-
PDF
- Split View
-
Views
-
Cite
Cite
Lukas B Krone, Vladyslav V Vyazovskiy, Unresponsive or just asleep? Do local slow waves in the perilesional cortex have a function?, Brain, Volume 143, Issue 12, December 2020, Pages 3513–3515, https://doi.org/10.1093/brain/awaa396
- Share Icon Share
This scientific commentary refers to ‘Local sleep-like cortical reactivity in the awake brain after focal injury’, by Sarasso et al. (doi:10.1093/brain/awaa338).
Sleep is typically defined by changes in an organism’s responsiveness to its environment. These manifest as an elevated arousal threshold at the global behavioural level, and diminished or enhanced neural responses to afferent inputs, arising either internally or externally. Both aspects of altered responsiveness during sleep may be related to the occurrence of slow waves, the EEG hallmark of non-rapid eye movement (NREM) sleep. While behavioural features of sleep show astonishing variation between species, the electrophysiological phenomenon of slow waves has become almost synonymous with sleep. For example, the discovery that slow waves can occur locally, and may be associated with impaired behavioural performance during wakefulness, led to the idea that the brain may engage in ‘local sleep’. But whether EEG slow waves are responsible for the altered responsiveness of the brain during sleep, remains to be determined.
Brain lesions as a result of ischaemia, haemorrhage, or trauma are the leading cause of permanent disability in adults. While it is not surprising that the lesioned area of the brain loses its function, the adjustments in the surrounding nervous tissue and the functional consequences thereof, remain poorly defined. In this issue of Brain, Sarasso and co-workers investigated cortical evoked responses in awake patients with chronic brain injuries of diverse aetiology, using a combination of transcranial magnetic stimulation (TMS) and high-density EEG championed by this research group for many years (Sarasso et al., 2020). Using TMS to ‘perturb’ perilesional cortex, the authors reveal a cortical response similar to that typically observed during NREM sleep or in disorders of consciousness. Importantly, this sleep-like response was not observed over cortical areas in the contralateral intact hemisphere of patients with unilateral injury, and did not occur when probing multiple cortical areas in a severely impaired group of patients with subcortical lesions (Fig. 1). The authors also describe how the presence of perilesional evoked ‘off periods’—an additional metric for local sleep-like responses—was associated with a local reduction in signal complexity, a TMS/EEG readout recently proposed for the objective evaluation of patients with disorders of consciousness (Casarotto et al., 2016). This novel observation of local sleep-like responses in perilesional cortex adds a new spatial dimension to the established findings of EEG slowing in patients after stroke (Herron et al., 2014), and unilateral changes observed over the lesioned hemisphere in patients with cortical, but not subcortical, lesions (Macdonell et al., 1988).
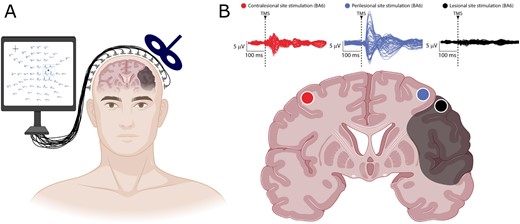
A combined TMS/EEG approach reveals local slow waves in perilesional cortex. Key findings of Sarasso et al. (2020). (A) Combination of a high-density EEG recording and neuronavigated TMS at different cortical sites (here illustrated for perilesional stimulation) allows mapping of EEG responses to cortical perturbations in awake patients with chronic brain injuries. (B) Slow waves in TMS-evoked EEG potentials of awake patients are specific for perilesional stimulation sites. Top: Butterfly plots of the TMS-evoked EEG potentials recorded at all 60 electrodes for three stimulation sites: contralesional (red), perilesional (blue) and targeting the lesion (black) in one representative patient. Bottom: Schematic of a cortico-subcortical brain lesion (shaded area) and different stimulation sites (coloured dots) shown on a coronal brain section. TMS = transcranial magnetic stimulation. Figure created with BioRender.com.
