-
PDF
- Split View
-
Views
-
Cite
Cite
A. Assadi, O. Desebbe, C. Kaminski, T. Rimmelé, F. Bénatir, J. Goudable, D. Chassard, B. Allaouchiche, Effects of sodium nitroprusside on splanchnic microcirculation in a resuscitated porcine model of septic shock, BJA: British Journal of Anaesthesia, Volume 100, Issue 1, January 2008, Pages 55–65, https://doi.org/10.1093/bja/aem278
- Share Icon Share
Abstract
We tested the hypothesis that sodium nitroprusside (SNP) might improve the impairment of hepatosplanchnic microcirculatory blood flow (MBF) in septic shock.
Fourteen pigs were anaesthetized and their lungs mechanically ventilated. Sepsis was induced with i.v. infusion of live Pseudomonas aeruginosa [1×108 colony forming units (CFU) ml−1 kg−1] for 1 h. Sixty minutes later, the animals received in a random succession either SNP or normal saline for 30 min. Mean arterial pressure (MAP), cardiac index (CI), mean pulmonary artery pressure (MPAP), carbon dioxide tension of the ileal mucosa (Pco2; by gas tonometry), ileal mucosal and hepatic MBF by laser Doppler flowmetry, blood gases, and lactates were assessed before, during administration, and 30 min after discontinuing the test drug.
Bacterial infusion promoted hypodynamic shock (MAP −18%, CI −33%, ileal MBF −19%, and hepatic MBF −27%), which was converted to normodynamic shock by resuscitation. During SNP infusion, ileal mucosal MBF significantly increased (+19%) compared with control (P = 0.033). Although hepatic MBF increased (+42% from baseline), this did not differ from control. In order to maintain a constant central venous pressure and MAP, fluid loading and norepinephrine (P < 0.01) were increased. Acid–base status was not altered by SNP.
In a resuscitated porcine model of the early phase of septic shock, SNP improved ileal mucosal MBF but required a concomitant increase in fluid and norepinephrine supplements to maintain constant systemic haemodynamic parameters.
During septic shock, hepatosplanchnic effects may provoke gut mucosal barrier dysfunction, resulting in bacterial translocation and multiple organ failure.1 Despite optimization of systemic circulation, increase in splanchnic blood flow may be insufficient to satisfy oxygen uptake.2 Indeed, some treatments aimed at improving systemic or regional circulation may not improve microcirculation.3 Therefore, a treatment improving splanchnic mucosal microcirculatory blood flow (MBF) could limit bacterial translocation and be of use in the treatment of septic shock.
Under basal conditions, the release of nitric oxide (NO) via constitutive NO synthase (cNOS) at a constant and low rate plays an essential role in the maintenance of capillary flow and oxygen availability to the tissues, which may increase acutely for a short time.4 During sepsis, cNOS expression decreases, and its activity may be depressed during the early phase of endotoxaemia, resulting in impaired endothelium-dependent vasodilatation, whereas inducible NOS (iNOS) release, contributing to hypotension, may take several hours to occur.4 These enzymatic modifications could then be considered as an adapted response to limit tissue injury in the acute setting by redistribution of blood flow4 and recruiting shunted microcirculatory units.5 It is therefore reasonable to hypothesize that early administration of an NO donor may be beneficial to preserve tissue perfusion. Moreover, the efficacy and safety of vasodilators have not often been tested on macro- and microcirculation in association with usual haemodynamic treatment of septic shock, particularly with fluid volume and norepinephrine. Therefore, the primary objective of this study was to test the effect of NO donor SNP on hepatosplanchnic MBF in a porcine resuscitated model of septic shock.
Methods
Animal preparation
The local animal ethics committee of the university of Claude-Bernard, Lyon, France, approved the protocol. Fourteen female pigs (weight, 30 kg) were fasted overnight but were allowed free access to water. Pigs were sedated with i.m. injection of ketamine (10 mg kg−1). Anaesthesia was induced with propofol (3 mg kg−1) and was maintained with sevoflurane (MAC=2), sufentanil (1 µg kg−1 h−1), and cisatracurium at doses of 0.3 mg kg−1 every 30 min. After performing a tracheostomy, animals' lungs were mechanically ventilated with an Fio2 that maintained an arterial partial pressure of O2 (Pao2) above 80 torr (10.7 kPa), at a tidal volume of 10–15 ml kg−1, and ventilatory frequency that maintained Paco2 at 40 (5) Torr [5.3 (0.7) kPa]. End-expiratory pressure was zero. Catheters were inserted into the right carotid artery, for continuous monitoring of mean arterial pressure (MAP) and for collecting blood samples, and into the right atrium through the left external jugular vein for fluid and drug administration. A 7-Fr thermister-tip catheter (Baxter Edwards Critical-Care, Irvine, CA, USA) was advanced into the pulmonary artery through the right external jugular vein to measure mean pulmonary artery pressure (MPAP) (mm Hg), central venous pressure (CVP) (mm Hg), pulmonary capillary wedge pressure (PCWP, mm Hg), and cardiac index (CI) (ml min−1 kg−1) and to draw mixed venous blood samples. The location of the catheter tip was determined by observing the characteristic pressure trace on the monitor as it was advanced through the right heart into the pulmonary artery. The central venous temperature was monitored with a thermistor in the pulmonary artery. Temperature of the animal was maintained at 37.2 (0.8)°C with a heating pad and heat lamps. All animals were given a continuous infusion of 15–20 ml kg−1 h−1 lactated Ringer's solution. This infusion was adjusted to maintain CVP and PCWP values at 6–8 mm Hg, which were our endpoints for fluid therapy.
A midline laparotomy was performed. A catheter was inserted into the portal vein for blood sampling.6 A 10 cm segment of distal ileum, 20 cm proximal to the ileocaecal junction, was defined. Initially, a tonometric silicone catheter (TRIP, NGS tonometry catheter, Tonometrics. Helsinki, Finland) was introduced into the ileum proximal to the isolated ileal segment. A platform was placed above the abdomen and fixed to a ring stand on either side of the pig. The isolated ileal segment was opened along its entire anti-mesenteric border and exteriorized on the ventral midline of the animal.7 The cut edges of ileum were stitched to the platform. The height of the platform above the ventral abdominal wall was adjusted in such a way that the mucosa of the opened bowel was well exposed, but the mesentery supplying the segment was not compressed. A laser Doppler flowmetry (LDF) probe was suspended and positioned perpendicularly on the intestinal mucosa with the aid of a special miniholder (Perimed, Probe 407 with PH 07 Miniholder); in this way, the LDF probe would generate minimal pressure on the tissue surface and artifacts caused by respiratory movements and intestinal peristalsis were eliminated (Fig. 1). The exteriorized ileal mucosa was covered with humidified cotton and gauze, and the temperature of the gut was maintained with warm water and a heat lamp.
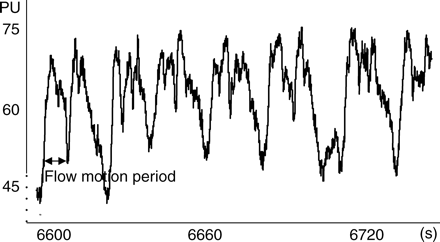
Extracts of 120 s of ileal MBF tracing by LDF in arbitrary PU. Note the absence of respiratory oscillations and peristaltic artifacts. Flow motion oscillations are present with frequency of 4–6 min−1.
