-
PDF
- Split View
-
Views
-
Cite
Cite
Vivek Singh, Howard Chertkow, Jason P. Lerch, Alan C. Evans, Adrienne E. Dorr, Noor Jehan Kabani, Spatial patterns of cortical thinning in mild cognitive impairment and Alzheimer's disease, Brain, Volume 129, Issue 11, November 2006, Pages 2885–2893, https://doi.org/10.1093/brain/awl256
- Share Icon Share
Abstract
Cortical thickness is a more reliable measure of atrophy than volume due to the low variability in the cytoarchitectural structure of the grey matter. However, this more desirable measure of disease-related alterations is not fully evaluated in early dementia. The study presented here is the first to report the spatial patterns of cortical thickness in the pre-clinical stages of Alzheimer's disease, namely mild cognitive impairment (MCI). Cortical thickness measurements for 34 healthy elderly, 62 MCI and 42 Alzheimer's disease subjects were made using fully automated magnetic resonance imaging-based analysis techniques in order to determine the pattern of cortical thinning as a function of disease progression. The thickness of the cortex decreased significantly when the healthy elderly brains were compared to those with MCI, mainly in the medial temporal lobe region and in some regions of the frontal and the parietal cortices. With the progression of disease from MCI to Alzheimer's disease, a general thinning of the entire cortex with significant extension into the lateral temporal lobe was found. In all cases, the results were more pronounced in the left hemisphere. In conclusion, we have shown that there is a specific pattern in the thinning of the cortical ribbon which is in agreement with the previous histological reports. These novel findings support the notion of increased isocortical involvement with the progression of disease.
Abbreviations
Introduction
The clinical syndrome termed mild cognitive impairment (MCI) designates a group of elderly individuals with subjective and objective mild memory loss or other cognitive decline, fully functional, who do not meet the criteria for dementia. Recent clinical studies have shown that individuals diagnosed with MCI have a much greater likelihood of developing dementia compared to healthy elderly subjects, and this progression to dementia occurs at an annual rate of 6–25% (Petersen et al., 2001). A comprehensive understanding of this condition will therefore provide the key knowledge about the formation of Alzheimer's related pathology as well as providing insight into the nature of MCI itself.
Clearest demonstration of the pattern of Alzheimer's disease has relied on post-mortem histopatholology (Braak and Braak, 1991). Pathological studies of MCI are rare and do not address the need for in vivo biomarkers that are capable of predicting the progression from MCI to dementia (Bennett et al., 2005). MRI-based volumetric studies of MCI and Alzheimer's disease have become increasingly common, with a large portion of the work focusing on medial temporal structures such as the entorhinal cortex and hippocampus which have been implicated in the early stages of Alzheimer's disease (Du et al., 2001; Killiany et al., 2002; Pennanen et al., 2004; Rusinek et al., 2004; Toledo-Morrell et al., 2004; Stoub et al., 2005). Recently, MRI-based whole-brain volumetric (Chetelat et al., 2002, 2005; Karas et al., 2003, 2004; Hirata et al., 2005) and cortical thickness techniques (Thompson et al., 2003; Lerch et al., 2005) have been employed in order to obtain a better overall picture of pathological structural changes.
The main global volumetric technique used to assess morphological change is voxel-based morphometry (VBM). In this approach tissue volume differences between populations are assessed on a voxel by voxel basis after normalizing brains to a standardized space (Ashburner and Friston, 2000). Interpreting such results however is difficult, given that the blurring stage the algorithm uses can involve structures that are in close spatial proximity but not closely anatomically connected. In addition, interpretation of activation in VBM can be difficult given that an actual physical characteristic is not measured directly (Allen et al., 2005).
Cortical thickness analysis involves analysing in vivo changes in the width of the cortical grey matter layer that covers the surface of the brain using MRI. Such techniques often involve extracting polygonal meshes of the inner and outer boundary of this layer and employing a distance metric between the surfaces to measure thickness (Fischl and Dale, 2000; Lerch and Evans, 2005; Kim et al., 2005). Alternatively, voxel-wise methods have also been employed (Chen et al., 2004; Sowell et al., 2004). Advantage of cortical thickness analysis over VBM is that the former capture a real physical quantity that is much easier to interpret and localize than the 3D activations generally seen with VBM. The localization is also improved by the fact that smoothing for thickness analysis is performed on a 2D manifold (Lerch and Evans, 2005), potentially reducing morphological artefacts with ageing brains.
Among the cortical thickness studies, a recently published article by Lerch et al. (2005), analysed thickness changes between normal elderly and Alzheimer's disease subjects, showing that pathological changes identified with histology mirror those present in cortical atrophy assessed through cortical thickness analysis. The current study builds upon this work by including individuals with MCI and analysing the thickness changes that are present in this intermediate condition in relation to healthy elderly and Alzheimer's disease subjects.
We hypothesize that thickness differences exist between the three groups and that these differences would follow a general expected pattern of thealthy elderly > tMCI > tAlzheimer's disease (where tx is the thickness value for condition x). The purpose of this study was also to evaluate the specific pattern of these changes when MCI and Alzheimer's disease subjects are compared to the healthy elderly. Since no post-mortem stereological data exists on MCI, we were unable to predict the specific pattern of cortical changes except for what is known in Alzheimer's disease. MCI being a heterogeneous group of subjects where all subjects do not progress to Alzheimer's disease, by comparing the three groups together and also by looking at the differences in cortical thinning. Moreover we expect these differences to exist in specific regions of the brain, especially in the areas that are first affected in the disease such as the temporal region.
