-
PDF
- Split View
-
Views
-
Cite
Cite
Y. Yamaguchi, T. Kubo, T. Murakami, M. Takahashi, Y. Hakamata, E. Kobayashi, S. Yoshida, K. Hosokawa, K. Yoshikawa, S. Itami, Bone marrow cells differentiate into wound myofibroblasts and accelerate the healing of wounds with exposed bones when combined with an occlusive dressing, British Journal of Dermatology, Volume 152, Issue 4, 1 April 2005, Pages 616–622, https://doi.org/10.1111/j.1365-2133.2005.06402.x
- Share Icon Share
Summary
Background The usefulness of bone marrow cells in accelerating wound healing has not been evaluated despite increasing evidence that bone marrow contains mesenchymal stem cells that have multipotentiality to differentiate into various types of cells after they enter the microenvironment of a specific tissue (niche).
Objectives To determine the effects of bone marrow cells and occlusive dressings in promoting wound healing in rats.
Methods We investigated by grafting, biopsy and immunohistochemistry whether various types of cells derived from green fluorescent protein (GFP)‐transgenic rats would differentiate into wound component cells when administered topically on the wounds of rats. We also investigated whether topical application of bone marrow cells with an occlusive dressing would accelerate the healing of wounds with exposed bones, as measured by planimetry.
Results GFP‐labelled bone marrow cells contained multipotent stem cells that sufficiently differentiated into wound myofibroblasts presenting with α‐smooth muscle actin in granulation tissue. Other types of cells, including myocytes, adipocytes, peripheral blood cells from buffy coat and dermal fibroblasts, did not express myofibroblast characteristics morphologically or immunohistochemically. Application of bone marrow cells and an occlusive dressing accelerated the repair of wounds with exposed bones, compared with an occlusive dressing only or with the topical administration of bone marrow cells plus a semidry to dry dressing.
Conclusions Our study indicates that bone marrow cells accelerate the healing of wounds at least in part through their differentiation into wound myofibroblasts. Thus, treatment of wounds with bone marrow cells and a supportive occlusive dressing is effective in promoting the formation of healthy granulation tissue and also for the preparation of an ideal wound bed.
There is increasing evidence that bone marrow cells contain multipotent stem cells that can differentiate not only into haematopoietic cells (including macrophages1) but also into nonhaematopoietic cells (including endothelial cells,2 pericytes,3 chondrocytes, osteoblasts, adipocytes,4 neural component cells,5 fibroblasts6, 7 and probably keratinocytes8), all of which play important roles in the process of tissue repair.9–11 Surprisingly, bone marrow cells have also been shown to differentiate into glomerular mesangial cells,12 hepatocytes13 and myogenic cells (including myocardial cells14 and myoblasts15) after they have entered the microenvironment of a specific tissue (niche). Consequently, many investigators treating various intractable diseases (including ischaemic myocardial diseases,16 osteogenesis imperfecta,17 Duchenne's muscular dystrophy18 and central nervous system disorders such as Parkinson's disease19) are focusing on bone marrow stem cells, which do not involve ethical considerations as do embryonic stem cells.
However, to date the effects of bone marrow cells on wound healing have not been clarified.9, 20, 21 Badiavas et al. reported that bone marrow cells could become perifollicular cells, blood vessel cells and perisebaceous gland cells during the healing process 21 days after wounding.9 Additionally, a few clinical investigations have reported the possible application of bone marrow cells to treat various chronic skin ulcers caused by ischaemic diseases, venous insufficiency,20 vasculitis accompanied with collagen diseases or diabetes mellitus.21
In this study, we determined which type(s) of cells bone marrow cells can differentiate into and whether other cell types can also differentiate into a wound environmental niche, using green fluorescent protein (GFP)‐transgenic rats.22 More specifically, we focused on the hypothesis that bone marrow cells topically administered to wounds can differentiate into myofibroblasts in sufficient numbers to promote the wound‐healing process. We examined whether other types of tissue transplants, including dermis, buffy coats, fatty layers and muscles, can also participate in the formation of wound myofibroblasts. We also investigated whether bone marrow cells and an occlusive dressing promoted the healing of wounds with exposed bones in rats. The results demonstrate that bone marrow cells differentiate into wound myofibroblasts presenting with α‐smooth muscle actin and that those cells play important roles in the formation of healthy granulation tissue when combined with an occlusive dressing.