The LDF probe, which was utilized to detect hepatic MBF, is flat and is positioned over the liver surface in the depth between the two lobes. It is secured in place by sutures and provided that the cord is loose enough to allow free movements without traction on the probe, there was no interference with liver movements.
In this way, the probe and the liver move in one block resulting in reliable recordings (Fig. 2). After satisfactory placement of LDF probes and tonometric catheter, animals were stabilized for 60 min.
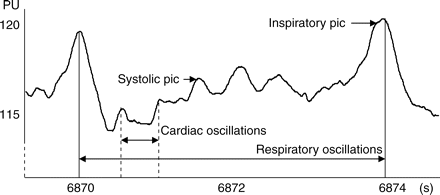
Extracts of hepatic MBF tracing by LDF in arbitrary PU. Respiratory oscillations are regular and constant throughout the experiment and slightly alter the signal amplitude.
Data acquisition
Haemodynamics
All intravascular pressure measurements were referenced to the mid-chest level. CI was measured by thermodilution. Three consecutive determinations of CI were made by injecting 10 ml of ice-cold bolus of dextrose 5% at end expiration. The mean value was recorded as CI. MAP, MPAP, PCWP, CVP, heart rate, and core temperature were continuously measured. Arterial, mixed venous, and portal vein blood samples were drawn for determinations of blood gases (ABL 520, Radiometer, Copenhagen, Denmark) and for the determination of lactate concentrations. Haemoglobin concentration was determined using a standard haematological method.
Hepatic and ileal mucosal blood flow
MBF was continuously measured with LDF,8 a well-characterized technique for measurement of tissue red blood cell flux. Because the optical probe has a spatial resolution of 0.5–1 mm3, the measurements obtained reflect only surface flow where the probe is applied and not in the entire intestinal wall.9 A detailed description and practical details have been published before.7,10–12 LDF has been validated in many organs, including gastrointestinal mucosa and the liver.10,12 In our case, LDF signals were exported via analogue output and acquired online via a multichannel interface (Mac Paq MP100; Biopac system Inc., Goleta, CA, USA) with acquisition/analysis software (Acqknowledge 7.2; Biopac system Inc.) to a portable computer. Two different signals were available for external recording. One output was proportional to the intensity of backscattered light. This signal is useful to determine whether the optical probe is making adequate contact with the tissue surface. The second signal is proportional to blood flow. LDF is not calibrated to measure absolute blood flow, but indicates MBF in arbitrary perfusion units (PU). Before each experiment, a calibration using random Brownian motion of small scatters in an emulsion (Periflux motility standard, Perimed) was performed. The quality of the LDF signal was controlled online by visualizing it on a computer screen, so that motion artifacts and noise due to inadequate probe attachment could be immediately detected and corrected before measurements started. Each reading was a mean of 2 min of LDF tracing. Because of large variability in baseline values, results are usually expressed as changes relative to baseline.
Ileal mucosal acid–base status
Measurement of ileal mucosal acid–base status by tonometry was described by Fiddian-Green and colleagues13 and was validated by Antonsson and colleagues.14 In our case, ileal mucosal Pco2 was measured using a standard tonometric tube (TRIP, NGS tonometry catheter, Tonometrics. Helsinki, Finland) connected to an automated gas analyzer (Tonocap TC-200, Datex, Helsinki, Finland). The silastic balloon of the tonometric catheter was inflated automatically in a closed circuit to measure the carbon dioxide tension (Pco2) of the ileal mucosa by infrared spectroscopy every 10 min. Ileal mucosal acid–base status was assessed by calculation of Pco2 gap as the difference between ileal mucosal Pco2 and arterial Pco2.
Bacterial preparation
A strain of Pseudomonas aeruginosa used in the study was isolated from stools of a patient. Bacteria were stored in special culture medium within cryotubes at −80°C at the Laboratory of Microbiology of Edouard Herriot Hospital, Lyon, France. The day before experimentation, bacteria were plated in culture and incubated at 37°C for 6 h. Broth (containing a mixture of heart and brain materials) was plated in gelose blood agar and incubated at 37°C for 18 h. Density of the suspension was controlled by numeration of two dilutions, which were plated in Muller Hinton gelose, by an interscience spiral system apparatus. Forty millilitres of bacterial suspension were prepared in sterile saline with a bacterial density of 1×108 CFU ml−1 (density of 1 on MacFarland scale) and were kept in a refrigerator ready for use.
Experimental design
When all preparations were complete (Fig. 3), the animals were allowed to recover for 60 min so that variables were stable. After baseline (T0) measurements were taken, sepsis was induced for 1 h (T0→T60) by i.v. infusion of live P. aeruginosa (1×108 CFU ml−1 kg−1 body weight). The porcine bacteraemic shock model used was adapted from models described by Bloomfield and colleagues15 and modified by Rimmele and colleagues.16 Thirty minutes after the start of bacterial infusion, CVP and PCWP were maintained at 6–8 mm Hg by adequate fluid replacement and MAP was maintained above 65 mm Hg, if necessary, by norepinephrine infusion (increments of 0.1 µg kg−1 min−1). One hour after the end of bacterial infusion (T120), each animal received, in a random succession, equal volumes of either SNP (5 µg kg−1 min−1) or normal saline (NS) (control) for 30 min (Fig. 4) through a central venous line (T120→T150), followed after 30 min of washout by the other drug (T180→T210). Measurements and blood sampling were performed before the administration of each test drug, 30 min after start of the test drug, and 30 min after discontinuing the test drug.
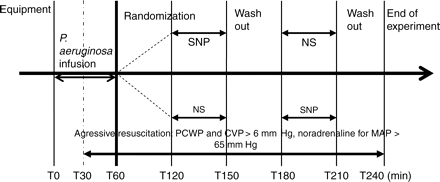
Experimental protocol. Each animal received in a random succession, equal volumes of either SNP (5 µg kg−1 min−1) or NS for 30 min.
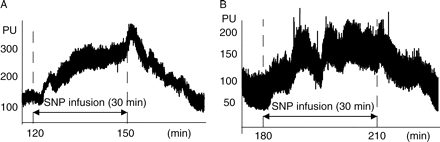
Extracts of typical (a) hepatic and (b) ileal MBF tracing by LDF in arbitrary PU. Relative MBF changes before, during continuous infusion of SNP, and after discontinuing drug administration. MBF increases during SNP administration and decreases after discontinuation.
After completing the experiment, all animals were killed with an i.v. injection of 20 mmol KCl under deep anaesthesia.
Statistical analysis
Parametric or non-parametric tests were used for continuous variables as appropriate after the normality of distribution was tested by a Shapiro–Wilk test. Measurements of LDF are expressed as changes relative to baseline. For all continuous variables between baseline and subsequent measurements, a Friedman repeated measures analysis of variance on ranks was used. To isolate groups that differ from one another, we used a three-way analysis of variance with order (control first or SNP first), group (control or SNP), and effect (before and during administration) as variation factors, and their interactions so as to evaluate a possible carry-over or a period effect. Post hoc, pairwise comparison among the groups was done using the Newman–Keuls test. For values before, during, and after administration of test drug, a Wilcoxon test was used. Results are expressed as median (inter-quartile range). Statistically significant differences were defined as P<0.05. We used Sigma Stat 3.1 software for Windows.
