-
PDF
- Split View
-
Views
-
Cite
Cite
Johanna Lind, Jonas Persson, Martin Ingvar, Anne Larsson, Marc Cruts, Christine Van Broeckhoven, Rolf Adolfsson, Lars Bäckman, Lars-Göran Nilsson, Karl Magnus Petersson, Lars Nyberg, Reduced functional brain activity response in cognitively intact apolipoprotein E ε4 carriers, Brain, Volume 129, Issue 5, May 2006, Pages 1240–1248, https://doi.org/10.1093/brain/awl054
- Share Icon Share
Abstract
The apolipoprotein E ε4 (APOE ε4) is the main known genetic risk factor for Alzheimer's disease. Genetic assessments in combination with other diagnostic tools, such as neuroimaging, have the potential to facilitate early diagnosis. In this large-scale functional MRI (fMRI) study, we have contrasted 30 APOE ε4 carriers (age range: 49–74 years; 19 females), of which 10 were homozygous for the ε4 allele, and 30 non-carriers with regard to brain activity during a semantic categorization task. Test groups were closely matched for sex, age and education. Critically, both groups were cognitively intact and thus symptom-free of Alzheimer's disease. APOE ε4 carriers showed reduced task-related responses in the left inferior parietal cortex, and bilaterally in the anterior cingulate region. A dose-related response was observed in the parietal area such that diminution was most pronounced in homozygous compared with heterozygous carriers. In addition, contrasts of processing novel versus familiar items revealed an abnormal response in the right hippocampus in the APOE ε4 group, mainly expressed as diminished sensitivity to the relative novelty of stimuli. Collectively, these findings indicate that genetic risk translates into reduced functional brain activity, in regions pertinent to Alzheimer's disease, well before alterations can be detected at the behavioural level.
Introduction
Alzheimer's disease is the most common form of dementia in all ages, with prevalence and incidence rates that increase exponentially with increasing age (Fratiglioni et al., 2000; Lobo et al., 2000). Worldwide, 0.3–1.0% in the age group 60–64 are affected, and 42.3–68.3% at the age of 95 and older (Fratiglioni et al., 1999). Clinically, the disease is characterized by gradual, inevitable loss of explicit memory, preceded by progressive neuropathological damage to the brain (Braak and Braak, 1997; Price and Morris, 1999). The neuronal hallmarks (neuritic plaques and neurofibrillary tangles) are yet not satisfactorily detectable in vivo, making early diagnosis difficult. This is a problem because emerging treatments are more efficient to slow or halt disease progression if administered at an early stage (Grundman et al., 1998; Sano, 2003).
Genetic studies have ameliorated these shortcomings by identifying the apolipoprotein E ε4 allele (APOE ε4) as a susceptibility gene for Alzheimer's disease (Corder et al., 1993; Saunders et al., 1993; Strittmatter et al., 1993; Farrer et al., 1997). The APOE locus has three naturally occurring isoforms (ε3, ε4 and ε2), of which ε3 is the most and ε2 the least common. While APOE ε2 appears to be protective, APOE ε4 increases risk for and decreases onset age of Alzheimer's disease in a dose-dependent manner such that homozygous APOE ε4 carriers are most at risk (Corder et al., 1993, 1994). The exact role of the APOE protein and the mechanisms by which APOE genotype influences the pathogenesis of Alzheimer's disease are still not fully understood. Nevertheless, the APOE protein has been associated with most of the biochemical disturbances characteristic of the disease (Cedazo-Minguez and Cowburn, 2001), including, for example, synaptic repair functions, neurotoxicity, neuritic plaques and neurofibrillary tangles.
Genetic information alone, however, is not sufficient as a predictor of who will eventually develop the disease. Instead, to improve sensitivity and specificity of predictions for Alzheimer's disease development on the individual level, genetic assessments need to be combined with other diagnostic tools such as biological markers and neuropsychology. In addition, the contribution of structural and functional brain imaging has been emphasized (Small, 1996). Previous studies with PET and functional MRI (fMRI) have indicated alterations in glucose metabolism (Small et al., 1995, 2000; Reiman et al., 1996, 2004) and task-related brain activation patterns (Smith et al., 1999; Bookheimer et al., 2000) in APOE ε4 carriers, well before the diagnosis of Alzheimer's disease. However, the nature of these changes remains unclear. Whereas some studies have found that increased risk is accompanied by reduced functional brain activity in parietal, temporal and frontal areas (Smith et al., 1999; Elgh et al., 2003), others have found increased activity in the same general areas (Bookheimer et al., 2000; Smith et al., 2002).
To further address the issue of how genetic risk translates into altered functional brain activity, we used fMRI to examine individuals who had been genotyped for APOE. We contrasted APOE ε4 carriers (n = 30) with APOE ε3/3 carriers (n = 30), and also examined a dose-related effect by comparing carriers of either one or two alleles (APOE ε3/4 and ε4/4) and non-carriers (APOE ε3/3), respectively. To our knowledge, this is the first fMRI study to investigate a possible dose effect in asymptomatic carriers of the APOE ε4 allele.
The fMRI measurements were taken while participants performed a simple semantic categorization task, with novel (not encountered previously during the test phase) and familiar (repeatedly presented during the test phase) items intermixed. This and related tasks have been found to engage several cortical regions, particularly in the left hemisphere (Kiehl et al., 1999; Wagner et al., 2000). Of chief interest was whether genetic risk would modulate activity in engaged regions, such as in the parietal cortex where changes have been observed for Alzheimer's disease patients (Frackowiak et al., 1981; Bäckman et al., 1999; Boxer et al., 2003), and for those at genetic risk (Small et al., 1995; Reiman et al., 2005). In addition, we were interested to see if APOE isoforms would affect hippocampal responding, as this region is one of the earliest to show pathological signs in Alzheimer's disease (Braak and Braak, 1997; Price and Morris, 1999; Fox et al., 2001; Scahill et al., 2002). Contrasts between processing of novel and familiar items have revealed differential activity in the hippocampal region (Tulving and Schacter, 1990; Tulving et al., 1994; Dolan and Fletcher, 1997; Grunwald et al., 1998; Saykin et al., 1999; Duzel et al., 2003). A recent study compared brain activity during processing of novel and familiar scenes, and found that a specific genetic polymorphism (BDNF val66met) modulated the associated hippocampal activity (Hariri et al., 2003). Analogously, we contrasted processing of novel and familiar items and compared the results with regard to APOE isoform.