Material and methods
A group of 124 subjects were scanned as part of a study conducted at the Jewish General Hospital/McGill University Memory Clinic, including 34 healthy, 65 individuals with MCI and 42 individuals with Alzheimer's disease. The diagnosis of MCI was based on consensus criteria of Petersen [subjective and objective evidence of decrease in short- or long-term memory, with no changes in intellect or daily functioning (Petersen et al., 2001)]. Duration of the impairment was always over 6 months and there was an objective memory decline on a psychometric measure of explicit memory, >1 SD and usually more than 1.5 SD from the age-appropriate mean. Alzheimer's disease subjects were required to meet the ADRDA-NINCDS criteria for possible or probable Alzheimer's disease (McKhann et al., 1984). Diagnosis was not pathologically confirmed for any subjects. Basic demographic information was acquired regarding the subjects and they each underwent a Mini-Mental State Examination (MMSE) as a measure of global severity (Folstein et al., 1975). The age distributions were 76 ± 7, 75 ± 6 and 76 ± 9, the MMSE distributions were 28.7 ± 1.2, 26.9 ± 2.5 and 22.2 ± 4.7, and the sex distributions were 22F/12M, 34F/26M and 21F/21M, in each case for the healthy elderly, MCI and Alzheimer's disease groups, respectively.
Image acquisition and processing
T1-weighted images were acquired for each subject with 1 mm isotropic resolution (TR = 22 ms, TE = 10 ms, and flip angle = 30°) at the McConnell Brain Imaging Centre of the Montreal Neurological Institute. The scans were then processed using a standard set of image processing algorithms developed at the BIC (Zijdenbos et al., 2002). Briefly this first entailed correcting for intensity inhomogeneity in the images using the N3 algorithm (Sled et al., 1998) and then linearly registering the images to a standardized space (Collins et al., 1994). The brain volumes were then classified into the three major brain tissues: grey matter, white matter and CSF (Zijdenbos et al., 2002). The Constrained Laplacian Anatomic Segmentation Using Proximities surface extraction procedure was then used to generate surface meshes representing the white matter and grey matter interfaces (Kim et al., 2005). The root mean square thickness between corresponding nodes on the surface meshes was then calculated (MacDonald et al., 2000). The thickness values were calculated in the MRI scanner space of the brains rather than in Talairach space which increases the amount of statistically significant findings (Ad-Dab'bagh et al., 2005). A 2D surface blurring algorithm was used to reduce noise in the thickness measurements and reduce the effective number of statistics comparisons being performed (Chung et al., 2003). The diameter of the blurring kernel was 20 mm. Finally, the thickness measurements were aligned using 2D surface registration in order to maximize thickness value correspondence between subjects in terms of gyral patterning (Robbins et al., 2004).
The two steps that differ from those used by Lerch et al. (2005), include the transformation of thickness values to native space which, as indicated, increases statistical significance. The original rationale for using standardized thickness values was to remove any confound of head size on thickness values; however, a recent study has shown no significant correlation between head size and average thickness (Ad-Dab'bagh et al., 2005). The second change was the use of the 2D non-linear registration procedure which ensures better alignment between structures in the brain and thus provides better anatomical correspondence between structures compared in the brain as shown in a recently published article (Robbins et al., 2004).
In order to identify gyral regions on the surface of the cortex, the Automatic Image Matching and Anatomical Labeling non-linear volumetric registration package was used to identify structures by registering the T1-weighted images to a probabilistic atlas (Collins et al., 1999). The labels for the structures were then transferred to the cortical surface by assigning the value of the closest voxel label to each vertex on the cortical surface.
Statistical analysis
For the statistical analysis, comparisons were carried out using mean thickness, regional thickness and vertex thickness values.
To analyse whether any difference in mean cortical thickness existed between the three groups, an analysis of co-variance (ANCOVA) was performed while controlling for the effect of age (Fig. 1). Post hoc t-tests were then performed to establish where the specific differences between the groups existed.
![Age-corrected differences in thickness among the healthy elderly (HE), MCI and Alzheimer's disease populations. Differences between the three groups are statistically significant [P < 2.2 × 10−16, F(3,142) = 38.9], while all paired differences were also significant (pHE, MCI = 0.00205, t104 = −3.098, pHE, Alzheimer's disease = 1.5 × 10−10, t80 = −7.095, pMCI, Alzheimer's disease = 2.9 × 10−5, t106 = −4.372). The graph illustrates that thickness differences are larger between the MCI and dementia groups than the healthy elderly and MCI groups.](https://oup.silverchair-cdn.com/oup/backfile/Content_public/Journal/brain/129/11/10.1093/brain/awl256/2/m_awl256f1.gif?Expires=1747923742&Signature=PewhrKZba-nwXRv6f90h9t-2Gjs452wD3SoiEuqTE1p0DTolfur-2pwX-gi0h0beyAw5i~Fi99ayRoYigA0VIPPrBGPq2CEj2Q-jJjp74l771tpdyxQe3nPb8Q-fYoSGNhEYa5QxY7eSR8n0u3HohXMzdMyDXo9JQs9i~SngGz4DGuWjTPzHUIm2vieEtXMK2TJV86it6MNVa7iG34INQ-Hf39PivPwJDKXzuyYYdlkVFQncBGq8vnx2Uw4jA3ExUvWJeWeGRAGLGhdcV372IDGH9lnlK1jgt3shFFH9JrGtEZmZBLdgkzcdq049mA7EwFnUjaBJUJV~ryN8NTjS4A__&Key-Pair-Id=APKAIE5G5CRDK6RD3PGA)
Age-corrected differences in thickness among the healthy elderly (HE), MCI and Alzheimer's disease populations. Differences between the three groups are statistically significant [P < 2.2 × 10−16, F(3,142) = 38.9], while all paired differences were also significant (pHE, MCI = 0.00205, t104 = −3.098, pHE, Alzheimer's disease = 1.5 × 10−10, t80 = −7.095, pMCI, Alzheimer's disease = 2.9 × 10−5, t106 = −4.372). The graph illustrates that thickness differences are larger between the MCI and dementia groups than the healthy elderly and MCI groups.