Results
Fourteen pigs were included in this study.
Systemic haemodynamic and MBF variables before drug administration
Infusion of live P. aeruginosa promoted a reduction in MAP (−18%, P=0.015) and CI (−33%). With i.v. fluid replacement and norepinephrine infusion, MAP was restored to its baseline level at 120 min and was maintained at this level until the end of the experiment. MPAP and PVR values remained above their baseline values. CI returned to its baseline level and then increased slightly (Fig. 5). Thus, resuscitation converted hypodynamic septic shock into a normodynamic shock with an increase in norepinephrine infusion at 120 min (P=0.001). At 30 min after bacterial infusion, hepatic MBF (−27%) and ileal mucosal MBF (−19%) diminished. However after resuscitation, hepatic MBF progressively increased, whereas ileal mucosal MBF remained below its basal values (Fig. 6). Systemic haemodynamic and MBF variables before drug administration are presented in Table 1 and variables before drug administration at T120 and T180 min are presented in Table 2.
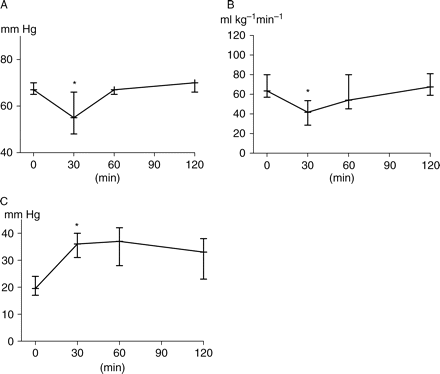
(a) MAP, (b) CI, and (c) MPAP changes before drug administration. Sepsis was induced at T0 min, at T30 min=data during hypodynamic shock, at T60 min=data after resuscitation to convert hypodynamic shock to normodynamic shock. At T120 min, pigs were stabilized 60 min before drug administration. Data are presented as median (inter-quartile range). *P<0.05 (different from baseline).
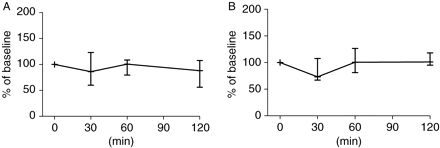
(a) Ileal and (b) hepatic MBF changes before drug administration. Sepsis was induced at T0 min; at T30 min resuscitation converted hypodynamic shock to normodynamic shock. At T120 min, pigs were stabilized for 60 min before drug administration. All MBFs were set at 100% at T0 min and values were expressed as percentage of baseline.
Haemodynamic and microcirculatory time course data, before drug administration. Sepsis was induced at T0 min; at T30 min=data during hypodynamic shock; at T60 min=data after resuscitation to convert hypodynamic shock to normodynamic shock. At T120 min, pigs were stabilized 60 min before drug administration. MBF, microcirculatory blood flow (% of baseline). All microcirculatory blood flows were set at 100% at T0 min. Data are presented as median (inter-quartile range). *P<0.05 (different from baseline)
Time (min) . | T0 . | T30 . | T60 . | T120 . |
---|---|---|---|---|
Mean arterial pressure (mm Hg) | 67 (65–70) | 55 (47–67)* | 67 (64–72) | 70 (66–71) |
Cardiac index (ml kg−1 min−1) | 63 (63–80) | 42 (29–53)* | 54 (45–81) | 67 (59–81) |
Heart rate (beats min−1) | 101 (93–112) | 100 (97–111) | 100 (97–110) | 115 (95–137) |
Pulmonary capillary wedge pressure (mm Hg) | 8 (6–10) | 6 (5–8) | 6 (6–8) | 8 (6–10) |
Central venous pressure (mm Hg) | 6 (5–80) | 6 (4–8) | 6 (4–7) | 7 (5–90) |
Mean pulmonary artery pressure (mm Hg) | 20 (17–24) | 36 (31–40)* | 37 (28–42) | 33 (23–38) |
Systemic vascular resistance (dyn s−1 cm−5) | 2815 (2557–3160) | 2954 (2652–4476) | 3496 (2492–4052) | 2949 (2378–3129) |
Pulmonary vascular resistance (dyn s−1 cm−5) | 529 (358–790) | 2222 (1590–2546)* | 1534 (801–2166) | 1023 (753–1354) |
Norepinephrine (µg kg−1 min−1) | 0.00 | 0.00 | 0.210 (0.050–0.230) | 0.195 (0.080–0.280) |
Time (min) . | T0 . | T30 . | T60 . | T120 . |
---|---|---|---|---|
Mean arterial pressure (mm Hg) | 67 (65–70) | 55 (47–67)* | 67 (64–72) | 70 (66–71) |
Cardiac index (ml kg−1 min−1) | 63 (63–80) | 42 (29–53)* | 54 (45–81) | 67 (59–81) |
Heart rate (beats min−1) | 101 (93–112) | 100 (97–111) | 100 (97–110) | 115 (95–137) |
Pulmonary capillary wedge pressure (mm Hg) | 8 (6–10) | 6 (5–8) | 6 (6–8) | 8 (6–10) |
Central venous pressure (mm Hg) | 6 (5–80) | 6 (4–8) | 6 (4–7) | 7 (5–90) |
Mean pulmonary artery pressure (mm Hg) | 20 (17–24) | 36 (31–40)* | 37 (28–42) | 33 (23–38) |
Systemic vascular resistance (dyn s−1 cm−5) | 2815 (2557–3160) | 2954 (2652–4476) | 3496 (2492–4052) | 2949 (2378–3129) |
Pulmonary vascular resistance (dyn s−1 cm−5) | 529 (358–790) | 2222 (1590–2546)* | 1534 (801–2166) | 1023 (753–1354) |
Norepinephrine (µg kg−1 min−1) | 0.00 | 0.00 | 0.210 (0.050–0.230) | 0.195 (0.080–0.280) |
Haemodynamic and microcirculatory time course data, before drug administration. Sepsis was induced at T0 min; at T30 min=data during hypodynamic shock; at T60 min=data after resuscitation to convert hypodynamic shock to normodynamic shock. At T120 min, pigs were stabilized 60 min before drug administration. MBF, microcirculatory blood flow (% of baseline). All microcirculatory blood flows were set at 100% at T0 min. Data are presented as median (inter-quartile range). *P<0.05 (different from baseline)
Time (min) . | T0 . | T30 . | T60 . | T120 . |
---|---|---|---|---|
Mean arterial pressure (mm Hg) | 67 (65–70) | 55 (47–67)* | 67 (64–72) | 70 (66–71) |
Cardiac index (ml kg−1 min−1) | 63 (63–80) | 42 (29–53)* | 54 (45–81) | 67 (59–81) |
Heart rate (beats min−1) | 101 (93–112) | 100 (97–111) | 100 (97–110) | 115 (95–137) |
Pulmonary capillary wedge pressure (mm Hg) | 8 (6–10) | 6 (5–8) | 6 (6–8) | 8 (6–10) |
Central venous pressure (mm Hg) | 6 (5–80) | 6 (4–8) | 6 (4–7) | 7 (5–90) |
Mean pulmonary artery pressure (mm Hg) | 20 (17–24) | 36 (31–40)* | 37 (28–42) | 33 (23–38) |
Systemic vascular resistance (dyn s−1 cm−5) | 2815 (2557–3160) | 2954 (2652–4476) | 3496 (2492–4052) | 2949 (2378–3129) |
Pulmonary vascular resistance (dyn s−1 cm−5) | 529 (358–790) | 2222 (1590–2546)* | 1534 (801–2166) | 1023 (753–1354) |
Norepinephrine (µg kg−1 min−1) | 0.00 | 0.00 | 0.210 (0.050–0.230) | 0.195 (0.080–0.280) |
Time (min) . | T0 . | T30 . | T60 . | T120 . |
---|---|---|---|---|
Mean arterial pressure (mm Hg) | 67 (65–70) | 55 (47–67)* | 67 (64–72) | 70 (66–71) |
Cardiac index (ml kg−1 min−1) | 63 (63–80) | 42 (29–53)* | 54 (45–81) | 67 (59–81) |
