed an elegant protocol to differentiate monocytes to DC by the interaction of monocytes with platelets under flow conditions in an experimental ECP chamber [17]. Feeding the resulting DC with ECP-treated tumor cells and subsequent infusion of tumor-loaded DC was an effective immunotherapeutic agent in tumor-bearing mice. ECP treatment (exposure to 8-MOP and UVA irradiation) of DC abrogated the anti-tumor activity, indicating that vital DC are required for this DC vaccination strategy [17]. Thus, 8-MOP-UVA-spared ECP-induced DC are antigen-specific immunizing agents mediating potent anti-cancer effects.
The finding that ECP-treated DC mediate tolerizing effects may be used for predicting the response to ECP in the clinical setting of GVHD. Furthermore, systemic immunosuppression has an impact upon DC function and may affect the efficacy of ECP.
By dissecting the role of the cellular contributors that facilitate the tolerizing effect of ECP, antigen-specific therapies directed to T cell-mediated pathologies can now be optimized. The protocol consists of ECP-treated DC presenting the cognate antigen of disease-driving pathological T cells: recipient histocompatibility antigens in case of GVHD, donor histocompatibility antigens in case of organ transplantation and autoantigens in case of autoimmune diseases. ECP has an excellent safety profile and does not lead to generalized immunosuppression, as the response to novel or recall antigens is normal in treated patients [50]. Thus, it deserves further research to test whether the concept of antigen-specific tolerizing DC therapy holds true for other pathologies and whether this targeted approach may be translated to optimized clinical application.
Acknowledgements
The authors would like to thank the Von Behring-Röntgen-Stiftung (64-0019) and the German José Carreras leukemia foundation (AH06-01) for funding of this project. Open access funding enabled and organized by ProjektDEAL.
Disclosures
The authors declare that they have no competing interests.
Author contributions
H. H., C. B., A. N., T. J. and G. B. designed the study. A. K., B. D., I. S. K., N. B. and G. M. performed in vivo and in vitro experiments. H. H., A. K. and N. B. analyzed the data. H. H., A. K. and G. B. contributed to the first draught of the manuscript. C. B., A. N. and T. J. interpreted the data and critically revised the manuscript. G. B. assumed the final responsibility to submit the manuscript for publication. All authors had full access to all the data, carefully reviewed the manuscript and approved the final version.
Data availability statement
The datasets generated during and/or analysed during the current study are available from the corresponding author on reasonable request.
References
Author notes
These authors contributed equally to this work as first authors.
These authors contributed equally to this work as senior authors.