Methods
Participants
Group characteristics are summarized in Table 1. All participants were from The Betula Prospective Cohort Study: memory, health, and aging (Nilsson et al., 1997), which is an ongoing longitudinal study containing extensive cognitive and medical data, including APOE status, for ∼3500 persons (for a full description of the Betula project, see Nilsson et al., 2004). For the present purpose, 60 cognitively intact persons between 49 and 79 years of age were recruited. Thirty subjects were carriers of at least one copy of APOE ε4: 10 were homozygous (ε4/4) and 20 were heterozygous (ε3/4). The remaining 30 subjects carried two copies of APOE ε3 and served as controls. To examine a possible dose effect, three subgroups consisting of 10 subjects each were formed: APOE ε4/4, APOE ε3/4 and APOE ε3/3. All test groups were closely matched according to sex, age and length of education. As an initial step—owing to the low frequency of homozygous APOE ε4 carriers in the population and hence in the Betula cohort—each APOE ε4/4 carrier in the Betula pool of subjects was contacted; 10 subjects met the inclusion criteria and agreed to participate. The ε3/4 and ε3/3 carriers were subsequently selected to match the initially recruited ε4/4 carriers. All subjects were non-demented and scored ≥24 on the Mini-Mental State Examination (Folstein et al., 1975). They all lived independently in their own homes. Approximately 2 years after the reported MRI testing, 55 of the original 60 subjects were re-tested as a part of the longitudinal Betula project and they still showed no signs of dementia (see Table 1 for Mini-Mental State Examination and word comprehension test results). In addition, we compared the APOE ε4 carriers' explicit memory performance (based on three tests: face recognition, verbal recall, and recall of actions; for detailed description of the tests, see Nilsson et al., 1997) with normative data available from the Betula database. Twenty-eight of the 30 ε4 carriers performed within 1 SD of the mean of their age group; two subjects scored below 1 SD, but performed within 1 SD on the follow-up test (see above) 2 years after MRI testing. These results provide evidence that all participants were cognitively intact.
. | APOE ε4a(n = 30) . | APOE ε3/3(n = 30) . | APOE ε4/4(n = 10) . | APOE ε3/4(n = 10) . | APOE ε3/3(n = 10) . |
---|---|---|---|---|---|
Female/male . | 19/11 . | 18/12 . | 9/1 . | 7/3 . | 8/2 . |
Age | 65.3 (7.9) | 66.6 (8.3) | 61.2 (9.4) | 65.0 (8.5) | 64 (11.1) |
Range | 49–74 | 49–79 | 49–74 | 49–74 | 50–79 |
Education (years) | 10.6 (3.5) | 10.2 (3.3) | 11.7 (3.1) | 10.7 (4.0) | 11.8 (3.1) |
Range | 6–17 | 6–16 | 8–16 | 6–17 | 9–16 |
MMSE | 28.2 (1.5) | 27.9 (1.7) | 28.5 (1.4) | 28.4 (1.4) | 28.1 (2.1) |
Range | 24–30 | 24–30 | 26–30 | 26–30 | 24–30 |
MMSEb (2 years later) | 28.3 (1.5) | 28.3 (1.6) | 27.9 (2.0) | 28.7 (1.1) | 28.3 (1.6) |
Range | 25–30 | 26–30 | 25–30 | 27–30 | 26–30 |
SRB | 25.0 (2.4) | 22.6 (4.8) | 23.7 (2.8) | 25.3 (2.4) | 23.2 (3.8) |
Range | 16–29 | 11–29 | 16–26 | 22–29 | 17–28 |
SRBb (2 years later) | 24.9 (2.5) | 23.4 (4.5) | 24.3 (3.2) | 25.3 (1.8) | 23.4 (4.5) |
Range | 18–28 | 14–28 | 18–27 | 23–28 | 14–28 |
AD in family (N) | 2 | 0 | 0 | 1 | 0 |
. | APOE ε4a(n = 30) . | APOE ε3/3(n = 30) . | APOE ε4/4(n = 10) . | APOE ε3/4(n = 10) . | APOE ε3/3(n = 10) . |
---|---|---|---|---|---|
Female/male . | 19/11 . | 18/12 . | 9/1 . | 7/3 . | 8/2 . |
Age | 65.3 (7.9) | 66.6 (8.3) | 61.2 (9.4) | 65.0 (8.5) | 64 (11.1) |
Range | 49–74 | 49–79 | 49–74 | 49–74 | 50–79 |
Education (years) | 10.6 (3.5) | 10.2 (3.3) | 11.7 (3.1) | 10.7 (4.0) | 11.8 (3.1) |
Range | 6–17 | 6–16 | 8–16 | 6–17 | 9–16 |
MMSE | 28.2 (1.5) | 27.9 (1.7) | 28.5 (1.4) | 28.4 (1.4) | 28.1 (2.1) |
Range | 24–30 | 24–30 | 26–30 | 26–30 | 24–30 |
MMSEb (2 years later) | 28.3 (1.5) | 28.3 (1.6) | 27.9 (2.0) | 28.7 (1.1) | 28.3 (1.6) |
Range | 25–30 | 26–30 | 25–30 | 27–30 | 26–30 |
SRB | 25.0 (2.4) | 22.6 (4.8) | 23.7 (2.8) | 25.3 (2.4) | 23.2 (3.8) |
Range | 16–29 | 11–29 | 16–26 | 22–29 | 17–28 |
SRBb (2 years later) | 24.9 (2.5) | 23.4 (4.5) | 24.3 (3.2) | 25.3 (1.8) | 23.4 (4.5) |
Range | 18–28 | 14–28 | 18–27 | 23–28 | 14–28 |
AD in family (N) | 2 | 0 | 0 | 1 | 0 |
Means and standard deviations (in parentheses).
MMSE = Mini-Mental State Examination (maximum = 30). SRB = word comprehension (maximum = 30). AD in family = first-degree family history of AD. The three right-most columns represent the matched subgroups.
Carriers of at least one copy of the APOE ε4 allele: 10 with APOE ε4/4; 20 with APOE ε3/4;
follow-up test; ∼2 years after fMRI-testing.