Heart rate (beats min−1) | 101 (93–112) | 100 (97–111) | 100 (97–110) | 115 (95–137) |
Pulmonary capillary wedge pressure (mm Hg) | 8 (6–10) | 6 (5–8) | 6 (6–8) | 8 (6–10) |
Central venous pressure (mm Hg) | 6 (5–80) | 6 (4–8) | 6 (4–7) | 7 (5–90) |
Mean pulmonary artery pressure (mm Hg) | 20 (17–24) | 36 (31–40)* | 37 (28–42) | 33 (23–38) |
Systemic vascular resistance (dyn s−1 cm−5) | 2815 (2557–3160) | 2954 (2652–4476) | 3496 (2492–4052) | 2949 (2378–3129) |
Pulmonary vascular resistance (dyn s−1 cm−5) | 529 (358–790) | 2222 (1590–2546)* | 1534 (801–2166) | 1023 (753–1354) |
Norepinephrine (µg kg−1 min−1) | 0.00 | 0.00 | 0.210 (0.050–0.230) | 0.195 (0.080–0.280) |
Variables before drug administration at T120 and T180 min. At T120 and T180 min, pigs are after stabilization periods before drug administration. MBF, microcirculatory blood flow (% of baseline). All microcirculatory blood flows were set at 100% at T0 min. Pco2 gap gradient between arterial and ileal mucosal Pco2. Data are presented as median (inter-quartile range)
Time (min) . | T120 . | T180 . |
---|---|---|
Hepatic MBF (% of baseline) | 101 (87 120) | 114 (95–155) |
Ileal MBF (% of baseline) | 84 (56–106) | 75 (60–117) |
Mean arterial pressure (mm Hg) | 70 (66–71) | 70.5 (66–71) |
Cardiac index (ml kg−1 min−1) | 67 (59–81) | 71 (64–108) |
Pulmonary capillary wedge pressure (mm Hg) | 8 (6–10) | 9 (5–9) |
Central venous pressure (mm Hg) | 8 (6–10) | 7 (5–8) |
Mean pulmonary artery pressure (mm Hg) | 33 (23–38) | 31 (24–32) |
Norepinephrine (µg kg−1 min−1) | 0.195 (0.080–0.280) | 0.185 (0.110–0.270) |
Fluid infusion (ml kg−1 h−1) | 650 (550–1100) | 1200 (1000–1500) |
Arterial lactate (mmol litre−1) | 2.89 (2.50–3.48) | 2.63 (2.03–2.86) |
Portal venous lactate (mmol litre−1) | 2.56 (2.14–3.05) | 2.27 (1.99–2.77) |
Mixed venous oxygen saturation (Svmo2) (%) | 79 (69–87) | 76 (68–86) |
Pco2 gap (Torr) | 22 (13–31) | 23 (18–28) |
Time (min) . | T120 . | T180 . |
---|---|---|
Hepatic MBF (% of baseline) | 101 (87 120) | 114 (95–155) |
Ileal MBF (% of baseline) | 84 (56–106) | 75 (60–117) |
Mean arterial pressure (mm Hg) | 70 (66–71) | 70.5 (66–71) |
Cardiac index (ml kg−1 min−1) | 67 (59–81) | 71 (64–108) |
Pulmonary capillary wedge pressure (mm Hg) | 8 (6–10) | 9 (5–9) |
Central venous pressure (mm Hg) | 8 (6–10) | 7 (5–8) |
Mean pulmonary artery pressure (mm Hg) | 33 (23–38) | 31 (24–32) |
Norepinephrine (µg kg−1 min−1) | 0.195 (0.080–0.280) | 0.185 (0.110–0.270) |
Fluid infusion (ml kg−1 h−1) | 650 (550–1100) | 1200 (1000–1500) |
Arterial lactate (mmol litre−1) | 2.89 (2.50–3.48) | 2.63 (2.03–2.86) |
Portal venous lactate (mmol litre−1) | 2.56 (2.14–3.05) | 2.27 (1.99–2.77) |
Mixed venous oxygen saturation (Svmo2) (%) | 79 (69–87) | 76 (68–86) |
Pco2 gap (Torr) | 22 (13–31) | 23 (18–28) |
Variables before drug administration at T120 and T180 min. At T120 and T180 min, pigs are after stabilization periods before drug administration. MBF, microcirculatory blood flow (% of baseline). All microcirculatory blood flows were set at 100% at T0 min. Pco2 gap gradient between arterial and ileal mucosal Pco2. Data are presented as median (inter-quartile range)
Time (min) . | T120 . | T180 . |
---|---|---|
Hepatic MBF (% of baseline) | 101 (87 120) | 114 (95–155) |
Ileal MBF (% of baseline) | 84 (56–106) | 75 (60–117) |
Mean arterial pressure (mm Hg) | 70 (66–71) | 70.5 (66–71) |
Cardiac index (ml kg−1 min−1) | 67 (59–81) | 71 (64–108) |
Pulmonary capillary wedge pressure (mm Hg) | 8 (6–10) | 9 (5–9) |
Central venous pressure (mm Hg) | 8 (6–10) | 7 (5–8) |
Mean pulmonary artery pressure (mm Hg) | 33 (23–38) | 31 (24–32) |
Norepinephrine (µg kg−1 min−1) | 0.195 (0.080–0.280) | 0.185 (0.110–0.270) |
Fluid infusion (ml kg−1 h−1) | 650 (550–1100) | 1200 (1000–1500) |
Arterial lactate (mmol litre−1) | 2.89 (2.50–3.48) | 2.63 (2.03–2.86) |
Portal venous lactate (mmol litre−1) | 2.56 (2.14–3.05) | 2.27 (1.99–2.77) |
Mixed venous oxygen saturation (Svmo2) (%) | 79 (69–87) | 76 (68–86) |
Pco2 gap (Torr) | 22 (13–31) | 23 (18–28) |
Time (min) . | T120 . | T180 . |
---|---|---|
Hepatic MBF (% of baseline) | 101 (87 120) | 114 (95–155) |
Ileal MBF (% of baseline) | 84 (56–106) | 75 (60–117) |
Mean arterial pressure (mm Hg) | 70 (66–71) | 70.5 (66–71) |
Cardiac index (ml kg−1 min−1) | 67 (59–81) | 71 (64–108) |
Pulmonary capillary wedge pressure (mm Hg) | 8 (6–10) | 9 (5–9) |
Central venous pressure (mm Hg) | 8 (6–10) | 7 (5–8) |
Mean pulmonary artery pressure (mm Hg) | 33 (23–38) | 31 (24–32) |
Norepinephrine (µg kg−1 min−1) | 0.195 (0.080–0.280) | 0.185 (0.110–0.270) |
Fluid infusion (ml kg−1 h−1) | 650 (550–1100) | 1200 (1000–1500) |
Arterial lactate (mmol litre−1) | 2.89 (2.50–3.48) | 2.63 (2.03–2.86) |
Portal venous lactate (mmol litre−1) | 2.56 (2.14–3.05) | 2.27 (1.99–2.77) |
Mixed venous oxygen saturation (Svmo2) (%) | 79 (69–87) | 76 (68–86) |
Pco2 gap (Torr) | 22 (13–31) | 23 (18–28) |
Haemodynamic and MBF variables during SNP administration
Ileal mucosal MBF (Fig. 7) increased during SNP infusion (+19%, P=0.013) and when compared with control (P=0.033). Hepatic MBF also increased during both SNP (+42%) and control (+7%) infusions, with no significant difference between the two groups (P>0.050). In order to maintain PCWP and CVP constant during SNP infusion, the quantity of fluid replacement (Fig. 8) was significantly increased during SNP when compared with control. Similarly, the rate of norepinephrine infusion (Fig. 8) was increased to maintain MAP constant during SNP infusion and when compared with control (P<0.001). It should be noted that all animals received norepinephrine. CI significantly increased during SNP infusion (P<0.01) but there was a poor correlation (Fig. 9) between CI and ileal MBF (r2=0.49, P=0.007) and no correlation with hepatic MBF (r2=0.26, P=0.07). Variables during SNP and control administration are presented in Table 3.