Materials and methods
Tissue transplantation studies
All rats were housed in the Centre for Molecular Medicine in the Jichi Medical School or in the Osaka University Animal Facility, according to institutional guidelines.23–25 Hemizygous GFP‐transgenic rats derived from Wistar rats22 at the age of 8–12 weeks were used as donors to check the morphological changes of various types of cells transplanted on to wounds of normal Wistar rats (Clea Japan, Tokyo, Japan) made when the animals were 10–12 weeks old. Two round areas (measuring 2 cm in diameter) were surgically excised in each normal rat down to the dorsal muscle fascia under Nembutal anaesthesia. Five types of tissues obtained from GFP‐transgenic rats were transplanted on to one of the two wound sites of five normal Wistar rats as follows: dermis obtained from full‐thickness skin with 500 U mL−1 dispase (Godo Shusei, Tokyo, Japan) treatment,26 adipose tissue, skeletal muscle, buffy coat obtained from 2 mL of heparinized whole blood by Ficoll–Paque (Pharmacia Biotech, Uppsala, Sweden) density gradient centrifugation27 and bone marrow cells obtained from a femur (Fig. 1). The corresponding tissues obtained from normal Wistar rats were transplanted on to the other wound site of each rat as negative controls. The expression of GFP in various organs was examined using a CCD camera under excitation at 489 nm (Leica MZFL III fluorescent microscope). Each graft was covered with an occlusive dressing (Tegaderm Plus; 3M Health Care, St Paul, MN, U.S.A.). Experiments were repeated three times to check the reproducibility. To prevent graft rejection, 0·64 mg kg−1 tacrolimus (Fujisawa Pharmaceutical Co., Osaka, Japan) was injected intramuscularly every day until the biopsy at 2 weeks postgrafting.28
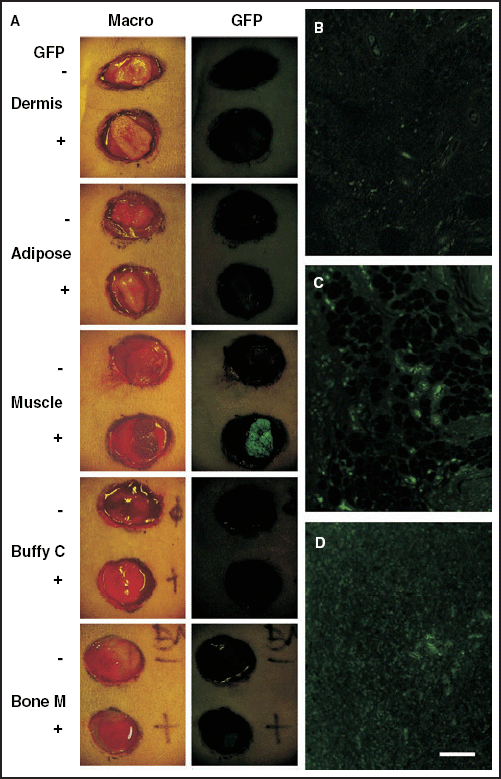
Tissue transplantation studies in rats. Two round areas, each measuring 2 cm in diameter, were surgically excised down to the dorsal muscle fascia of each normal rat as donor sites. Five types of tissues obtained from normal rats (upper site in each panel) or from green fluorescent protein (GFP)‐transgenic rats (lower site in each panel) were transplanted onto the wounds: dermis, adipose tissue, skeletal muscle, buffy coat from heparinized whole blood, or bone marrow cells. Compared with normal rats, intense GFP expression was observed only in muscle cells derived from GFP‐transgenic rats macroscopically and faint expression was detected in bone marrow cells under excitation at 489 nm (A). Microscopic examination showed enhanced GFP expression in the dermis (B), in adipose tissues (C) and in peripheral blood cells from buffy coats (D), and obvious morphological differences among the various GFP‐positive tissues. These are representative figures of three independent studies. Macro, Macroscopic view; GFP, GFP expression macroscopically examined under excitation at 489 nm; Buffy C, buffy coat; Bone M, bone marrow cells. Scale bar = 40 µm (B–D).