Thickness data were also analysed to determine cortical regional differences between healthy elderly/MCI and MCI/Alzheimer's disease groups (Table 1). Specifically, the four lobes and sub-gyri of frontal and temporal lobes were compared. This analysis included a Bonferroni correction to control for multiple comparisons. A Bonferroni correction may be quite conservative given the correlations that may exist between regional mean thickness measurements. Therefore, any differences that survive this correction will definitely be significant.
Differences in lobar thickness between the healthy elderly–MCI and MCI–Alzheimer's disease groups
Structure . | Healthy elderly versus MCI . | MCI versus Alzheimer's disease . | ||||||
---|---|---|---|---|---|---|---|---|
. | . | . | ||||||
. | Difference (mm) . | t-Stat . | P-value . | % Atrophy . | Difference (mm) . | t-Stat . | P-value . | % Atrophy . |
Temporal lobe | 0.206 | 3.633 | 0.014 | 8.3 | 0.351 | 5.586 | 0.000006 | 16.1 |
Frontal lobe | 0.197 | 3.175 | 0.064 | 8.9 | 0.252 | 3.730 | 0.0099 | 12.7 |
Parietal lobe | 0.172 | 3.239 | 0.052 | 9.1 | 0.225 | 3.931 | 0.005 | 13.4 |
Occipital lobe | 0.124 | 2.314 | 0.725 | 6.8 | 0.174 | 3.186 | 0.061 | 10.3 |
Inferior temporal gyrus | 0.233 | 3.776 | 0.006 | 9.3 | 0.396 | 6.043 | 0.000001 | 18.2 |
Middle temporal gyrus | 0.223 | 3.562 | 0.018 | 9.0 | 0.355 | 5.046 | 0.00006 | 16.4 |
Parahippocampal gyrus | 0.189 | 3.278 | 0.042 | 8.1 | 0.357 | 5.715 | 0.000002 | 17.4 |
Superior temporal gyrus | 0.177 | 2.857 | 0.155 | 7.1 | 0.340 | 5.047 | 0.00006 | 15.4 |
Lateral occipitotemporal gyrus | 0.206 | 3.869 | 0.007 | 8.5 | 0.295 | 5.075 | 0.00004 | 13.6 |
Entorhinal cortex | 0.262 | 3.524 | 0.0006 | 9.9 | 0.413 | 5.570 | 0.0000002 | 17.9 |
Medial frontal gyrus | 0.197 | 2.878 | 0.156 | 8.1 | 0.296 | 3.876 | 0.006 | 13.6 |
Superior frontal gyrus | 0.204 | 2.902 | 0.135 | 9.7 | 0.274 | 3.739 | 0.009 | 14.8 |
Medial fronto-orbital gyrus | 0.235 | 3.727 | 0.008 | 9.5 | 0.252 | 3.493 | 0.019 | 11.3 |
Lateral fronto-orbital gyrus | 0.245 | 3.390 | 0.030 | 10.3 | 0.258 | 3.457 | 0.023 | 12.2 |
Middle frontal gyrus | 0.220 | 3.294 | 0.044 | 9.9 | 0.257 | 3.541 | 0.018 | 13.1 |
Inferior frontal gyrus | 0.206 | 3.065 | 0.083 | 9.0 | 0.236 | 3.172 | 0.059 | 11.5 |
Structure . | Healthy elderly versus MCI . | MCI versus Alzheimer's disease . | ||||||
---|---|---|---|---|---|---|---|---|
. | . | . | ||||||
. | Difference (mm) . | t-Stat . | P-value . | % Atrophy . | Difference (mm) . | t-Stat . | P-value . | % Atrophy . |
Temporal lobe | 0.206 | 3.633 | 0.014 | 8.3 | 0.351 | 5.586 | 0.000006 | 16.1 |
Frontal lobe | 0.197 | 3.175 | 0.064 | 8.9 | 0.252 | 3.730 | 0.0099 | 12.7 |
Parietal lobe | 0.172 | 3.239 | 0.052 | 9.1 | 0.225 | 3.931 | 0.005 | 13.4 |
Occipital lobe | 0.124 | 2.314 | 0.725 | 6.8 | 0.174 | 3.186 | 0.061 | 10.3 |
Inferior temporal gyrus | 0.233 | 3.776 | 0.006 | 9.3 | 0.396 | 6.043 | 0.000001 | 18.2 |
Middle temporal gyrus | 0.223 | 3.562 | 0.018 | 9.0 | 0.355 | 5.046 | 0.00006 | 16.4 |
Parahippocampal gyrus | 0.189 | 3.278 | 0.042 | 8.1 | 0.357 | 5.715 | 0.000002 | 17.4 |
Superior temporal gyrus | 0.177 | 2.857 | 0.155 | 7.1 | 0.340 | 5.047 | 0.00006 | 15.4 |
Lateral occipitotemporal gyrus | 0.206 | 3.869 | 0.007 | 8.5 | 0.295 | 5.075 | 0.00004 | 13.6 |
Entorhinal cortex | 0.262 | 3.524 | 0.0006 | 9.9 | 0.413 | 5.570 | 0.0000002 | 17.9 |
Medial frontal gyrus | 0.197 | 2.878 | 0.156 | 8.1 | 0.296 | 3.876 | 0.006 | 13.6 |
Superior frontal gyrus | 0.204 | 2.902 | 0.135 | 9.7 | 0.274 | 3.739 | 0.009 | 14.8 |
Medial fronto-orbital gyrus | 0.235 | 3.727 | 0.008 | 9.5 | 0.252 | 3.493 | 0.019 | 11.3 |
Lateral fronto-orbital gyrus | 0.245 | 3.390 | 0.030 | 10.3 | 0.258 | 3.457 | 0.023 | 12.2 |
Middle frontal gyrus | 0.220 | 3.294 | 0.044 | 9.9 | 0.257 | 3.541 | 0.018 | 13.1 |
Inferior frontal gyrus | 0.206 | 3.065 | 0.083 | 9.0 | 0.236 | 3.172 | 0.059 | 11.5 |
Changes in specific gyri in the frontal and temporal lobes are presented as well. The thickness was always healthy elderly > MCI > Alzheimer's disease. The largest changes in both transitions occur in the temporal lobe, which also shows the greatest increase in atrophy. Interestingly, the fronto-orbital gyri show differences comparable to the temporal lobe structures in the transition from healthy elderly to MCI. A Bonferonni correction was performed on the P-values in order to account for multiple comparisons.