In its traditional meaning, the word ‘sleep’ refers to a global behavioural state that, on the one hand is incompatible with behavioural performance, and, on the other hand, has numerous benefits, including prophylactic, protective and recovery functions. Whether this applies to the same degree to ‘local sleep’ is unknown, but Sarasso et al. entertain the idea that investigating properties of altered network activity in the perilesional area may represent a valuable and as yet unexplored diagnostic tool, for example, for evaluating the chances of recovery. One could even speculate that non-invasive brain stimulation techniques, commonly used to modulate local brain activity, could ‘wake up’ the dormant areas around the centre of the lesion and promote recovery. However, equating sleep (with its well-known benefits) with EEG slow waves or off periods warrants great caution. Beyond the field of sleep research, slow wave-like activity has been described as a primordial state of neural networks, as a predominant type of oscillation during some forms of anaesthesia, and as the result of brain hypoperfusion associated with cardiac arrest in syncope. Slow wave-like activity has also been reported in disconnected cortical slabs and even in cell cultures. Some studies suggest a neuroplastic effect of slow waves while others favour the view of a ‘default mode’ in which slow waves merely represent persistent bistable activity in interconnected neuronal cell populations. The jury is still out to which of these categories local perilesional slow waves belong, and further research is needed to clarify their physiological origin, such as a diminished blood supply, or a partial deafferentation. While it is likely that local network bistability in the perilesional area is merely an epiphenomenon, and impairs behavioural performance, the possibility remains that slow waves in perilesional areas have a neuroprotective role and therefore improve the prospect of recovery for those functions that were impaired by the lesion. Given the fact that NREM sleep is generally associated with a decrease in cerebral metabolism, which further correlates, at a local level, with slow-wave activity (Tüshaus et al., 2017), it is tempting to speculate that local slow waves in perilesional areas may have a restorative role.
It must further be emphasized that sleep need is under homeostatic control, ensuring that sleep deficits are readily compensated. Extensive research on the bidirectional relationship between sleep and stroke reveals a high prevalence of sleep disorders in stroke patients (Baglioni et al., 2016; Duss et al., 2017). While there are many explanations for this, including commonly observed disordered breathing during sleep, evidence suggests that cortical stroke locations are associated with poorer sleep quality and the most severe changes in sleep architecture (Gottlieb et al., 2019). Even in basic research models, it is still unclear whether or how local cortical activity contributes to global sleep need (Thomas et al., 2020), but very recent evidence suggests the intriguing possibility of a role of the cortex in sleep regulation (Krone et al., 2020). While it remains unknown how and at what level or timescale the local and global aspects of sleep homeostasis interact, the possibility that persistent local sleep in cortical areas around focal lesions influences the global dynamics of sleep homeostasis cannot be excluded. It would be interesting to investigate, for example, whether the precise location or the extent of the perilesional area enriched with slow waves correlates with the overall amount of sleep, sleep latency, global sleep architecture or the homeostatic build-up of sleep pressure.
The clinical observations made by von Economo in the early 20th century describing sleep disruptions in encephalitic lethargica patients with local brain lesions provided the groundwork for today’s understanding of the sleep-wake switch (Saper et al., 2005). Still, a century later, the nature of the mysterious processes underlying sleep need remain unclear, and we continue to lack insight into the neurochemical nature of slow wave regulation—both during physiological sleep and in pathology. The observation that slow wave-like events can be readily induced in the perilesional area suggests the interesting possibility of a lowered threshold for their occurrence, possibly indicating that the local environment is closer to the one observed during physiological sleep than in wakefulness. Among many potential mechanistic explanations for perilesional ‘local sleep’ are metabolic changes, neuroplastic changes, an altered neurotransmitter milieu or local immune responses. These are not easy to assess directly in human patients and therefore perturbation approaches, such as those used by Sarasso et al., remain central to the quantitative assessment of the degree of perilesional impairment, and, in combination with functional brain imaging and nuclear magnetic resonance tomography, could help unravel the neurochemical basis of slow waves.
In summary, Sarasso et al. describe a form of sleep-like activity in the area surrounding the lesioned cortex of patients with chronic focal brain injury. This finding poses a tantalizing question to clinicians and basic researchers alike about the functional significance of local sleep and its relationship to global sleep regulation. The possibility remains that the local slow waves described here represent the electrophysiological expression of a dysfunctional cortex irreversibly trapped in its default mode of activity. Alternatively, local slow waves may reflect neuroprotective or restorative processes, which contribute to recovery. Yet, an even more exciting possibility is that local sleep phenomena reflect the functional state of a brain area unable to partake in information processing, and exerting a detrimental effect on the fine-tuned global sleep-regulatory process. If this is the case, perilesional cortex is only waiting to be awoken: its reintegration into functional brain networks would then help the patient to regain lost functions. Transcranial magnetic, sensory or electrical stimulation methods could make it possible not only to probe local sleep-like mechanisms, but also to awaken the ‘sleeping beauty’ in the lesioned brain.
Funding
This work was supported by the Wellcome Trust PhD studentship 203971/Z/16/Z, Medical Research Council grant MR/S01134X/1 and Hertford College, University of Oxford.
Competing interests
The authors report no competing interests.