Immunohistochemical studies
Biopsy samples were fixed in 4% paraformaldehyde, embedded in paraffin, sectioned, and then examined immunohistochemically (Figs 2 and 3). The enhanced GFP expression was still observed after this procedure. The 5‐µm sections were blocked with 10% normal donkey serum (Jackson Immuno Research, West Grove, PA, U.S.A.) at room temperature for 30 min. The primary antibody used was monoclonal mouse anti‐α‐smooth muscle actin (Dako, Glostrup, Denmark). After washing with phosphate‐buffered saline containing 0·05% Tween 20, samples were incubated with CyTM3‐conjugated donkey antimouse IgG antibody (Jackson Immuno Research). Samples incubated with preimmune sera were used as negative controls. The enhanced GFP expression was still intense after these procedures. Immunoreactivity was visualized using a Nikon fluorescence microscope or a BioRad laser scanning confocal microscope. The samples were also processed for routine haematoxylin and eosin staining, but the enhanced GFP expression disappeared during that staining procedure.
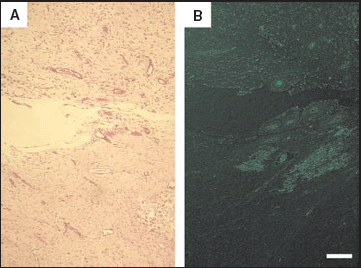
Formation of granulation tissue in green fluorescent protein (GFP)‐transgenic rats. A biopsy was taken from the tissue‐transplanted rats (Fig. 1) at 2 weeks. Granulation tissue had formed in all wounds transplanted with dermis, adipose tissue, skeletal muscle, peripheral blood cells or bone marrow cells (A, haematoxylin and eosin staining). GFP‐positive granulation tissue, examined under excitation at 489 nm, formed only in wounds treated with bone marrow cells derived from GFP‐transgenic rats (B) and to a much lesser degree in those treated with buffy coat. GFP‐positive granulation tissue did not form in wounds treated with dermis derived from GFP‐transgenic rats. Scale bar = 80 µm (A,B).
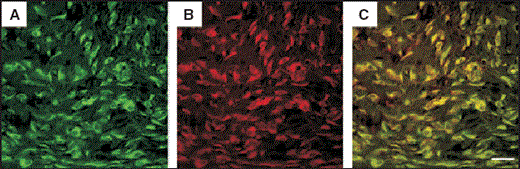
Immunohistochemical staining for α‐smooth muscle actin in granulation tissue formed by green fluorescent protein (GFP)‐labelled bone marrow cells. Specimens were analysed with anti‐α‐smooth muscle actin antibody conjugated with CyTM3 (indocarbocyanine) as a specific marker for wound myofibroblasts. Immunofluorescence images showed that the green fluorescence was derived from spindle‐shaped GFP‐positive bone marrow cells (A) and the red fluorescence from CyTM3‐conjugated α‐smooth muscle actin (B) under excitation at 489 nm and 550 nm, respectively. Double‐labelling fluorescence revealed that most spindle‐shaped GFP‐positive cells also expressed the wound myofibroblast marker (C). This differentiation did not occur in muscle, adipose or dermal tissue (of GFP‐transgenic rat origin) transplanted onto normal rat wounds. Some GFP‐positive peripheral blood cell transplants very slightly differentiated into myofibroblasts, but the number of cells was much lower than with bone marrow cells. Scale bar = 20 µm (A–C).