. | APOE ε4a(n = 30) . | APOE ε3/3(n = 30) . | APOE ε4/4(n = 10) . | APOE ε3/4(n = 10) . | APOE ε3/3(n = 10) . |
---|---|---|---|---|---|
Female/male . | 19/11 . | 18/12 . | 9/1 . | 7/3 . | 8/2 . |
Age | 65.3 (7.9) | 66.6 (8.3) | 61.2 (9.4) | 65.0 (8.5) | 64 (11.1) |
Range | 49–74 | 49–79 | 49–74 | 49–74 | 50–79 |
Education (years) | 10.6 (3.5) | 10.2 (3.3) | 11.7 (3.1) | 10.7 (4.0) | 11.8 (3.1) |
Range | 6–17 | 6–16 | 8–16 | 6–17 | 9–16 |
MMSE | 28.2 (1.5) | 27.9 (1.7) | 28.5 (1.4) | 28.4 (1.4) | 28.1 (2.1) |
Range | 24–30 | 24–30 | 26–30 | 26–30 | 24–30 |
MMSEb (2 years later) | 28.3 (1.5) | 28.3 (1.6) | 27.9 (2.0) | 28.7 (1.1) | 28.3 (1.6) |
Range | 25–30 | 26–30 | 25–30 | 27–30 | 26–30 |
SRB | 25.0 (2.4) | 22.6 (4.8) | 23.7 (2.8) | 25.3 (2.4) | 23.2 (3.8) |
Range | 16–29 | 11–29 | 16–26 | 22–29 | 17–28 |
SRBb (2 years later) | 24.9 (2.5) | 23.4 (4.5) | 24.3 (3.2) | 25.3 (1.8) | 23.4 (4.5) |
Range | 18–28 | 14–28 | 18–27 | 23–28 | 14–28 |
AD in family (N) | 2 | 0 | 0 | 1 | 0 |
. | APOE ε4a(n = 30) . | APOE ε3/3(n = 30) . | APOE ε4/4(n = 10) . | APOE ε3/4(n = 10) . | APOE ε3/3(n = 10) . |
---|---|---|---|---|---|
Female/male . | 19/11 . | 18/12 . | 9/1 . | 7/3 . | 8/2 . |
Age | 65.3 (7.9) | 66.6 (8.3) | 61.2 (9.4) | 65.0 (8.5) | 64 (11.1) |
Range | 49–74 | 49–79 | 49–74 | 49–74 | 50–79 |
Education (years) | 10.6 (3.5) | 10.2 (3.3) | 11.7 (3.1) | 10.7 (4.0) | 11.8 (3.1) |
Range | 6–17 | 6–16 | 8–16 | 6–17 | 9–16 |
MMSE | 28.2 (1.5) | 27.9 (1.7) | 28.5 (1.4) | 28.4 (1.4) | 28.1 (2.1) |
Range | 24–30 | 24–30 | 26–30 | 26–30 | 24–30 |
MMSEb (2 years later) | 28.3 (1.5) | 28.3 (1.6) | 27.9 (2.0) | 28.7 (1.1) | 28.3 (1.6) |
Range | 25–30 | 26–30 | 25–30 | 27–30 | 26–30 |
SRB | 25.0 (2.4) | 22.6 (4.8) | 23.7 (2.8) | 25.3 (2.4) | 23.2 (3.8) |
Range | 16–29 | 11–29 | 16–26 | 22–29 | 17–28 |
SRBb (2 years later) | 24.9 (2.5) | 23.4 (4.5) | 24.3 (3.2) | 25.3 (1.8) | 23.4 (4.5) |
Range | 18–28 | 14–28 | 18–27 | 23–28 | 14–28 |
AD in family (N) | 2 | 0 | 0 | 1 | 0 |
Means and standard deviations (in parentheses).
MMSE = Mini-Mental State Examination (maximum = 30). SRB = word comprehension (maximum = 30). AD in family = first-degree family history of AD. The three right-most columns represent the matched subgroups.
Carriers of at least one copy of the APOE ε4 allele: 10 with APOE ε4/4; 20 with APOE ε3/4;
follow-up test; ∼2 years after fMRI-testing.
All participants were right-handed, native Swedish speakers and had no reported neurological problems. Vision was normal or corrected to near normal using scanner-compatible glasses or contact lenses. Subjects were paid for participation, and informed consent was obtained in accordance with the guidelines of the Swedish Council for Research in the Humanities and Social Sciences.
APOE genotyping
A PCR was performed using 200 ng of genomic DNA as template in a 25 ml reaction mixture containing 20 pmol of PCR primers APOE-A (5′-TCC-AAG-GAG-CTG-CAG-GCG-GCG-CA-3′) and APOE-B (5′-ACA-GAA-TTC-GCC-CCG-GCC-TGG-TAC-ACT-GCC-A-3′) (Wenham et al., 1991), 0.2 U of Taq DNA polymerase (GibcoBRL, Gaithersburg, MD), 1.0 mM MgCl2, 75 mM Tris–HCl (pH 9.0), 20 mM (NH4)2SO4 and 10% dimethyl sulphoxide. The PCR amplification consisted of 35 cycles of 30 s at 94°C, 30 s at 65°C and 30 s at 72°C. PCR products were digested using 5 U of HhaI (Life Technologies, Rockville, MD) by incubating for 3 h at 37°C. Bands were separated on a 5% agarose gel and visualized on an ultraviolet transilluminator after ethidium bromide staining. Alternatively, electrophoresis was performed using ExcellGel gels (Pharmacia, Piscataway, NJ) and the MultiphorII electrophoresis system (Pharmacia), and the bands were visualized by silver staining.
Procedure
Functional MRI was used to assess brain responses while participants performed a semantic categorization task (abstract or concrete) that promoted incidental encoding of a word list, containing in total 160 words. Eighty of the words were familiarized before functional scanning by letting the subjects make abstract/concrete decisions on them: the first time outside the scanner and the second time (15–20 min later) inside the scanner during the collection of structural scans. The word order was shifted across presentations. Responses were given by pressing one of two buttons, using the right index and middle finger. During functional scanning, a blocked-task paradigm was used, altering between the experimental (‘categorization’) condition (30 s) and baseline (‘fixation’) condition (21 s) (Demb et al., 1995; Wagner et al., 1998, 2000). We used a block design to maximize statistical power and hence our chances of detecting APOE-related influences on patterns of brain activity (Birn et al., 2002; Hariri et al., 2003). During fixation, subjects viewed a cross-hair constantly displayed on the centre of the screen. Each run started and ended with brief fixation blocks (12 s). Four runs were used and they consisted of four categorization blocks containing 10 words each: either 10 familiar words (presented twice before) or 10 novel words (presented for the first time).
Subjects' behavioural performance was recorded for response reaction times and categorization accuracy. In addition, a self-paced yes/no surprise recognition test was administered 15–20 min after the scanning session, in which participants indicated whether they saw a new or a previously studied word. In all, subjects made recognition decisions on 240 words: 80 familiar (studied three times before: two times before fMRI scanning and once during scanning), 80 novel (studied once before: during fMRI scanning) and 80 new (not studied before during test phase), presented in mixed order.
During all sessions, the same words were presented in the same order to all subjects.
Imaging methods
Images were collected using a 1.5 T Philips Intera scanner (Philips Medical Systems, Netherlands) equipped for echo-planar imaging (EPI). A T2*-weighted single-shot gradient echo EPI sequence was used to acquire blood-oxygen-level-dependent (BOLD) contrast images. The following parameters were used: repetition time: 3000 ms (33 slices acquired), echo time: 50 ms, flip angle: 90°, field of view: 22 × 22 cm2, 64 × 64 matrix and 3.9 mm slice thickness. To avoid signals arising from progressive saturation, five dummy scans were performed before image acquisition. In the scanner, cushions and headphones were used to reduce movement, dampen scanner noise and communicate with the participant. Stimuli were displayed on a projection screen at the head of the bore, viewed by the subjects from within the magnet via a tilted mirror placed on the head coil. Words were presented on the screen at a frequency of one every 3 s, centred in lower case letters in white 60-point Courier New font on black background. Word presentation and registration of reaction time data were handled by a PC running E-Prime 1.0 (Psychology Software Tools, PA, USA). Responses were collected with a fibre-optic response box held in the right hand (Lumitouch reply system, Lightwave Medical Industries, Canada). High-resolution T1- and T2-weighted structural images were also acquired. The total time in the MRI scanner was ∼75 min/subject.