Differences in lobar thickness between the healthy elderly–MCI and MCI–Alzheimer's disease groups
Structure . | Healthy elderly versus MCI . | MCI versus Alzheimer's disease . | ||||||
---|---|---|---|---|---|---|---|---|
. | . | . | ||||||
. | Difference (mm) . | t-Stat . | P-value . | % Atrophy . | Difference (mm) . | t-Stat . | P-value . | % Atrophy . |
Temporal lobe | 0.206 | 3.633 | 0.014 | 8.3 | 0.351 | 5.586 | 0.000006 | 16.1 |
Frontal lobe | 0.197 | 3.175 | 0.064 | 8.9 | 0.252 | 3.730 | 0.0099 | 12.7 |
Parietal lobe | 0.172 | 3.239 | 0.052 | 9.1 | 0.225 | 3.931 | 0.005 | 13.4 |
Occipital lobe | 0.124 | 2.314 | 0.725 | 6.8 | 0.174 | 3.186 | 0.061 | 10.3 |
Inferior temporal gyrus | 0.233 | 3.776 | 0.006 | 9.3 | 0.396 | 6.043 | 0.000001 | 18.2 |
Middle temporal gyrus | 0.223 | 3.562 | 0.018 | 9.0 | 0.355 | 5.046 | 0.00006 | 16.4 |
Parahippocampal gyrus | 0.189 | 3.278 | 0.042 | 8.1 | 0.357 | 5.715 | 0.000002 | 17.4 |
Superior temporal gyrus | 0.177 | 2.857 | 0.155 | 7.1 | 0.340 | 5.047 | 0.00006 | 15.4 |
Lateral occipitotemporal gyrus | 0.206 | 3.869 | 0.007 | 8.5 | 0.295 | 5.075 | 0.00004 | 13.6 |
Entorhinal cortex | 0.262 | 3.524 | 0.0006 | 9.9 | 0.413 | 5.570 | 0.0000002 | 17.9 |
Medial frontal gyrus | 0.197 | 2.878 | 0.156 | 8.1 | 0.296 | 3.876 | 0.006 | 13.6 |
Superior frontal gyrus | 0.204 | 2.902 | 0.135 | 9.7 | 0.274 | 3.739 | 0.009 | 14.8 |
Medial fronto-orbital gyrus | 0.235 | 3.727 | 0.008 | 9.5 | 0.252 | 3.493 | 0.019 | 11.3 |
Lateral fronto-orbital gyrus | 0.245 | 3.390 | 0.030 | 10.3 | 0.258 | 3.457 | 0.023 | 12.2 |
Middle frontal gyrus | 0.220 | 3.294 | 0.044 | 9.9 | 0.257 | 3.541 | 0.018 | 13.1 |
Inferior frontal gyrus | 0.206 | 3.065 | 0.083 | 9.0 | 0.236 | 3.172 | 0.059 | 11.5 |
Structure . | Healthy elderly versus MCI . | MCI versus Alzheimer's disease . | ||||||
---|---|---|---|---|---|---|---|---|
. | . | . | ||||||
. | Difference (mm) . | t-Stat . | P-value . | % Atrophy . | Difference (mm) . | t-Stat . | P-value . | % Atrophy . |
Temporal lobe | 0.206 | 3.633 | 0.014 | 8.3 | 0.351 | 5.586 | 0.000006 | 16.1 |
Frontal lobe | 0.197 | 3.175 | 0.064 | 8.9 | 0.252 | 3.730 | 0.0099 | 12.7 |
Parietal lobe | 0.172 | 3.239 | 0.052 | 9.1 | 0.225 | 3.931 | 0.005 | 13.4 |
Occipital lobe | 0.124 | 2.314 | 0.725 | 6.8 | 0.174 | 3.186 | 0.061 | 10.3 |
Inferior temporal gyrus | 0.233 | 3.776 | 0.006 | 9.3 | 0.396 | 6.043 | 0.000001 | 18.2 |
Middle temporal gyrus | 0.223 | 3.562 | 0.018 | 9.0 | 0.355 | 5.046 | 0.00006 | 16.4 |
Parahippocampal gyrus | 0.189 | 3.278 | 0.042 | 8.1 | 0.357 | 5.715 | 0.000002 | 17.4 |
Superior temporal gyrus | 0.177 | 2.857 | 0.155 | 7.1 | 0.340 | 5.047 | 0.00006 | 15.4 |
Lateral occipitotemporal gyrus | 0.206 | 3.869 | 0.007 | 8.5 | 0.295 | 5.075 | 0.00004 | 13.6 |
Entorhinal cortex | 0.262 | 3.524 | 0.0006 | 9.9 | 0.413 | 5.570 | 0.0000002 | 17.9 |
Medial frontal gyrus | 0.197 | 2.878 | 0.156 | 8.1 | 0.296 | 3.876 | 0.006 | 13.6 |
Superior frontal gyrus | 0.204 | 2.902 | 0.135 | 9.7 | 0.274 | 3.739 | 0.009 | 14.8 |
Medial fronto-orbital gyrus | 0.235 | 3.727 | 0.008 | 9.5 | 0.252 | 3.493 | 0.019 | 11.3 |
Lateral fronto-orbital gyrus | 0.245 | 3.390 | 0.030 | 10.3 | 0.258 | 3.457 | 0.023 | 12.2 |