Wound contraction studies
Twenty‐four Wistar rats (aged 8–12 weeks) were used to investigate the effects of bone marrow cells on wound contraction. An oval area (2 × 3 cm) was surgically excised down to the muscle fascia, then an oval area (1 × 2 cm) within that site was further excised down to the calvarium bone, under Nembutal anaesthesia. Three types of treatments were performed to treat the wounds with exposed bones: topical administration of bone marrow cells obtained from an autologous femur bone plus an occlusive dressing, an occlusive dressing without bone marrow transplantation, and topical administration of autologous bone marrow cells plus a semidry to dry dressing (Fig. 4). The results were photographed every week, and were followed by planimetry using NIH image software.
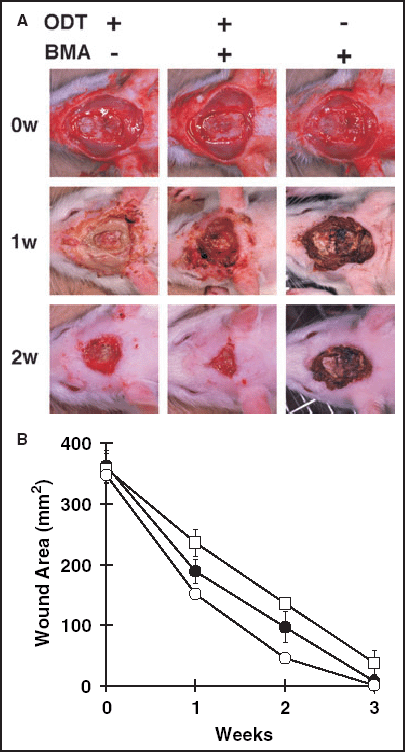
Wound contraction studies in rats. An oval area (2 × 3 cm) was surgically excised down to the muscle fascia, then an oval area (1 × 2 cm) was further excised down to the calvarium bone. Three types of treatments were performed to treat eight wounds with exposed bones (n = 24 animals in total) (A). The results were photographed every week, followed by planimetry using NIH image software. Two weeks after the operation, deep wounds treated with bone marrow cells plus an occlusive dressing had healed significantly more rapidly (B) than those treated either with the occlusive dressing only (P < 0·0001) or with the topical administration of bone marrow cells plus a semidry to dry dressing (P < 0·0001). ODT, Occlusive dressing therapy; BMA, bone marrow administration; ○, ODT(+), BMA(+); •, ODT(+), BMA(–); □, ODT(–), BMA(+).
Statistical analyses
The SPSS 10.0 system (SPSS Inc., Chicago, IL, U.S.A.) was used for statistical analyses. Bonferroni's multiple comparisons were used for statistical analyses of wound contraction experiments.
Results
Bone marrow cells differentiate into myofibroblasts
First, we examined whether various tissues obtained from GFP‐transgenic rats expressed enhanced GFP under excitation at 489 nm (Fig. 1). Compared with negative control tissues obtained from normal Wistar rats, intense GFP expression was observed macroscopically only in muscle tissue derived from GFP‐transgenic rats, and faint expression was detected in bone marrow cells (Fig. 1A). However, microscopic examination under excitation showed enhanced GFP expression in dermis, adipose tissue and peripheral blood cells from buffy coats (Fig. 1B–D). There were obvious morphological differences among the various GFP‐positive tissues immediately after the transplantation, except for peripheral blood cells from buffy coat and bone marrow cells (Fig. 1D).