Data analyses
All images were sent to a PC and converted to Analyze format. Functional images were pre-processed and analysed using SPM99 (Friston et al., 1995) (Wellcome Department of Cognitive Neurology, UK, http://www.fil.ion.ucl.ac.uk) implemented in Matlab 6.1 (Mathworks Inc, MA). Before analysis, all images were realigned to the first image volume acquired. The functional images were subsequently spatially normalized and transformed into a common space, as defined by the SPM99 MNI EPI template (Evans et al., 1993), and finally spatially smoothed using a 6.0-mm full-width at half-maximum Gaussian filter kernel. Single-subject statistical contrasts were set up using the general linear model. Each condition was modelled as a fixed response (box-car) waveform convolved with the haemodynamic response function. The baseline condition was implicitly modelled for all comparisons. Statistical parametric maps (SPMs) were generated using t-statistics to identify regions activated according to the model. Group comparisons were investigated with a random-effects model [ANOVA (analysis of variance)] including the factors task (categorization versus baseline or novel versus familiar) and genotype (APOE ε3/3 versus APOE ε4). For the whole-brain comparisons, we report as significant local maxima that belonged to supra-threshold clusters defined by a voxel-level threshold of P < 0.001 (uncorrected). An extent threshold of 50 contiguous voxels was used for comparisons without prior anatomical hypothesis (i.e. categorization versus baseline). Peak locations are expressed in coordinates according to MNI space (SPM99). In addition, given our prior anatomical hypothesis regarding the medial temporal lobe (MTL) region (cf. Introduction), we investigated group differences in processing of novel and familiar items at high sensitivity by defining a spherical search volume centred at (x, y, z) = (±28, −22, −16) with a radius of 16 mm (in order to cover the hippocampal area). The P-values were corrected for multiple non-independent comparisons [family-wise error (FWE) at P < 0.05] based on a small-volume-correction (SVC).
In order to further characterize the regions in which the response differed between groups as a function of genotype, we used the SPM region-of-interest (ROI) toolbox (http://sourceforge.net/projects/spm-toolbox) to investigate the dose-related response pattern relative baseline in each subgroup (i.e. APOE ε4/4 versus APOE ε3/4 versus APOE ε3/3) in the supra-threshold clusters (i.e. the cluster mean level of activation). We also characterized the MTL results using this approach as outlined in the Results section.
Results
Behavioural data
Behavioural data are summarized in Table 2. For analyses of behavioural data, the significance level was set to P < 0.05 (Student's t-test, two-tailed). Both groups were accurate in classifying words as abstract or concrete, and there was no significant between-group difference. Nor were there any significant correlations between task performance and fMRI BOLD responses for either of the APOE groups (P ≥ 0.10). The response time data revealed a significant (P < 0.01) priming effect (Tulving and Schacter, 1990) for both groups; that is, both groups needed less time to categorize familiar compared with novel words. There were no between-group differences for the priming effect or for the total response time (novel and familiar words). Finally, two subjects did not complete the post-scan recognition test; hence these results were based on 58 subjects only. There was a non-significant tendency for a higher proportion of false alarms for APOE ε4 carriers (P = 0.10), but the recognition data (hits minus false alarms) revealed no significant difference between the groups. Collectively, these results indicate that both groups were cognitively well functioning and well matched. Subgroup comparisons yielded similar results, thus confirming that the homozygous APOE ε4 carriers had equal performance compared with heterozygous and non-carriers.
. | APOE ε4 . | APOE ε3/3 . | Pa . | APOE ε4/4 . | APOE ε3/4 . | APOE ε3/3 . |
---|---|---|---|---|---|---|
Word classification accuracy (%) | 97.2 (2.7) | 94.6 (8.9) | 0.14 | 95.7 (3.5) | 97.4 (2.3) | 89.1 (14.1) |
RT difference: novel—familiar (ms) | 111 (49) | 116 (63) | 0.74 | 113 (62) | 118 (43) | 125 (81) |
RT total: novel and familiar (ms) | 2184 (217) | 2194 (360) | 0.89 | 2174 (300) | 2258 (169) | 2279 (479) |
Recognition test (hits—false alarms) (%) | 61.5 (11.0) | 62.5 (13.2) | 0.77 | 62.3 (6.1) | 65.8 (9.8) | 61.0 (11.0) |
. | APOE ε4 . | APOE ε3/3 . | Pa . | APOE ε4/4 . | APOE ε3/4 . | APOE ε3/3 . |
---|---|---|---|---|---|---|
Word classification accuracy (%) | 97.2 (2.7) | 94.6 (8.9) | 0.14 | 95.7 (3.5) | 97.4 (2.3) | 89.1 (14.1) |
RT difference: novel—familiar (ms) | 111 (49) | 116 (63) | 0.74 | 113 (62) | 118 (43) | 125 (81) |
RT total: novel and familiar (ms) | 2184 (217) | 2194 (360) | 0.89 | 2174 (300) | 2258 (169) | 2279 (479) |
Recognition test (hits—false alarms) (%) | 61.5 (11.0) | 62.5 (13.2) | 0.77 | 62.3 (6.1) | 65.8 (9.8) | 61.0 (11.0) |
Means and standard deviations (in parentheses). The three right-most columns represent the matched subgroups. RT = response time.
Student's t-test, two-tailed.
. | APOE ε4 . | APOE ε3/3 . | Pa . | APOE ε4/4 . | APOE ε3/4 . | APOE ε3/3 . |
---|---|---|---|---|---|---|
Word classification accuracy (%) | 97.2 (2.7) | 94.6 (8.9) | 0.14 | 95.7 (3.5) | 97.4 (2.3) | 89.1 (14.1) |
RT difference: novel—familiar (ms) | 111 (49) | 116 (63) | 0.74 | 113 (62) | 118 (43) | 125 (81) |
RT total: novel and familiar (ms) | 2184 (217) | 2194 (360) | 0.89 | 2174 (300) | 2258 (169) | 2279 (479) |
Recognition test (hits—false alarms) (%) | 61.5 (11.0) | 62.5 (13.2) | 0.77 | 62.3 (6.1) | 65.8 (9.8) | 61.0 (11.0) |
. | APOE ε4 . | APOE ε3/3 . | Pa . | APOE ε4/4 . | APOE ε3/4 . | APOE ε3/3 . |
---|---|---|---|---|---|---|
Word classification accuracy (%) | 97.2 (2.7) | 94.6 (8.9) | 0.14 | 95.7 (3.5) | 97.4 (2.3) | 89.1 (14.1) |
RT difference: novel—familiar (ms) | 111 (49) | 116 (63) | 0.74 | 113 (62) | 118 (43) | 125 (81) |
RT total: novel and familiar (ms) | 2184 (217) | 2194 (360) | 0.89 | 2174 (300) | 2258 (169) | 2279 (479) |
Recognition test (hits—false alarms) (%) | 61.5 (11.0) | 62.5 (13.2) | 0.77 | 62.3 (6.1) | 65.8 (9.8) | 61.0 (11.0) |
Means and standard deviations (in parentheses). The three right-most columns represent the matched subgroups. RT = response time.
Student's t-test, two-tailed.