Middle frontal gyrus | 0.220 | 3.294 | 0.044 | 9.9 | 0.257 | 3.541 | 0.018 | 13.1 |
Inferior frontal gyrus | 0.206 | 3.065 | 0.083 | 9.0 | 0.236 | 3.172 | 0.059 | 11.5 |
Changes in specific gyri in the frontal and temporal lobes are presented as well. The thickness was always healthy elderly > MCI > Alzheimer's disease. The largest changes in both transitions occur in the temporal lobe, which also shows the greatest increase in atrophy. Interestingly, the fronto-orbital gyri show differences comparable to the temporal lobe structures in the transition from healthy elderly to MCI. A Bonferonni correction was performed on the P-values in order to account for multiple comparisons.
A vertex-by-vertex analysis was carried out using a univariate general linear model. Cortical thickness was regressed against diagnosis and age. Multiple comparisons were taken into account for the vertex data using a false discovery rate correction at a 0.05 level of significance (Genovese et al., 2002). This ensured that, on average, only 5% of the surviving results were false positive.
The hypothesis tested in this work was to determine whether thickness differences exist between the three groups. In addition, we wish to test whether differences found follow the general pattern of thealthy elderly > tMCI > tAlzheimer's disease (where tx is the thickness value for condition x), and where such differences exist regionally. We used one-tailed t-tests at a 5% level of significance for the vertex comparisons.
Specifically, the following vertex-by-vertex analyses were performed:
ANCOVA analysis of thickness among healthy elderly, MCI and Alzheimer's disease to identify the extent to which various cortical regions are affected with respect to the disease (Fig. 2).
Thickness differences between healthy elderly and MCI (Fig. 3).
Thickness differences between MCI and Alzheimer disease (Fig. 4).
Thickness differences between healthy elderly and Alzheimer's disease (Fig. 5).
Assessment of thickness differences in transition from healthy elderly to MCI and MCI to Alzheimer's disease (Fig. 6).
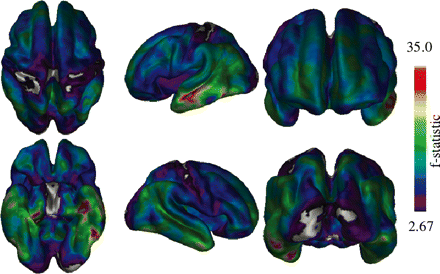
Vertex-by-vertex ANCOVA analysis of thickness data. Most regions of the brain show significant differences with the exception of the occipital lobe and some areas of the pre- and post-central gyri.
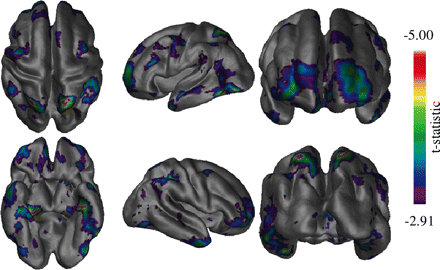
Vertex thickness differences between the healthy elderly and MCI groups. The most significant difference seen is in the left medial temporal region. The frontal and posterior parietal areas also show differences in a more bilateral fashion.
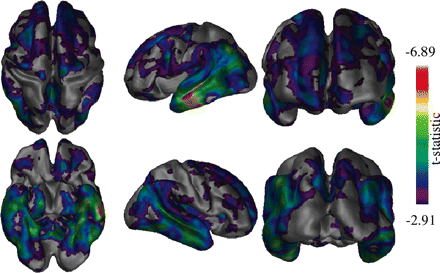
Differences between the MCI and Alzheimer's disease groups. Differences in the temporal regions are shown bilaterally as well as in the frontal lobe regions. The most pronounced differences clearly occurring in the left lateral temporal area.
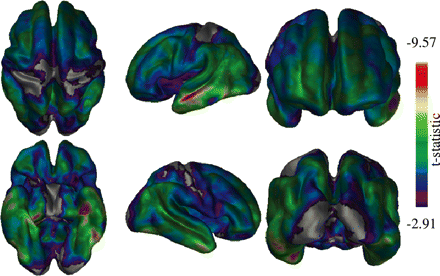
Differences between healthy elderly and Alzheimer's disease subjects across the cortex. Clear medial and lateral temporal lobe differences are present bilaterally. Occipital, primary motor and primary sensory cortices show the least significant differences.
