-
PDF
- Split View
-
Views
-
Cite
Cite
Y. H. Liu, B. P. Dhakal, C. Keesakul, R. M. Kacmarek, G. D. Lewis, Y. Jiang, Continuous non-invasive cardiac output monitoring during exercise: validation of electrical cardiometry with Fick and thermodilution methods, BJA: British Journal of Anaesthesia, Volume 117, Issue 1, July 2016, Pages 129–131, https://doi.org/10.1093/bja/aew156
- Share Icon Share
Editor—Cardiopulmonary exercise testing (CPET) is an important diagnostic procedure, and gas exchange variables along with invasive haemodynamic parameters measured during CPET provide diagnostic and prognostic information regarding patients with a variety of cardiopulmonary conditions, such as heart failure and pulmonary hypertension.1,2 Measurement of cardiac output (CO) and the corresponding stroke volume in response to different levels of exercise is a critical component of CPET. The CO during CPET has traditionally been measured by the Fick method. Owing to its complexity, thermodilution (TD) is often used as a surrogate. However, TD is as invasive as Fick and requires pulmonary artery catheter placement. Therefore, interest has been great regarding the development of non-invasive techniques for CO measurements.3,4
Electrical Cardiometry™ (EC) is an advanced form of transthoracic bio-impedance for non-invasive CO measurement. It takes advantage of changes in electrical impedance associated with the change in orientation of red blood cells in the aorta.5–7 This technique has been validated against TD in critically ill,8,9 preoperative,10 and paediatric patients.11 However, comparison of EC with the Fick method across a large range of COs obtained during CPET has not been conducted. The aims of our study were as follows: (i) to compare COs obtained with the Fick method (COFick) and EC (COEC; AESCULON®; Cardiotronic, La Jolla, CA, USA) at rest and at 1 min intervals during incremental CPET; and (ii) to compare COs obtained with thermodilution (COTD), Fick, and EC before exercise because COTD was not obtainable during excise.
We enrolled 47 patients (≥18 yr old) with dyspnoea on exertion who underwent maximal incremental upright cycle ergometry CPET with invasive haemodynamic monitoring (MedGraphics, St Paul, MN, USA). The patients exercised for 7.3 (1.8 sd) min, achieving 114.6 (53.7 sd) W. During exercise, we found a significant correlation between COFick and COEC (r2=0.89, P<0.0001; Fig. 1a). Bland–Altman analysis indicated a bias (mean difference) of 0.62 litres min−1 and 95% limit of agreement (1.96×sd) of 2.77 litres min−1 (Fig. 1b). The error per cent (95% limit of agreement/mean CO) was 29.2%. Analysis of COs at rest showed bias and 95% limit of agreement 0.28 and 2.00 litres min−1 between COEC and COFick (Fig. 1c), and 0.30 and 2.20 litres min−1 between COTD and COFick (Fig. 1d), respectively. The error per cent was 36.8 and 38.8% for COEC and COTD, respectively.
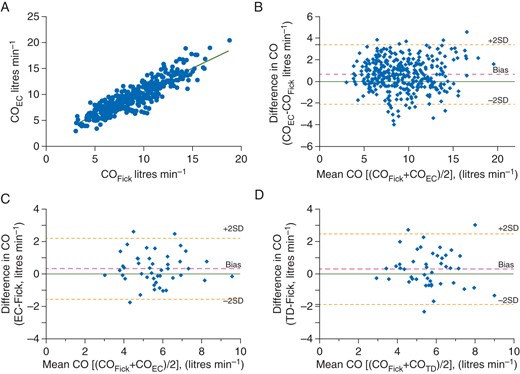
Comparison of cardiac output measured with electrical cardiometry (COEC), the Fick method (COFick), and the thermodilution method (COTD). (a) Correlation of COFick and COEC during exercise. (b) Bland–Altman plot of the difference in COFick and COEC during exercise. (c) Bland–Altman plot of the difference in COFick and COEC at rest. (d) Bland–Altman plot of the difference in COFick and COTD at rest.
In our study, a signal quality index of <50% was chosen as the data exclusion criterion. Using this value as cut-off, 25% (128 of 521) of data points collected during exercise were considered unreliable and excluded. Poor EC signal quality was clustered in 13 (28%) subjects, causing 82% (105 of 128) of poor-quality data points. In 10 of these patients, BMI was ≥30 kg m−2. An exploratory analysis indicated that morbid obesity was one of the contributing factors to low signal quality.
Our results show that EC was able to track the direction of changes in CO during CPET. The precision of COECvs COFick during exercise was within the criteria of acceptability, i.e. error per cent ≤30%.12 Considering the advantages of EC, including safety, user friendliness, and low cost, it may be clinically advantageous to use EC during CPET.
The COEC at rest achieved limits of agreement very close to that of COTD, although the absolute precision of both techniques was out of the 30% limit. The potential cause of poor precision at rest is most likely to be the patients' co-morbidities; heart failure (n=3), pulmonary hypertension (n=3), and valvular insufficiency (n=10). Previous studies have suggested that the accuracy and precision of TD and Fick methods may be compromised in such conditions.13–16 Further studies are needed to validate the clinical significance of EC in these subgroups of patients.
Declaration of interest
None declared.
Funding
Departmental funding. The equipment used in this study for measurement of cardiac output, AESCULON®, was provided by the manufacturer, Cardiotronic, Oyspka Medical Inc., La Jolla, CA, USA. R.M.K. is a consultant for Covidien and OrangeMed and has received research grants form Covidien and Venner Medical.