Differences in brain activity during categorization as a function of genetic risk
Figure 1A and B display the brain areas that were significantly activated during categorization relative to baseline in each group. The patterns of activations were highly similar and showed increased neuronal responses in visual, motor and frontal regions, mainly in the left hemisphere (Table 3).
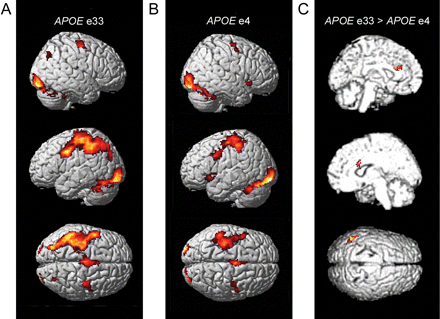
Main effects for categorization versus baseline in (A) APOE ε3/3 carriers (n = 30) and in (B) APOE ε4 carriers (n = 30). There is an apparent difference between groups in left parietal cortex that was confirmed in the group contrast depicted in (C). Additionally, in the group comparison, differences were seen in the anterior cingulate region, bilaterally.
APOE group . | Brain region . | BA . | x . | y . | z . | Z . |
---|---|---|---|---|---|---|
ε3/3 | Inferior occipital | 18 | 22 | −92 | −18 | 6.73 |
18 | −28 | −92 | −18 | 6.17 | ||
Superior occipital | 19 | 28 | −68 | 42 | 4.55 | |
Primary motor | 6 | −44 | 2 | 38 | 6.41 | |
6 | 42 | −14 | 58 | 4.08 | ||
Inferior parietal | 40 | −44 | −46 | 38 | 5.07 | |
Supplementary motor | 6 | −4 | −12 | 58 | 5.05 | |
6 | −8 | −14 | 76 | 4.00 | ||
ε4 | Inferior occipital | 18 | −30 | −94 | −8 | 7.20 |
18 | 20 | −92 | −16 | 6.54 | ||
Primary motor | 6 | −34 | −26 | 68 | 5.66 | |
6 | 50 | −4 | 54 | 4.62 | ||
Superior temporal | 38 | −52 | 18 | −8 | 5.01 | |
38 | 52 | 18 | −8 | 4.78 | ||
Thalamus | −12 | −22 | 4 | 4.29 |
APOE group . | Brain region . | BA . | x . | y . | z . | Z . |
---|---|---|---|---|---|---|
ε3/3 | Inferior occipital | 18 | 22 | −92 | −18 | 6.73 |
18 | −28 | −92 | −18 | 6.17 | ||
Superior occipital | 19 | 28 | −68 | 42 | 4.55 | |
Primary motor | 6 | −44 | 2 | 38 | 6.41 | |
6 | 42 | −14 | 58 | 4.08 | ||
Inferior parietal | 40 | −44 | −46 | 38 | 5.07 | |
Supplementary motor | 6 | −4 | −12 | 58 | 5.05 | |
6 | −8 | −14 | 76 | 4.00 | ||
ε4 | Inferior occipital | 18 | −30 | −94 | −8 | 7.20 |
18 | 20 | −92 | −16 | 6.54 | ||
Primary motor | 6 | −34 | −26 | 68 | 5.66 | |
6 | 50 | −4 | 54 | 4.62 | ||
Superior temporal | 38 | −52 | 18 | −8 | 5.01 | |
38 | 52 | 18 | −8 | 4.78 | ||
Thalamus | −12 | −22 | 4 | 4.29 |
Peak locations are expressed in MNI coordinates and an approximate anatomical region is given for each peak; statistical criteria: P < 0.001, uncorrected; k > 50 voxels.
APOE group . | Brain region . | BA . | x . | y . | z . | Z . |
---|---|---|---|---|---|---|
ε3/3 | Inferior occipital | 18 | 22 | −92 | −18 | 6.73 |
18 | −28 | −92 | −18 | 6.17 | ||
Superior occipital | 19 | 28 | −68 | 42 | 4.55 | |
Primary motor | 6 | −44 | 2 | 38 | 6.41 | |
6 | 42 | −14 | 58 | 4.08 | ||
Inferior parietal | 40 | −44 | −46 | 38 | 5.07 | |
Supplementary motor | 6 | −4 | −12 | 58 | 5.05 | |
6 | −8 | −14 | 76 | 4.00 | ||
ε4 | Inferior occipital | 18 | −30 | −94 | −8 | 7.20 |
18 | 20 | −92 | −16 | 6.54 | ||
Primary motor | 6 | −34 | −26 | 68 | 5.66 | |
6 | 50 | −4 | 54 | 4.62 | ||
Superior temporal | 38 | −52 | 18 | −8 | 5.01 | |
38 | 52 | 18 | −8 | 4.78 | ||
Thalamus | −12 | −22 | 4 | 4.29 |
APOE group . | Brain region . | BA . | x . | y . | z . | Z . |
---|---|---|---|---|---|---|
ε3/3 | Inferior occipital | 18 | 22 | −92 | −18 | 6.73 |
18 | −28 | −92 | −18 | 6.17 | ||
Superior occipital | 19 | 28 | −68 | 42 | 4.55 | |
Primary motor | 6 | −44 | 2 | 38 | 6.41 | |
6 | 42 | −14 | 58 | 4.08 | ||
Inferior parietal | 40 | −44 | −46 | 38 | 5.07 | |
Supplementary motor | 6 | −4 | −12 | 58 | 5.05 | |
6 | −8 | −14 | 76 | 4.00 | ||
ε4 | Inferior occipital | 18 | −30 | −94 | −8 | 7.20 |
18 | 20 | −92 | −16 | 6.54 | ||
Primary motor | 6 | −34 | −26 | 68 | 5.66 | |
6 | 50 | −4 | 54 | 4.62 | ||
Superior temporal | 38 | −52 | 18 | −8 | 5.01 | |
38 | 52 | 18 | −8 | 4.78 | ||
Thalamus | −12 | −22 | 4 | 4.29 |
Peak locations are expressed in MNI coordinates and an approximate anatomical region is given for each peak; statistical criteria: P < 0.001, uncorrected; k > 50 voxels.
Although most of the regions were activated to a comparable extent in both groups, a direct contrast (APOE ε3/3 > APOE ε4) showed significantly higher activation in the APOE ε3/3 carriers of the left inferior parietal cortex [Fig. 1C; Brodmann area (BA) 39, (−44, −56, 36), Z = 4.31]. In addition, differences were observed bilaterally in the anterior cingulate region [(22, 12, 26), Z = 4.33, and (−18, 30, 24), Z = 4.01]. The reverse contrast (APOE ε4 > APOE ε3/3) showed weaker differences, and the most prominent effect was localized to the right occipital cortex [BA 17, (10, −98, 2), Z = 3.85].
The dose-related effects were further investigated in the three regions that showed significant group differences (APOE ε3/3 > APOE ε4). A positive APOE dose–effect response was observed in the parietal region (Fig. 2) and no significant effect was observed in the two frontal regions (P > 0.1).