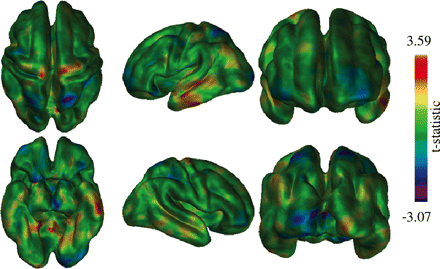
Assessment of thickness differences between the transition from healthy elderly to MCI and from MCI to Alzheimer's disease. Darker (purple/blue) areas represent areas where thickness changes from healthy elderly to MCI are larger than those from MCI to Alzheimer's disease, while lighter (red/yellow) areas represent the opposite. Note that results do not reach statistical significance, but interesting trends are apparent.
Results
Mean and region differences
An ANOVA on mean thickness revealed a statistical significance between groups [F(3,142) = 38.9, P = 2.2 × 10−16] and post hoc t-tests were significant for all comparisons (Fig. 1). As expected, the Alzheimer's disease subjects show the lowest mean thickness, while the MCI patients show an intermediate thickness value between the normal and the Alzheimer's disease subjects, though were somewhat closer in average thickness to the healthy elderly (a 0.18 mm difference) than to the MCI subjects (a 0.26 mm difference).
Table 1 shows healthy elderly–MCI and MCI–Alzheimer's disease differences in thickness calculated bilaterally across the lobes of the brain and in gyri of the temporal and frontal lobes. The largest lobar thickness difference appeared in the temporal lobe both between the healthy elderly and MCI populations as well as the MCI and Alzheimer's disease groups. This area also showed the greatest percentage difference in thickness between the conditions. The thickness changes in the other brain areas show less percentage difference among the groups, with the occipital lobe displaying the smallest difference.
The most significant thickness differences within the temporal lobe appear in the inferior and middle temporal gyri in both the healthy elderly–MCI and the MCI–Alzheimer's disease comparisons. Thickness differences in the superior temporal and parahippocampal gyri were also present between the MCI and Alzheimer's disease groups. The inferior orbitofrontal area showed a difference thinning that is comparable to that seen in the medial and inferior temporal regions between the healthy elderly and MCI groups, but did not show the thickness difference that is apparent between the MCI and Alzheimer's disease groups in most other regions, including the more superior frontal areas.
Table 1 also shows the atrophy in the entorhinal region, separated from the parahippocampal gyrus. Differences in this area were more pronounced than any of the other structures analysed and increased quite markedly in the transition from MCI to Alzheimer's disease.
Vertex-wise group differences
Figure 2 shows the ANCOVA analysis performed on the thickness data using all three groups. Thickness changes were found to be quite widespread except for the occipital lobes and pre- and post-central gyri which showed little to no differences in thickness among healthy elderly, MCI and Alzheimer's disease. The most significant differences appear in the left medial lateral temporal lobes, as indicated by the areas shown in red.
Figure 3 shows vertex-by-vertex differences in thickness between the healthy elderly and MCI groups. The most significant difference appeared in the medial temporal lobe, while differences are also seen in the frontal and superior parietal areas bilaterally. In the frontal lobe, the most significant changes appeared in the right prefrontal area. Some degree of change can also be seen bilaterally on the lateral temporal lobes. The occipital lobe however showed no significant differences.
The differences between the MCI and Alzheimer's disease groups (Fig. 4) were more generalized than those seen between the healthy elderly and MCI groups, particularly in the temporal lobe region. Although most changes were bilateral, the left lateral temporal lobe showed the greatest thickness difference, spanning the width of the middle temporal gyrus. Frontal lobe changes were also present, though parietal and occipital differences were not as apparent.
Comparing differences between healthy elderly and Alzheimer's disease subjects (Fig. 5) showed significant atrophy throughout the cortex, with the exception of the primary motor, sensory and visual areas. Atrophy in the temporal lobes was very strong particularly in the lateral regions, while medial temporal atrophy was primarily left lateralized.
In order to check for differences between the transition from healthy elderly to MCI and from MCI to Alzheimer's disease, an analysis was carried out to compare whether slope differences were present between the two transitions (Fig. 6). Although results did not reach statistical significance, clear trends are evident particularly in analysing the t-statistic map. Most cortical areas showed similar levels of atrophy in both transitions, though an increase in atrophy was evident on the left lateral temporal lobe. The changes in the medial temporal area, however, appear fairly consistent with little differences between the transitions. Other areas which showed differences include the superior parietal lobes bilaterally, which are the only cortical regions that showed a higher thickness difference in the healthy elderly–MCI transition than the MCI–Alzheimer's disease transition. Also, a greater change in the MCI–Alzheimer's disease transition was seen in the precentral gyrus, particularly on the right-hand side.
Fig. 7 illustrates thickness differences on two particular vertices chosen on the cortical surface. One vertex on the medial side of the left temporal lobe showed a gradual change in thickness among the healthy elderly, MCI and Alzheimer's disease populations, while on the lateral side, a larger difference in thickness was seen between the MCI and Alzheimer's disease groups.
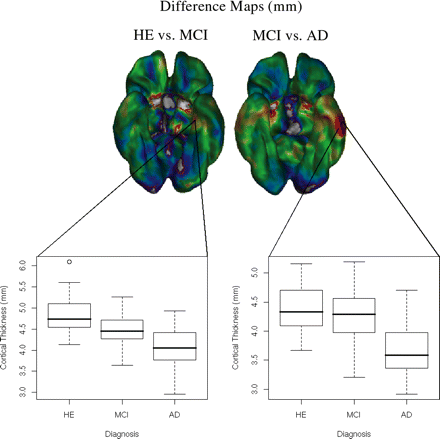
An illustration of thickness differences between the medial and lateral temporal lobe areas. The graphs illustrate the age-corrected thickness differences between groups for vertices on the medial and lateral left temporal areas. On the medial side, the cortex appears to thin gradually between the different conditions, while laterally a much more pronounced difference is seen between the MCI and Alzheimer's disease groups, than between the healthy elderly and MCI groups.