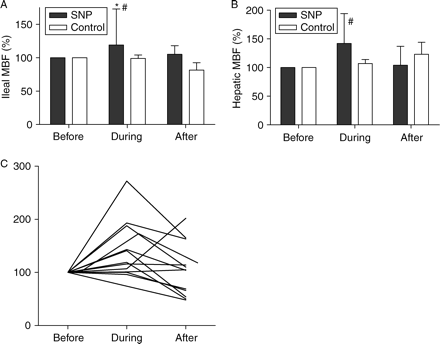
Relative (a) ileal and (b) hepatic MBF changes during drug administration. Endotoxic pigs were resuscitated with either SNP or NS (control). Relative changes before, during continuous infusion, and 30 min after discontinuing administration of the test drug. All MBFs were set at 100% before administration of the test drug. MBFs were expressed as percentage of their values before drug administration. (c) Individual ileal MBF during SNP infusion. *P<0.05 (significant difference between values, before and after drug administration); #P<0.05 (significant difference from control).
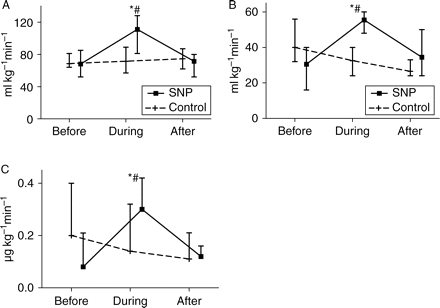
Relative changes of (a) CI, (b) fluid replacement, and (c) norepinephrine infusions during drug administration. Endotoxic pigs were resuscitated with either SNP or NS (control). Relative changes before, during continuous infusion, and 30 min after discontinuing administration of the test drug. *P<0.05 (significant difference between values before and after drug administration); #P<0.05 (significant difference from control).
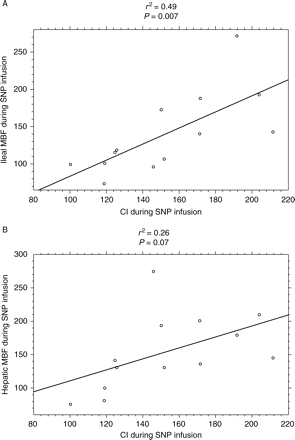
Relationship between MBF and CI variables in endotoxic pigs during SNP infusion. (a) There is a poor correlation between ileal MBF and CI (r2=0.49, P=0.007); (b) there is no correlation between hepatic MBF and CI (r2=0.26, P=0.07). MBFs were expressed as percentage of their values, between before and after SNP administration. CI, cardiac index.
Microcirculatory, haemodynamic, and acid–base variables during either SNP or control infusion. Pigs were resuscitated with either SNP or NS (control). All microcirculatory blood flows were set at 100% before administration of the test drug. MBF, microcirculatory blood flows were expressed as percentage of their values before drug administration). CI, cardiac index; MAP, mean arterial pressure; PCWP, pulmonary capillary wedge pressure; CVP, central venous pressure; MPAP, mean pulmonary arterial pressure; Pco2 gap, gradient between arterial and ileal mucosal Pco2. Data presented as median (inter-quartile range). *P<0.05 (significant difference between before and after administration); †P<0.05 (different from control)
Time . | Before SNP . | During SNP . | 30 min after SNP . | Before control . | During control . | 30 min after control . |
---|---|---|---|---|---|---|
Hepatic MBF (%) | 100 | 142 (123–196)* | 104 (88–137) | 100 | 107 (100–134) | 123 (123–177) |
Ileal MBF (%) | 100 | 119 (101–176)*,† | 105 (63–129) | 100 | 99 (92–104) | 82 (73–94) |
CI (ml kg−1 min−1) | 68 (52–85) | 111 (81–128)*,† | 71 (63–91) | 69 (64–81) | 72 (57–81) | 74 (62–87) |
MAP (mm Hg) | 70 (66–71) | 66 (65–71)* | 71 (65–72) | 70 (66–72) | 65 (65–72) | 69 (66–71) |
Heart rate (beats min−1) | 115 (100–123) | 129 (129–139)* | 114 (107–119) | 116 (107–126) | 115 (104–136) | 118 (106 123) |
PCWP (mm Hg) | 8.5 (7–10) | 8 (6–8) | 9 (7–10) | 8 (5–9) | 9 (6–10) | 8 (6–90) |
CVP (mm Hg) | 8 (5–9) | 6.5 (5–10) | 7.5 (5–9) | 6.5 (5–8) | 6.5 (6–9) | 6 (5–8) |
MPAP (mm Hg) | 31.5 (24–38) | 29 (24–34) | 32 (25–33) | 32 (24–35) | 30 (24–33) | 29 (24–33) |
I.V. fluids (ml kg−1 h−1) | 31 (24–40) | 55 (48–61)*,† | 34 (24–50) | 40 (32–56) | 33 (24–40) | 27 (24–33) |
Norepinephrine (µg kg−1 min−1) | 0.08 (0.00–0.21) | 0.31 (0.23–0.42)*,† | 0.12 (0.03–0.16) | 0.20 (0.03–0.40) | 0.14 (0.00–0.32) | 0.11 (0.07–0.21) |
Arterial lactate (mmol litre−1) | 2.83 (2.39–3.05) | 2.41 (1.92–2.95) | 2.59 (2.31–2.84) | 2.57 (2.16–2.99) | 2.53 (2.06–2.99) | 2.83 (2.02–3.46) |
Mixed venous lactate (mmol litre−1) | 2.36 (1.82–2.79) | 2.11 (1.59–2.30) | 2.34 (2.06–2.43) | 2.34 (1.95–2.64) | 2.26 (1.85–2.52) | 2.57 (1.79–2.82) |
Portal venous lactate (mmol litre−1) | 2.45 (2.10–2.91) | 2.41 (2.13–2.70) | 2.41 (2.19–3.41) | 2.02 (2.02–2.86) | 2.55 (2.16–2.79) | 2.45 (2.10–2.84) |
Pco2 gap (Torr) | 22 (16–13) | 23 (21–32) | 23 (20–26) | 22 (18–27) | 25 (17–28) | 25.5 (18–30) |
Time . | Before SNP . | During SNP . | 30 min after SNP . | Before control . | During control . | 30 min after control . |
---|---|---|---|---|---|---|
Hepatic MBF (%) | 100 | 142 (123–196)* | 104 (88–137) | 100 | 107 (100–134) | 123 (123–177) |
Ileal MBF (%) | 100 | 119 (101–176)*,† | 105 (63–129) | 100 | 99 (92–104) | 82 (73–94) |
CI (ml kg−1 min−1) | 68 (52–85) | 111 (81–128)*,† | 71 (63–91) | 69 (64–81) | 72 (57–81) | 74 (62–87) |
MAP (mm Hg) | 70 (66–71) | 66 (65–71)* | 71 (65–72) | 70 (66–72) | 65 (65–72) | 69 (66–71) |