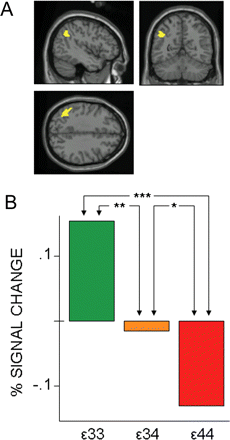
Dose-dependency of the APOE ε4 in the activation pattern: subgroup (n = 10) comparison of left parietal response. (A) The anatomical search was constrained by means of a functional ROI derived from the group contrast (Fig. 1C). (B) The dose of APOE ε4 predicted the failure to recruit the left parietal region (BA 39), (x = −44, y = −56, z = 36). In bar graph, *P < 0.1; **P < 0.05; ***P < 0.01 (one-tailed).
Differences in novelty/familiarity responses as a function of genetic risk
The group (APOE ε3/3 versus APOE ε4) by novel/familiar interaction revealed a significant effect in the MTL [right hippocampus, (30, −30, −8), Z = 3.83, P = 0.038, SVC FWE corrected; Fig. 3A]. The mean supra-threshold cluster effects for each group are plotted in Fig. 3B. While the APOE ε3/3 group expressed the expected difference in MTL response as a function of stimulus familiarity (novel > familiar), the APOE E4 group showed an effect in the opposite direction (Fig. 3B). In the novel item condition the APOE ε3/3 group showed a signal increase relative to baseline, whereas the APOE ε4 group showed a relative decrease (group difference: P = 0.002, two-tailed). In the familiar item condition the reverse was the case (group difference: P = 0.05, two-tailed). There was no support for a dose effect in the hippocampal area.

Differences in brain response to novel versus familiar items. (A) The group comparison APOE ε3/3 (n = 30) versus APOE ε4 (n = 30) revealed a significant interaction between group and the novel versus familiar contrast in a right-sided hippocampal region, (x = 30, y = −30, z = −8). (B) Functional ROI analyses revealed that only the APOE ε3/3 group showed the expected reduction in hippocampal activity during processing of familiar compared with novel items whereas the pattern was reversed in the APOE ε4 group. In bar graph, y-axis in arbitrary unit: MR signal change was computed relative to baseline and then compared for novel versus familiar item processing; **P < 0.05; ***P < 0.01 (two-tailed).
Group differences in relation to chronological age
To rule out that any observed difference in functional brain activity was driven by the older participants who potentially could have been in a preclinical stage of Alzheimer's disease, we excluded all subjects over age 70 (5 ε4 carriers and 8 ε3 carriers) and repeated all fMRI data analyses a second time. All group differences reported above were reproduced.
Discussion
A main finding of the present study was that cognitively intact carriers of the APOE ε4 allele had reduced functional brain activity in response to a semantic categorization task, compared with closely matched non-carriers. Reductions were seen in the left inferior parietal cortex and bilaterally in the anterior cingulate region. A dose-related response was observed in the parietal cortex such that homozygous carriers of the risk allele exhibited greater reduction than heterozygous carriers, which strengthens the association between reduced activity in this brain region and risk for Alzheimer's disease (Corder et al., 1993, 1994). In several previous studies, the left parietal region has been associated with the Alzheimer's disease diagnosis and its associated preclinical dysfunction. For example, Boxer et al. (2003) showed that atrophy in patients diagnosed with Alzheimer's disease was most pronounced in this region. Also, it has been frequently shown that the inferior parietal cortex is one brain region where Alzheimer's disease patients have abnormally low rates of cerebral glucose metabolism (Frackowiak et al., 1981; Smith et al., 1992; Mielke et al., 1994; Ibanez et al., 1998; Alexander et al., 2002)—findings that have been replicated in studies of cognitively normal elderly (Small et al., 1995, 2000; Reiman et al., 1996) and younger (Reiman et al., 2004) APOE ε4 carriers. Moreover, functional activation studies have demonstrated reduced task-related parietal activity responses in early clinical Alzheimer's disease patients compared with healthy elderly adults (Bäckman et al., 1999; Kato et al., 2001; Grossman et al., 2003). The left inferior parietal cortex and also the anterior cingulate cortex have been associated with semantic category judgements (Grossman et al., 2002). Notably, this ability is routinely impaired in patients with Alzheimer's disease (Chan et al., 1993, 1997; Grossman et al., 2001). Thus, our observations of differences in the left parietal and possibly also anterior cingulate regions in individuals at increased risk for Alzheimer's disease are in good agreement with several related lines of evidence.
In addition, this study extends previous findings by demonstrating a genetic dose effect on the fMRI BOLD response in the parietal area. Similarly, Reiman et al. (2005) recently reported a significant correlation between APOE ε4 gene dose and resting state glucose hypometabolism in the left parietotemporal area (BA 39).
Since the hippocampus and related medial temporal regions are among the earliest to show Alzheimer's disease-related pathological signs (Braak and Braak, 1997; Price and Morris, 1999; Fox et al., 2001; Scahill et al., 2002), a major goal of this study was to examine differences in hippocampal responding in relation to APOE isoforms. In keeping with related work (Hariri et al., 2003) we compared processing of novel versus familiar items to reveal differential activation of the hippocampal region. We found a typical reduction in hippocampal activity during processing of familiar compared with novel items in the APOE ε3/3 group, whereas the pattern was reversed in APOE ε4 carriers.
Previous functional imaging studies have found reduced task-related activity in medial temporal brain regions in Alzheimer's disease patients (Bäckman et al., 1999) and in those at risk (Elgh et al., 2003). These findings are consistent with morphological evidence of early hippocampal pathology in the course of the disease. In an fMRI study by Weiss et al. (2004), schizophrenic patients with hippocampal atrophy showed significantly reduced right hippocampal response to novel (but not familiar) verbal stimuli. Similarly, Grunwald et al. (1998) found that hippocampal damage in epilepsy patients selectively reduced event-related potentials in the medial temporal region to new but not old verbal stimuli. Also notable in this context is that the APOE ε4 allele has been associated with more prominent hippocampal atrophy compared with other APOE isoforms, in Alzheimer's disease patients (Lehtovirta et al., 1995) as well as in non-demented subjects (Soininen et al., 1995; Tohgi et al., 1997; Cohen et al., 2001; den Heijer et al., 2002). Tohgi et al. (1997) found partial-volume loss in APOE ε4 carriers as young as in their 40s, mainly in the right hippocampus. Together with these previous findings, our observation of an altered response in the right hippocampus for APOE ε4 carriers might be interpreted as reflecting early hippocampal pathology in individuals at risk for Alzheimer's disease, although disease-unrelated genetic variation cannot be ruled out as a source of variability in hippocampal responses (cf. Hariri et al., 2003).