We performed tissue transplantation studies on to wounds generated deep into the dorsal muscle fascia of normal rats. Among wounds treated with various transplanted tissues and covered with an occlusive dressing, those treated with bone marrow cells displayed higher rates of healing than those treated with peripheral blood cells (data not shown). Biopsies were taken at 2 weeks postgrafting, and we examined the morphological changes of various types of cells obtained from GFP‐transgenic rats transplanted on to the wounds. Granulation tissue, which consists of newly formed vessels and dense fibroblastic cells,10, 11 was observed in all wounds transplanted with dermis, adipose tissue, skeletal muscle, peripheral blood cells or bone marrow cells (Fig. 2A). However, GFP‐positive granulation tissue formed only in wounds treated with bone marrow cells derived from GFP‐transgenic rats (Fig. 2B), and to a much lesser degree in those treated with peripheral blood cells (data not shown). In other words, morphological changes into granulation tissue occurred exclusively in wounds treated with bone marrow cells and only very slightly in wounds treated with the buffy coat. Surprisingly, GFP‐positive granulation tissue did not form in wounds treated with dermis derived from GFP‐transgenic rats (data not shown). We could not detect GFP‐positive blood vessels, even in wounds treated with bone marrow cells, by microscopic examination.
The specimens were analysed with anti‐α‐smooth muscle actin antibody conjugated with CyTM3 (indocarbocyanine) as a specific marker for wound myofibroblasts.11 Immunofluorescence images produced by confocal microscopy showed green fluorescence derived from spindle‐shaped GFP‐positive bone marrow cells (Fig. 3A) and red fluorescence derived from CyTM3‐conjugated α‐smooth muscle actin (Fig. 3B) under excitation at 489 nm and 550 nm, respectively. Double‐labelling fluorescence revealed that most spindle‐shaped GFP‐positive cells also expressed the wound myofibroblast marker (yellow in Fig. 3C). The dramatic differentiation of bone marrow cells into wound myofibroblasts did not occur in muscular, fatty or dermal tissues (from GFP‐transgenic rat origin) transplanted on to rat wounds (data not shown). Some GFP‐positive peripheral blood cell transplants differentiated slightly into myofibroblasts, but that occurred to a much lesser extent than seen for bone marrow cells (data not shown).
Wounds heal fastest when treated topically with bone marrow cells plus an occlusive dressing
Finally, wound contraction studies were performed to determine whether exposure to bone marrow and a supportive occlusive dressing would be useful for treating such wounds. The healing rate of wounds reaching down to the calvarium bone was measured for three types of therapy (Fig. 4A), each group consisting of eight animals. Two weeks after the injury, deep wounds treated with bone marrow cells plus an occlusive dressing had healed significantly more rapidly (Fig. 4B) than those treated with the occlusive dressing only (P < 0·0001) or with the topical administration of bone marrow cells only (P < 0·0001).
Discussion
Surprisingly, transplanted dermis does not differentiate into myofibroblasts after it is transplanted on to a wound and observed at 2 weeks postgrafting. Skin graft healing can be divided into three phases: the plasmatic imbibition phase, the inosculatory phase, and the revascularization phase.24 Fibroblasts derived from the transplanted dermis may not contribute to the graft acceptance or as a source of wound myofibroblasts, as vascular anastomosis and reperfusion play exclusive and important roles in graft healing. Additionally, it may be difficult for transplanted dermal fibroblasts to migrate into a wound to act as a source of myofibroblasts and to contract the wound, as a skin graft is conventionally used to prevent the undesired wound contracture.
Furthermore, myofibroblasts derived from transplanted buffy coat cells were barely seen in the wounds, which suggests that circulating fibrocytes in the blood are not major sources of wound myofibroblasts.29 However, 2 mL of peripheral haematopoietic cells might not be sufficient to observe cells positive for GFP and for α‐smooth muscle actin. Furthermore, we could not use vimentin expression as a marker to localize fibroblasts as the rat tissue did not cross‐react well with antibodies under conditions in which the expression of GFP remained.
In contrast, bone marrow cells showed sufficient differentiation into myofibroblasts presenting with α‐smooth muscle actin after they entered the microenvironment of a wound. Although recent studies have reported the differentiation of bone marrow cells into various types of cells,1–9, 12–19 few of them have shown that these cells sufficiently differentiate into a functional tissue or organ. More recently, the fact that bone marrow cells possess a broad differentiation potential has been attributed to the fusion of bone marrow‐derived cells with a specific cell type,30, 31 including hepatocytes,32, 33 cardiomyocytes34 and neurones.35 The sufficiency of differentiation of bone marrow cells into myofibroblasts (rather than the mechanisms of differentiation by transdifferentiation or by cell fusion) was important for us to investigate as the hypothesis we tested was whether bone marrow cells would promote wound healing.