Heart rate (beats min−1) | 115 (100–123) | 129 (129–139)* | 114 (107–119) | 116 (107–126) | 115 (104–136) | 118 (106 123) |
PCWP (mm Hg) | 8.5 (7–10) | 8 (6–8) | 9 (7–10) | 8 (5–9) | 9 (6–10) | 8 (6–90) |
CVP (mm Hg) | 8 (5–9) | 6.5 (5–10) | 7.5 (5–9) | 6.5 (5–8) | 6.5 (6–9) | 6 (5–8) |
MPAP (mm Hg) | 31.5 (24–38) | 29 (24–34) | 32 (25–33) | 32 (24–35) | 30 (24–33) | 29 (24–33) |
I.V. fluids (ml kg−1 h−1) | 31 (24–40) | 55 (48–61)*,† | 34 (24–50) | 40 (32–56) | 33 (24–40) | 27 (24–33) |
Norepinephrine (µg kg−1 min−1) | 0.08 (0.00–0.21) | 0.31 (0.23–0.42)*,† | 0.12 (0.03–0.16) | 0.20 (0.03–0.40) | 0.14 (0.00–0.32) | 0.11 (0.07–0.21) |
Arterial lactate (mmol litre−1) | 2.83 (2.39–3.05) | 2.41 (1.92–2.95) | 2.59 (2.31–2.84) | 2.57 (2.16–2.99) | 2.53 (2.06–2.99) | 2.83 (2.02–3.46) |
Mixed venous lactate (mmol litre−1) | 2.36 (1.82–2.79) | 2.11 (1.59–2.30) | 2.34 (2.06–2.43) | 2.34 (1.95–2.64) | 2.26 (1.85–2.52) | 2.57 (1.79–2.82) |
Portal venous lactate (mmol litre−1) | 2.45 (2.10–2.91) | 2.41 (2.13–2.70) | 2.41 (2.19–3.41) | 2.02 (2.02–2.86) | 2.55 (2.16–2.79) | 2.45 (2.10–2.84) |
Pco2 gap (Torr) | 22 (16–13) | 23 (21–32) | 23 (20–26) | 22 (18–27) | 25 (17–28) | 25.5 (18–30) |
Microcirculatory, haemodynamic, and acid–base variables during either SNP or control infusion. Pigs were resuscitated with either SNP or NS (control). All microcirculatory blood flows were set at 100% before administration of the test drug. MBF, microcirculatory blood flows were expressed as percentage of their values before drug administration). CI, cardiac index; MAP, mean arterial pressure; PCWP, pulmonary capillary wedge pressure; CVP, central venous pressure; MPAP, mean pulmonary arterial pressure; Pco2 gap, gradient between arterial and ileal mucosal Pco2. Data presented as median (inter-quartile range). *P<0.05 (significant difference between before and after administration); †P<0.05 (different from control)
Time . | Before SNP . | During SNP . | 30 min after SNP . | Before control . | During control . | 30 min after control . |
---|---|---|---|---|---|---|
Hepatic MBF (%) | 100 | 142 (123–196)* | 104 (88–137) | 100 | 107 (100–134) | 123 (123–177) |
Ileal MBF (%) | 100 | 119 (101–176)*,† | 105 (63–129) | 100 | 99 (92–104) | 82 (73–94) |
CI (ml kg−1 min−1) | 68 (52–85) | 111 (81–128)*,† | 71 (63–91) | 69 (64–81) | 72 (57–81) | 74 (62–87) |
MAP (mm Hg) | 70 (66–71) | 66 (65–71)* | 71 (65–72) | 70 (66–72) | 65 (65–72) | 69 (66–71) |
Heart rate (beats min−1) | 115 (100–123) | 129 (129–139)* | 114 (107–119) | 116 (107–126) | 115 (104–136) | 118 (106 123) |
PCWP (mm Hg) | 8.5 (7–10) | 8 (6–8) | 9 (7–10) | 8 (5–9) | 9 (6–10) | 8 (6–90) |
CVP (mm Hg) | 8 (5–9) | 6.5 (5–10) | 7.5 (5–9) | 6.5 (5–8) | 6.5 (6–9) | 6 (5–8) |
MPAP (mm Hg) | 31.5 (24–38) | 29 (24–34) | 32 (25–33) | 32 (24–35) | 30 (24–33) | 29 (24–33) |
I.V. fluids (ml kg−1 h−1) | 31 (24–40) | 55 (48–61)*,† | 34 (24–50) | 40 (32–56) | 33 (24–40) | 27 (24–33) |
Norepinephrine (µg kg−1 min−1) | 0.08 (0.00–0.21) | 0.31 (0.23–0.42)*,† | 0.12 (0.03–0.16) | 0.20 (0.03–0.40) | 0.14 (0.00–0.32) | 0.11 (0.07–0.21) |
Arterial lactate (mmol litre−1) | 2.83 (2.39–3.05) | 2.41 (1.92–2.95) | 2.59 (2.31–2.84) | 2.57 (2.16–2.99) | 2.53 (2.06–2.99) | 2.83 (2.02–3.46) |
Mixed venous lactate (mmol litre−1) | 2.36 (1.82–2.79) | 2.11 (1.59–2.30) | 2.34 (2.06–2.43) | 2.34 (1.95–2.64) | 2.26 (1.85–2.52) | 2.57 (1.79–2.82) |
Portal venous lactate (mmol litre−1) | 2.45 (2.10–2.91) | 2.41 (2.13–2.70) | 2.41 (2.19–3.41) | 2.02 (2.02–2.86) | 2.55 (2.16–2.79) | 2.45 (2.10–2.84) |
Pco2 gap (Torr) | 22 (16–13) | 23 (21–32) | 23 (20–26) | 22 (18–27) | 25 (17–28) | 25.5 (18–30) |
Time . | Before SNP . | During SNP . | 30 min after SNP . | Before control . | During control . | 30 min after control . |
---|---|---|---|---|---|---|
Hepatic MBF (%) | 100 | 142 (123–196)* | 104 (88–137) | 100 | 107 (100–134) | 123 (123–177) |
Ileal MBF (%) | 100 | 119 (101–176)*,† | 105 (63–129) | 100 | 99 (92–104) | 82 (73–94) |
CI (ml kg−1 min−1) | 68 (52–85) | 111 (81–128)*,† | 71 (63–91) | 69 (64–81) | 72 (57–81) | 74 (62–87) |
MAP (mm Hg) | 70 (66–71) | 66 (65–71)* | 71 (65–72) | 70 (66–72) | 65 (65–72) | 69 (66–71) |
Heart rate (beats min−1) | 115 (100–123) | 129 (129–139)* | 114 (107–119) | 116 (107–126) | 115 (104–136) | 118 (106 123) |
PCWP (mm Hg) | 8.5 (7–10) | 8 (6–8) | 9 (7–10) | 8 (5–9) | 9 (6–10) | 8 (6–90) |
CVP (mm Hg) | 8 (5–9) | 6.5 (5–10) | 7.5 (5–9) | 6.5 (5–8) | 6.5 (6–9) | 6 (5–8) |
MPAP (mm Hg) | 31.5 (24–38) | 29 (24–34) | 32 (25–33) | 32 (24–35) | 30 (24–33) | 29 (24–33) |