The analyses provided limited support for increased functional brain activity in subjects at genetic risk for developing Alzheimer's disease. The only area showing a relatively increased response in APOE ε4 carriers during the categorization task was an occipital region. This is consistent with previous studies (Grady et al., 1993; Kato et al., 2001; Elgh et al., 2003), but at variance with results and conclusions reported by Bookheimer et al. (2000) that genetic risk is associated with compensatory activity in frontal, hippocampal and temporal regions. There are several possible explanations for this inconsistency. For example, the cognitive task used during the scanning session may play a role. Bookheimer et al. (2000) used a relatively demanding task (to memorize and recall unrelated pairs of words) and observed the greatest differences during periods of recall. We, on the other hand, used a fairly simple task (semantic categorization) and studied incidental encoding. In a follow-up study on the same participants as in the Bookheimer et al. (2000) study, Burggren et al. (2002) found no differences related to genetic status when a digit span task was used. Thus, the level of difficulty in a task may interact with the possibility of detecting group differences that can be attributed to the genetic profile. Here, it is relevant to note that there were no correlations between word categorization accuracy and brain activity in either group in the present study. Another factor is the cognitive status of participants. In our study the APOE ε4 carriers had cognitive test results that were indistinguishable from their matched non-carrier counterparts, whereas the APOE ε4 carriers in the Bookheimer et al. (2000) study performed worse than controls on a delayed recall test and also showed a significant decline in memory performance at a 2-year-later follow-up test. Conceivably, compensatory processes and associated brain activity come into play when the actual disease process has begun, whereas genetic risk in symptom-free individuals mainly translates into reduced activity in regions pertinent to Alzheimer's disease (cf. Reiman et al., 2004).
In conclusion, we have demonstrated a relationship between APOE ε4 and altered neuronal activity response in regions pertinent to Alzheimer's disease. The observed differences between APOE ε4 carriers and non-carriers occurred despite the fact that the two groups were well functioning and indistinguishable on classification accuracy and latency as well as on episodic recognition performance and word comprehension. Thus, our findings indicate that changes in task-related brain responses appear before detection of accompanying alterations at the behavioural level.
This work was supported by grants from the Bank of Sweden Tercentenary Foundation (J2001-0683:3), the Swedish Research Council (2003-5810) and Fund for Scientific Research Flanders (FWO). The Betula Study from where subjects were recruited is funded by the Bank of Sweden Tercentenary Foundation (1988-0082:17, J2001-0682), Swedish Council for Planning and Coordination of Research (D1988-0092, D1989-0115, D1990-0074, D1991-0258, D1992-0143, D1997-0756, D1997-1841, D1999-0739, B1999-474), Swedish Council for Research in the Humanities and Social Sciences (F377/1988-2000), the Swedish Council for Social Research (1988-1990: 88-0082, and 311/1991-2000) and the Swedish Research Council (2001-6654, 2002-3794, 2003-3883). Funding to pay the Open Access publication charges for this article was provided by the Swedish Research Council.
References
Alexander GE, Chen K, Pietrini P, Rapoport SI, Reiman EM. Longitudinal PET evaluation of cerebral metabolic decline in dementia: a potential outcome measure in Alzheimer's disease treatment studies.
Birn RM, Cox RW, Bandettini PA. Detection versus estimation in event-related fMRI: choosing the optimal stimulus timing.
Bäckman L, Andersson JL, Nyberg L, Winblad B, Nordberg A, Almkvist O. Brain regions associated with episodic retrieval in normal aging and Alzheimer's disease.
Bookheimer SY, Strojwas MH, Cohen MS, Saunders AM, Pericak-Vance MA, Mazziotta JC, et al. Patterns of brain activation in people at risk for Alzheimer's disease.
Boxer AL, Rankin KP, Miller BL, Schuff N, Weiner M, Gorno-Tempini ML, et al. Cinguloparietal atrophy distinguishes Alzheimer disease from semantic dementia.
Braak H, Braak E. Diagnostic criteria for neuropathologic assessment of Alzheimer's disease.
Burggren AC, Small GW, Sabb FW, Bookheimer SY. Specificity of brain activation patterns in people at genetic risk for Alzheimer disease.
Cedazo-Minguez A, Cowburn RF. Apolipoprotein E: a major piece in the Alzheimer's disease puzzle.
Chan AS, Butters N, Salmon DP, McGuire KA. Dimensionality and clustering in the semantic network of patients with Alzheimer's disease.
Chan AS, Butters N, Salmon DP. The deterioration of semantic networks in patients with Alzheimer's disease: a cross-sectional study.
Cohen RM, Small C, Lalonde F, Friz J, Sunderland T. Effect of apolipoprotein E genotype on hippocampal volume loss in aging healthy women.
Corder EH, Saunders AM, Strittmatter WJ, Schmechel DE, Gaskell PC, Small GW, et al. Gene dose of apolipoprotein E type 4 allele and the risk of Alzheimer's disease in late onset families.
Corder EH, Saunders AM, Risch NJ, Strittmatter WJ, Schmechel DE, Gaskell PC Jr, et al. Protective effect of apolipoprotein E type 2 allele for late onset Alzheimer disease.
Demb JB, Desmond JE, Wagner AD, Vaidya CJ, Glover GH, Gabrieli JD. Semantic encoding and retrieval in the left inferior prefrontal cortex: a functional MRI study of task difficulty and process specificity.
den Heijer T, Oudkerk M, Launer LJ, van Duijn CM, Hofman A, Breteler MM. Hippocampal, amygdalar, and global brain atrophy in different apolipoprotein E genotypes.
Dolan RJ, Fletcher PC. Dissociating prefrontal and hippocampal function in episodic memory encoding.
Duzel E, Habib R, Rotte M, Guderian S, Tulving E, Heinze HJ. Human hippocampal and parahippocampal activity during visual associative recognition memory for spatial and nonspatial stimulus configurations.
Elgh E, Larsson A, Eriksson S, Nyberg L. Altered prefrontal brain activity in persons at risk for Alzheimer's disease: an fMRI study.
Evans AC, Collins DL, Mills SR, Brown ED, Kelly RL, Peters TM. 3D statistical neuroanatomical models from 305 MRI volumes. In: Proceedings of the IEEE-Nuclear Science Symposium and Medical Imaging Conference, San Francisco (CA);
Farrer LA, Cupples LA, Haines JL, Hyman B, Kukull WA, Mayeux R, et al. Effects of age, sex, and ethnicity on the association between apolipoprotein E genotype and Alzheimer disease. A meta-analysis. APOE and Alzheimer Disease Meta Analysis Consortium.
Folstein MF, Folstein SE, McHugh PR. ‘Mini-mental state’. A practical method for grading the cognitive state of patients for the clinician.
Fox NC, Crum WR, Scahill RI, Stevens JM, Janssen JC, Rossor MN. Imaging of onset and progression of Alzheimer's disease with voxel-compression mapping of serial magnetic resonance images.
Frackowiak RS, Pozzilli C, Legg NJ, Du Boulay GH, Marshall J, Lenzi GL, et al. Regional cerebral oxygen supply and utilization in dementia. A clinical and physiological study with oxygen-15 and positron tomography.
Fratiglioni L, De Ronchi D, Aguero-Torres H. Worldwide prevalence and incidence of dementia.
Fratiglioni L, Launer LJ, Andersen K, Breteler MM, Copeland JR, Dartigues JF, et al. Incidence of dementia and major subtypes in Europe: a collaborative study of population-based cohorts. Neurologic Diseases in the Elderly Research Group.