Discussion
In the course of this study we had hypothesized that the thickness of the cortex would systematically decrease from healthy elderly to MCI and will be the lowest in Alzheimer's disease. This hypothesis was based on post-mortem brain findings which have shown that in normal ageing and Alzheimer's disease cytoarchitectural changes due to processes such as neuronal loss, synaptic degeneration or cell shrinkage ultimately affect the thickness of the cortex (Henderson et al., 1980; Anderson et al., 1983; Davies et al., 1987; Terry et al., 1987; Hansen et al., 1988; Regeur et al., 1994). MRI methodology is currently the best in vivo method available for indirectly detecting the patterns of spatial distribution of the cortex (Thompson et al., 2003, 2004) which may be changing as a consequence of underlying cytoarchitectural alteration. Although it is more desirable to study such patterns over time, as carried out in previous longitudinal volumetric studies (Thompson et al., 2003), valuable information can be gained from the study presented here which is the first to describe the patterns of cortical thinning in MCI while comparing it to healthy elderly and Alzheimer's disease. Post-mortem literature on MCI (Mitchell et al., 2002; Guillozet et al., 2003; Petersen et al., 2006) is scarce due to the lack of stereological data on this population and information on cortical thickness variations in MCI is non-existent. Our findings make an important contribution towards the relationship between thickness of the cortical ribbon and the early development of the disease.
The global thickness differences seen in Fig. 1 display a decline in mean thickness with severity of the condition. While comparing these statistically, we found that the mean cortical thickness difference between healthy elderly and MCI was less pronounced than the difference between the MCI and Alzheimer's disease groups, which may bear a relationship to the much more severe clinical symptoms seen in Alzheimer's disease than in MCI, as evidenced by the 1.4 and 4.7 unit MMSE difference between the healthy elderly–MCI and the MCI–Alzheimer's disease groups, respectively. The placement of MCI in between healthy elderly and Alzheimer's disease is in accordance with the recent pathological findings on amnestic MCI, which demonstrates that MCI fall close to healthy elderly when compared on various types of plaques but were relatively advanced in the distribution of neurofibrillary tangles (Petersen et al., 2006).
The ANOVA performed on the vertex-by-vertex analysis (Fig. 2) show a generalized thickness differences between groups. The key areas that show the greatest difference include the medial and lateral left temporal lobe regions; whereas the areas that were spared included the primary motor and sensory cortices as well as parts of occipital cortex. These findings are also in accordance with neuropathological reports on both MCI and Alzheimer's disease population (Braak and Braak, 1991; Guillozet et al., 2003; Petersen et al., 2006). The paired difference analysis is able to distinguish clearly that the medial temporal lobe difference seen is primarily between healthy elderly and MCI (Fig. 3), compared to the lateral temporal lobe changes seen between MCI and Alzheimer's disease (Fig. 4).
Using VBM, Chetelat et al. (2002) revealed findings much similar to ours with medial temporal changes occurring in the healthy elderly to MCI transition, and lateral temporal changes occurring in the MCI to Alzheimer's disease transition, with both results showing left lateralization. VBM studies have also noted superior parietal changes, though primarily in the transition from MCI to Alzheimer's disease rather than healthy elderly to MCI (Chetelat et al., 2002; Karas et al., 2004).
Comparison of healthy elderly and MCI
The difference seen in the medial temporal region while comparing MCI to healthy elderly, close to the position of the entorhinal cortex, showed a strong agreement with previous MRI findings related to changes seen in MCI and Alzheimer's disease (Jack et al., 1997; Pennanen et al., 2004; Rusinek et al., 2004). Interestingly, this area has also been found to have the maximum number of neurofibrillary tangles in pathological evaluation of amnestic MCI (Guillozet et al., 2003; Petersen et al., 2006). Other areas showing differences included the prefrontal association areas, as well as the superior parietal lobes, bilaterally. The prefrontal association areas are known to play a significant role in memory function and thus changes in this region would not be unexpected (Petrides, 2005).
The findings in the parietal association cortex in MCI compared to healthy elderly could be explicable by recent work suggesting that subjects diagnosed with amnestic MCI rarely have a pure form of the condition and in fact that other subtle cognitive deficits are often present (Alladi et al., 2006). These include visuospatial (Mapstone et al., 2003) and visual attention (Tales et al., 2005) deficits that may relate to parietal lobe function. Another possibility could be that parietal region in Alzheimer's disease shows accelerated degeneration (Thompson et al., 2003) and since amnestic MCI tend to develop dementia of Alzheimer's type (Gauthier et al., 2006), what we found could be a reflection of very early stage of the disease in some fraction of this group. In terms of neuropathological findings in MCI, this area contains the highest amount of neurofibrillary tangles after the temporal lobes (Guillozet et al., 2003; Petersen et al., 2006).