I.V. fluids (ml kg−1 h−1) | 31 (24–40) | 55 (48–61)*,† | 34 (24–50) | 40 (32–56) | 33 (24–40) | 27 (24–33) |
Norepinephrine (µg kg−1 min−1) | 0.08 (0.00–0.21) | 0.31 (0.23–0.42)*,† | 0.12 (0.03–0.16) | 0.20 (0.03–0.40) | 0.14 (0.00–0.32) | 0.11 (0.07–0.21) |
Arterial lactate (mmol litre−1) | 2.83 (2.39–3.05) | 2.41 (1.92–2.95) | 2.59 (2.31–2.84) | 2.57 (2.16–2.99) | 2.53 (2.06–2.99) | 2.83 (2.02–3.46) |
Mixed venous lactate (mmol litre−1) | 2.36 (1.82–2.79) | 2.11 (1.59–2.30) | 2.34 (2.06–2.43) | 2.34 (1.95–2.64) | 2.26 (1.85–2.52) | 2.57 (1.79–2.82) |
Portal venous lactate (mmol litre−1) | 2.45 (2.10–2.91) | 2.41 (2.13–2.70) | 2.41 (2.19–3.41) | 2.02 (2.02–2.86) | 2.55 (2.16–2.79) | 2.45 (2.10–2.84) |
Pco2 gap (Torr) | 22 (16–13) | 23 (21–32) | 23 (20–26) | 22 (18–27) | 25 (17–28) | 25.5 (18–30) |
Acid–base and metabolic variables before and after drug administration
Arterial pH, bicarbonate production, and mixed venous oxygen saturation transiently decreased, whereas arterial lactate and Pco2 gap transiently increased before drug administration at T120 min. Portal vein oxygen saturation, pH, lactate, and bicarbonate did not change. Oxygen delivery and oxygen consumption did not change (data not shown). Haemoglobin level was stable during the whole experiment (P=0.502). Acid–base and metabolic variables before randomization are presented in Table 4. During drug administration, Pco2 gap, oxygen saturation and lactate of arterial, mixed venous and portal blood samples did not differ between the two groups (Table 3).
Acid–base and biological variables before drug administration. Sepsis was induced at T0 min. Data at T120 min represent variables before drug administration. Pco2 gap, gradient between arterial and ileal mucosal Pco2. Data are presented as median (inter-quartile range)
Time (min) . | T0 . | T120 . |
---|---|---|
Arterial pH | 7.46 (7.41–7.48) | 7.42 (7.37–7.44) |
Bicarbonates (mmol litre−1) | 27.5 (26–28) | 27 (26–28) |
Arterial lactate (mmol litre−1) | 2.53 (2.25–2.89) | 2.89 (2.50–3.48) |
Mixed venous lactate | 2.48 (2.06–2.72) | 2.44 (2.27–3.10) |
Portal venous lactate | 2.78 (2.21–3.02) | 2.56 (2.14–3.05) |
Pco2 gap (Torr) | 18 (14–22) | 22 (13–31) |
Mixed venous oxygen saturation (Svmo2) (%) | 83 (77–86) | 79 (69–87) |
Haemoglobin (g litre−1) | 89 (86–98) | 93 (87–96) |
Time (min) . | T0 . | T120 . |
---|---|---|
Arterial pH | 7.46 (7.41–7.48) | 7.42 (7.37–7.44) |
Bicarbonates (mmol litre−1) | 27.5 (26–28) | 27 (26–28) |
Arterial lactate (mmol litre−1) | 2.53 (2.25–2.89) | 2.89 (2.50–3.48) |
Mixed venous lactate | 2.48 (2.06–2.72) | 2.44 (2.27–3.10) |
Portal venous lactate | 2.78 (2.21–3.02) | 2.56 (2.14–3.05) |
Pco2 gap (Torr) | 18 (14–22) | 22 (13–31) |
Mixed venous oxygen saturation (Svmo2) (%) | 83 (77–86) | 79 (69–87) |
Haemoglobin (g litre−1) | 89 (86–98) | 93 (87–96) |
Acid–base and biological variables before drug administration. Sepsis was induced at T0 min. Data at T120 min represent variables before drug administration. Pco2 gap, gradient between arterial and ileal mucosal Pco2. Data are presented as median (inter-quartile range)
Time (min) . | T0 . | T120 . |
---|---|---|
Arterial pH | 7.46 (7.41–7.48) | 7.42 (7.37–7.44) |
Bicarbonates (mmol litre−1) | 27.5 (26–28) | 27 (26–28) |
Arterial lactate (mmol litre−1) | 2.53 (2.25–2.89) | 2.89 (2.50–3.48) |
Mixed venous lactate | 2.48 (2.06–2.72) | 2.44 (2.27–3.10) |
Portal venous lactate | 2.78 (2.21–3.02) | 2.56 (2.14–3.05) |
Pco2 gap (Torr) | 18 (14–22) | 22 (13–31) |
Mixed venous oxygen saturation (Svmo2) (%) | 83 (77–86) | 79 (69–87) |
Haemoglobin (g litre−1) | 89 (86–98) | 93 (87–96) |
Time (min) . | T0 . | T120 . |
---|---|---|
Arterial pH | 7.46 (7.41–7.48) | 7.42 (7.37–7.44) |
Bicarbonates (mmol litre−1) | 27.5 (26–28) | 27 (26–28) |
Arterial lactate (mmol litre−1) | 2.53 (2.25–2.89) | 2.89 (2.50–3.48) |
Mixed venous lactate | 2.48 (2.06–2.72) | 2.44 (2.27–3.10) |
Portal venous lactate | 2.78 (2.21–3.02) | 2.56 (2.14–3.05) |
Pco2 gap (Torr) | 18 (14–22) | 22 (13–31) |
Mixed venous oxygen saturation (Svmo2) (%) | 83 (77–86) | 79 (69–87) |
Haemoglobin (g litre−1) | 89 (86–98) | 93 (87–96) |
Discussion
Several aspects of the methods used warrant comment. We used a large animal model to have easy access to global and regional blood flow measurements. Measurements of the MBF by LDF and Pco2 gap by gas tonometry were made in the terminal ileum, which allows direct in situ evaluation of the relationship between splanchnic MBF and acid–base status.