The plasticity of bone marrow cells prompted us to study the effects of transplantation of these cells on wound healing. Usually, a specified niche is required to induce the differentiation of multipotent bone marrow stem cells into a specific type of cell,1–9, 12–19 thus making the clinical application difficult. One of our major objectives in this study was to prove the usefulness of bone marrow cells to treat chronic wounds in a wound environmental niche. We recently reported that early aggressive debridement of diabetic foot ulcers with exposed bones down to a bleeding vascularized base (to expose bone marrow cells) and subsequent epidermal grafting (to induce the characteristics of the palmoplantar phenotype rapidly11, 26, 36, 37) significantly improved healing and reduced the rate of amputation.21 Granulation tissue consists of new vessels formed by angiogenesis and vasculogenesis along with the accumulation of fibroblasts, myofibroblasts and dermal matrix.10, 11 Myofibroblasts have the features of fibroblasts and of smooth muscle cells and contain α‐smooth muscle actin, which plays a major role in wound contraction.10, 11 Our novel finding of the differentiation of bone marrow cells into wound myofibroblasts in vivo partly explains why wounds treated topically with bone marrow cells plus an occlusive dressing heal well and why exposure of the bone marrow significantly reduces amputation in patients with diabetic foot ulcers.21 Badiavas and Falanga reported similar observations on the effectiveness of bone marrow cells in three patients with venous ulcers20 and they also showed the participation of bone marrow cells in the wound‐healing process using transfusion of GFP‐positive bone marrow cells into lethally irradiated mice.9 Bone marrow cells may have also differentiated into endothelial cells2 and pericytes3 at the wound site, as both types of cells play key roles in the formation of new vessels, although further investigation is needed to confirm this.
Occlusive dressings for wound management are replacing dry dressings as various products for moist treatment are now available which have been proven to be clinically effective.11, 38 Our present study also shows enhanced wound contraction when treated with an occlusive dressing. However, most clinicians rarely use this type of dressing to treat wounds accompanied with osteomyelitis, because occlusive dressings are thought to increase the risk of microbial infection. This probably explains the few reports in the literature combining bone marrow shaving and an occlusive dressing,21 despite accumulating evidence for the plasticity of bone marrow cells.1–9, 12–19 We decided to combine these two types of therapies for the following reasons: (i) bone marrow cells have been reported to differentiate into macrophages that could phagocytose microorganisms;1 (ii) microorganisms could be eliminated spontaneously when healthy granulation tissue forms rapidly; and (iii) the condition of the wound bed could be readily checked through a clear film dressing. This combined therapy to treat rat wounds resulted in a healthy wound bed preparation and in wound contraction within several weeks.
In summary, we have shown that bone marrow cells accelerate the healing of wounds, at least in part through differentiation into wound myofibroblasts presenting with α‐smooth muscle actin. Other tissues, including dermis, adipose tissue, muscle and peripheral blood cells from the buffy coat, were not able to differentiate into myofibroblasts in our experimental system. Topical administration of bone marrow cells plus an occlusive dressing enhances wound contraction, compared with an occlusive dressing only or topical administration of bone marrow cells plus a semidry or dry dressing. Thus, exposure of the bone marrow in wounds and a supportive occlusive dressing can be a major therapeutic approach to treat chronic wounds.
Acknowledgments
We thank Dr Vincent J. Hearing, Laboratory of Cell Biology, NCI, NIH, and the National Cancer Institute, Center for Cancer Research Fellows Editorial Board for their critical review of this work. Supported by a Grant‐in‐Aid for Scientific Research from the Ministry of Education, Science, Sports and Culture of Japan and from the Tissue Engineering Research Project managed by the New Energy & Industrial Technology Development Organization.
References
Author notes
Conflicts of interest: None declared