One key factor in our findings is the population used. Specifically, the MCI population in our study has primarily memory problems (amnestic MCI) and most of them are likely to develop Alzheimer's disease if their conditions were to get worse (Gauthier et al., 2006). For this reason we have carried out our analysis comparing this population primarily to Alzheimer's disease and have found results as expected. It is likely that the clinics that use other criteria for MCI or do not focus primarily on amnestic MCI would find alternate results based on the type of dementia their MCI population may proceed to develop. For instance, in our unpublished analysis we have found while the cortical mantle thins all over in Alzheimer's disease, the frontotemporal dementia subjects show significant differences only in frontal and temporal regions, with the greatest differences located in prefrontal cortex, anterior cingulate cortex, and the anterior temporal lobes.
Comparison of MCI and Alzheimer's disease
Differences between the MCI and Alzheimer's disease groups showed much more diffuse isocortical involvement in the Alzheimer's disease subjects with the lateral temporal regions showing strong significance. Difference in the temporal lobes occurred bilaterally, though it was generally more pronounced in the left lobe. These findings are supported by the findings of left lateralized acceleration of grey matter degeneration in Alzheimer's disease (Thompson et al., 2003).
Clinical Alzheimer's disease is generally believed to correspond to Braak stages V–VI (Braak and Braak, 1991; Markesbery et al., 2006), where neurofibrillary changes occur across the temporal, parietal and frontal areas. The thickness results showed a similar trend, with significant changes across the entire temporal lobe, as well as changes in the frontal lobe extending posteriorly to the precentral gyrus. The parietal lobe showed less regional significance, with exceptions being in the temporoparietal and medial parietal regions.
Also in correspondence with Braak staging, the motor and sensory cortices (temporal lobe, pre- and post-central gyri) showed little significant difference. These areas are expected to be the last regions which display associations with Alzheimer's related pathological changes.
These results are borne out even further in the thickness difference seen in Fig. 6, with isocortical changes in the temporal lobes accelerating with progression from MCI to Alzheimer's disease. Also, some changes in the primary motor cortex appear to be starting in the case of the Alzheimer's disease group, but absent for the MCI subjects, suggesting that Alzheimer's related changes are invading the entire cortex.
Comparison of Alzheimer's disease and healthy elderly
In relation to the Lerch et al. (2005) study, our findings while comparing Alzheimer's disease and healthy elderly, are generally similar, though specific differences do exist. In the medial temporal region, the Lerch results showed the highest significance level near the temporal poles bilaterally, while in our study the most significant region is located more posterior, in the inferior temporal lobe and is left lateralized. In terms of lateral temporal differences, the areas of greatest significance appeared closer to the temporal pole than those seen in the Lerch study. The overall pattern of difference, however, is very similar to sensory and motor areas showing little or no significant change. The differences between the two studies may be related to a number of factors. Alzheimer's disease patients in the Lerch study were marginally more severe in terms of their MMSE scores. Nevertheless, the age distribution in the Lerch study was quite a bit younger with average ages for the healthy elderly and Alzheimer's disease populations of 61 and 68 years old, respectively, while in our study both groups had a mean age of 76. The Lerch study may thus be looking at subjects with earlier onset of Alzheimer's disease, which could have different patterns of atrophy. Differences also exist in terms of image processing, in that our study used non-linear surface registration in order to achieve better structural alignment. We also calculated thickness values in the MRI scanner space rather than Talairach space, which likely contributed to the larger statistical differences we saw (in addition to our larger sample size).
In conclusion, we have shown that there is a specific pattern in the thinning of the cortical ribbon which is in agreement with the histological findings of disease progression. Although there is a high level of variability in normal brain volume, the cortical ribbon is relatively stable due to the cytoarchitectural features of the grey matter and thus is a more desirable measure of disease-related alterations. The main advantage of measuring cortical thickness over cortical volume has recently been demonstrated in a stereological study of dementia, where cortical thickness measures were found to be the most stable estimator (coefficient of variation; CV = 8–9%) and had the closest relationship to dementia in comparison to ventricular volume (CV = 35–65%) and cortical volume (CV = 13–18%) (Regeur, 2000). Although the study measured cortical thickness indirectly, using a ratio of cortical volume and surface area, its validity is supported by another detailed post-mortem stereological human study which has shown that while a 100% increase in total number of neurons is accompanied by an 85% increase in neocortical volume, a 100% increase in cortical volume only results in a 23% increase in cortical thickness, thereby indicating that cortical thickness is a more stable parameter in both males and females with a CV of 10% compared with neocortical volume that has a CV of 16% (Pakkenberg and Gundersen, 1997). Moreover, the fact that excessive cortical expansion during the development is not accompanied by comparable increase in cortical thickness and that a 1000-fold increase in cortical surface area between humans and mice is only accompanied by a 2-fold increase in cortical thickness (Rakic, 1988) also supports the idea of stability of cortical thickness over cortical volume. The high variability in cortical volumes may be one of the explanations of inconsistent findings in the literature while comparing MCI and Alzheimer's disease groups (Chetelat and Baron, 2003). As such, cortical thickness may be a more reliable measure to detect differences between MCI and Alzheimer's disease groups and may also play an important role in identifying the MCI subjects that would progressively develop Alzheimer's disease compared to those who remain stable (Ritchie et al., 2001).
As a first step we have presented novel cross-sectional findings from our data. However, the MCI population in our clinic is being followed annually and in future we will be reporting about the longitudinal patterns of cortical thickness with the progression of the disease.
We wish to thank Shelly Solomon and Randi Pilon for invaluable technical assistance and Blake Richards for valuable editorial and technical comments. This study was supported by operating grants from the Canadian Institutes for Health Research, the Alzheimer Society of Canada, and the Fonds de la recherche en santé du Québec. Funding to pay the Open Access publication charges for this article was provided by Canadian Institutes of Health Research (CIHR).