Friston KJ, Holmes AP, Poline JB, Grasby PJ, Williams SC, Frackowiak RS, et al. Analysis of fMRI time-series revisited.
Grady CL, Haxby JV, Horwitz B, Gillette J, Salerno JA, Gonzalez-Aviles A, et al. Activation of cerebral blood flow during a visuoperceptual task in patients with Alzheimer-type dementia.
Grossman M, Robinson K, Bernhardt N, Koenig P. A rule-based categorization deficit in Alzheimer's disease?
Grossman M, Smith EE, Koenig P, Glosser G, DeVita C, Moore P, et al. The neural basis for categorization in semantic memory.
Grossman M, Koenig P, Glosser G, DeVita C, Moore P, Rhee J, et al. Neural basis for semantic memory difficulty in Alzheimer's disease: an fMRI study.
Grundman M, Corey-Bloom J, Thal LJ. Perspectives in clinical Alzheimer's disease research and the development of antidementia drugs.
Grunwald T, Lehnertz K, Heinze HJ, Helmstaedter C, Elger CE. Verbal novelty detection within the human hippocampus proper.
Hariri AR, Goldberg TE, Mattay VS, Kolachana BS, Callicott JH, Egan MF, et al. Brain-derived neurotrophic factor val66met polymorphism affects human memory-related hippocampal activity and predicts memory performance.
Ibanez V, Pietrini P, Alexander GE, Furey ML, Teichberg D, Rajapakse JC, et al. Regional glucose metabolic abnormalities are not the result of atrophy in Alzheimer's disease.
Kato T, Knopman D, Liu H. Dissociation of regional activation in mild AD during visual encoding: a functional MRI study.
Kiehl KA, Liddle PF, Smith AM, Mendrek A, Forster BB, Hare RD. Neural pathways involved in the processing of concrete and abstract words.
Lehtovirta M, Laakso MP, Soininen H, Helisalmi S, Mannermaa A, Helkala EL, et al. Volumes of hippocampus, amygdala and frontal lobe in Alzheimer patients with different apolipoprotein E genotypes.
Lobo A, Launer LJ, Fratiglioni L, Andersen K, Di Carlo A, Breteler MM, et al. Prevalence of dementia and major subtypes in Europe: a collaborative study of population-based cohorts. Neurologic Diseases in the Elderly Research Group.
Mielke R, Herholz K, Grond M, Kessler J, Heiss WD. Clinical deterioration in probable Alzheimer's disease correlates with progressive metabolic impairment of association areas.
Nilsson L, Bäckman L, Erngrund K, Nyberg L, Adolfsson R, Bucht G, et al. The Betula Prospective Cohort Study: memory, health, and aging.
Nilsson L, Adolfsson R, Backman L, de Frias C, Molander B, Nyberg L. Betula: a prospective cohort study on memory, health and aging.
Price JL, Morris JC. Tangles and plaques in nondemented aging and ‘preclinical’ Alzheimer's disease.
Reiman EM, Caselli RJ, Yun LS, Chen K, Bandy D, Minoshima S, et al. Preclinical evidence of Alzheimer's disease in persons homozygous for the epsilon 4 allele for apolipoprotein E.
Reiman EM, Chen K, Alexander GE, Caselli RJ, Bandy D, Osborne D, et al. Functional brain abnormalities in young adults at genetic risk for late-onset Alzheimer's dementia.
Reiman EM, Chen K, Alexander GE, Caselli RJ, Bandy D, Osborne D, et al. Correlations between apolipoprotein E epsilon4 gene dose and brain-imaging measurements of regional hypometabolism.
Saunders AM, Schmader K, Breitner JC, Benson MD, Brown WT, Goldfarb L, et al. Apolipoprotein E epsilon 4 allele distributions in late-onset Alzheimer's disease and in other amyloid-forming diseases.
Saykin AJ, Johnson SC, Flashman LA, McAllister TW, Sparling M, Darcey TM, et al. Functional differentiation of medial temporal and frontal regions involved in processing novel and familiar words: an fMRI study.
Scahill RI, Schott JM, Stevens JM, Rossor MN, Fox NC. Mapping the evolution of regional atrophy in Alzheimer's disease: unbiased analysis of fluid-registered serial MRI.
Small GW. Neuroimaging and genetic assessment for early diagnosis of Alzheimer's disease.
Small GW, Mazziotta JC, Collins MT, Baxter LR, Phelps ME, Mandelkern MA, et al. Apolipoprotein E type 4 allele and cerebral glucose metabolism in relatives at risk for familial Alzheimer disease.
Small GW, Ercoli LM, Silverman DH, Huang SC, Komo S, Bookheimer SY, et al. Cerebral metabolic and cognitive decline in persons at genetic risk for Alzheimer's disease.
Smith CD, Andersen AH, Kryscio RJ, Schmitt FA, Kindy MS, Blonder LX, et al. Altered brain activation in cognitively intact individuals at high risk for Alzheimer's disease.
Smith CD, Andersen AH, Kryscio RJ, Schmitt FA, Kindy MS, Blonder LX, et al. Women at risk for AD show increased parietal activation during a fluency task.
Smith GS, de Leon MJ, George AE, Kluger A, Volkow ND, McRae T, et al. Topography of cross-sectional and longitudinal glucose metabolic deficits in Alzheimer's disease. Pathophysiologic implications.
Soininen H, Partanen K, Pitkanen A, Hallikainen M, Hanninen T, Helisalmi S, et al. Decreased hippocampal volume asymmetry on MRIs in nondemented elderly subjects carrying the apolipoprotein E epsilon 4 allele.
Strittmatter WJ, Saunders AM, Schmechel D, Pericak-Vance M, Enghild J, Salvesen GS, et al. Apolipoprotein E: high-avidity binding to beta-amyloid and increased frequency of type 4 allele in late-onset familial Alzheimer disease.
Tohgi H, Takahashi S, Kato E, Homma A, Niina R, Sasaki K, et al. Reduced size of right hippocampus in 39- to 80-year-old normal subjects carrying the apolipoprotein E epsilon4 allele.
Tulving E, Markowitsch HJ, Kapur S, Habib R, Houle S. Novelty encoding networks in the human brain: positron emission tomography data.
Wagner AD, Desmond JE, Glover GH, Gabrieli JD. Prefrontal cortex and recognition memory. Functional-MRI evidence for context-dependent retrieval processes.
Wagner AD, Koutstaal W, Maril A, Schacter DL, Buckner RL. Task-specific repetition priming in left inferior prefrontal cortex.
Weiss AP, Zalesak M, DeWitt I, Goff D, Kunkel L, Heckers S. Impaired hippocampal function during the detection of novel words in schizophrenia.
Author notes
1Department of Clinical Neuroscience, MR Research Center, Karolinska Hospital N-8, 2Stockholm Aging Research Center, 3Department of Psychology, Stockholm University, Stockholm, 4Department of Psychology, 5Department of Radiation Sciences, 6Clinical Sciences and Psychiatry, Umeå University, Umeå, Sweden and 7Department of Molecular Genetics, Flanders Interuniversity Institute for Biotechnology, University of Antwerp, Antwerpen, Belgium