As this is a cross-over study in which the animals were their own controls, certain precautions were taken to assure model stability and reproducibility and limiting of any bias (Table 3). In this regard, it is important to specify that there were neither carry-over effect nor period effect during SNP infusion (P<0.05). These properties allowed us to compare SNP with NS (control) for each data set. Furthermore, as the effect of SNP disappears quickly 30 min of washout was sufficient. Moreover, animals were randomized to receive either SNP or control infusion first. Again, before administration of either SNP or control (at T120 and T180), systemic haemodynamic parameters (MAP, PAOP, and CVP) were maintained within comparable levels by adequate adjustment of fluid replacement and norepinephrine infusions, thus preventing any macrocirculatory bias on the microcirculation. Furthermore, during the duration of this experiment, we were able to measure ileal MBF by LDF continuously at one point by fixing the exposed ileal mucosa on a platform (Methods). This eliminates any artifact caused by respiratory or peristaltic movements. These measurement conditions were reproducible and reliable. Nevertheless, this approach may be less physiological because the ileum is exteriorized and it is difficult to maintain constant temperature. The LDF tracing was clearly physiological with rhythmical oscillations (vasomotion) and these become more obvious after onset of sepsis. This was reproducible during the whole period of the experiment (Fig. 2). Again, to limit minor variations, LDV reading was averaged over 2 min. Haemoglobin was stable during the study, as LDF probe detects movement of red blood cells in relation to fixed tissues; therefore, avoiding any false readings resulting from modification of red blood cell count.
One limitation of this study is that animals were anaesthetized throughout the experiment. However, general anaesthesia allowed us to obtain accurate measurements, in particular laser Doppler tracing, which is very sensitive to movement. Of course, this is a confounding factor on all measurements, because anaesthetic drugs might have directly or indirectly affected the measured variables. However, all animals in the two groups were anaesthetized in the same manner and both groups should be comparable. Moreover, patients are often anaesthetized in the early phase of septic shock due to problems with consciousness and respiratory failure.
Infusion of live P. aeruginosa induced haemodynamic changes that are characteristic of a hypodynamic septic shock, with a reduction of cardiac output and sustained systemic arterial hypotension (Fig. 5). Resuscitation converted hypodynamic septic shock into normotensive, and normodynamic shock by adequate fluid and norepinephrine management, therefore restoring baseline macrocirculatory values mimicking resuscitation seen in man. During hypodynamic shock, there was hepatic and ileal mucosal microcirculatory hypoperfusion at T30 min. Although ileal mucosal MBF apparently improved at T60 min, it progressively deteriorated when we tested both drugs (Table 2) at T120 min (84% of baseline) and further at T180 min (75% of baseline), despite intensive resuscitation. It should be remembered that values at T120 and T180 min were set at 100% before administration of the test drug (Table 3). Hepatic MBF was progressively increased with resuscitation (Fig. 6), which can be explained in part by hepatic artery buffer response,17 in which hepatic arterial blood flow increases during the early stage of sepsis. Our results confirm that hepatic MBF increases during early phase of severe sepsis.
We found that during administration of SNP, ileal mucosal MBF (Fig. 7) significantly increased (P=0.013). A recent study showed the effect of l-arginine (the physiological precursor of NO synthesis) in association with a vasopressor (vasopressin or norepinephrine) on villus microcirculation.18 However in that study, a potential increase in CI (which was not measured) may have explained these results. In our study, due to the poor correlation between CI and ileal mucosal MBF (r2=0.49), this increase in ileal mucosal MBF cannot be explained exclusively by an increase in cardiac output but rather to a direct vasodilatory effect of SNP on the ileal mucosal microcirculation. An interesting finding is that despite adequate fluid replacement [40 ml kg−1 h−1 (33–68) at T120 and 40 ml kg−1 h−1 (23–47) at T180] and normal filling pressures (comparable with baseline), the administration of SNP decreased CVP, PAOP, and MAP. This required additional volume loading and norepinephrine infusion. It might have been better if both groups had been resuscitated in the same manner, thereby excluding the possible effects of additional fluid replacement and norepinephrine on the ileal mucosal MBF. However, our aim was to observe the vasodilatory effect of SNP on the microcirculation in association with conventional systemic treatment of septic shock. Interestingly, Nakajima and colleagues18 did not show an increase in villus microcirculation with norepinephrine. Moreover, Lagoa and colleagues6 showed that large volume infusion alone was not able to correct mucosal ischaemia.
The dose of SNP (5 µg kg−1 min−1) was chosen as mean of the average doses of SNP (0.5–10 µg kg−1 min−1) recommended for therapeutic indications (arterial hypertension, anaesthesia, and cardiology). When we formulated our hypothesis, we did not know the optimal dose that might improve the target parameters and therefore we chose a dose that has been previously used for other indications in the clinical setting. The challenge for the future is to test the minimal dose of SNP that improves the microcirculation while avoiding fluid loading or norepinephrine infusion.
It should be mentioned that all animals received norepinephrine together with crystalloids. This model closely approximates the clinical situation in a resuscitated septic patient who maintains normal arterial pressure yet progresses to organ failure. One limitation of this study is that we did not investigate other microcirculatory areas (e.g. renal), which could have been altered by norepinephrine infusion. However, perfusion pressure seems to be one of the keys to maintaining renal blood flow, so that MBF seems not to decrease if MAP is maintained at 65 mm Hg with norepinephrine.19 Additional fluid loading could have deleterious effects as, for example, hydrostatic pulmonary oedema. Nevertheless, this pathology depends on filling pressures (CVP and PAOP), which are not raised during SNP infusion.20 It is possible that the additional fluid is destined for the capillaries but this will require further experimentation.
In order to better define the impact of SNP on splanchnic MBF during sepsis, it would have been more elegant to study a sham group (non-septic animal with NS) and a non-septic group treated with SNP. Nevertheless, as each animal was its own control, we may postulate that the surgical procedure was comparable between our two groups.
On the basis of the idea that supplementation of NO would promote microcirculatory recruitment and perfusion, several NO donors have been tested in relation to sepsis in experimental animals, and many studies indicate the important role of NO in maintaining microcirculatory integrity. In this regard, during endotoxaemia, SNP attenuated the detrimental effects of LPS on liver microcirculation in rats21 and increased hepatic arterial blood flow in pigs.22 Spronk and colleagues23 performed an observational study on sublingual microcirculation in septic patients by orthogonal polarization spectral imaging (OPS). Their results showed that administration of nitroglycerin 0.5 mg, while lowering arterial pressure somewhat, resulted in enhanced sublingual MBF. Zhang and colleagues24 observed that the NO donor 3-morpholinosyndnonimine (SIN-1) increased hepatic, portal, and mesenteric blood flow, without deleterious effects on arterial pressure, and increased tissue oxygen extraction early during endotoxic shock, whereas NOS inhibitor l-NMMA decreased regional hepatosplanchnic blood flow. Splanchnic microcirculation was not measured. De Backer and colleagues25 used OPS to investigate the sublingual microcirculation in patients with severe sepsis. They found that the density of all vessels was significantly reduced, and the proportion of perfused small vessels was reduced in patients with sepsis vs healthy volunteers. Topical application of acetylcholine (endothelium-dependent vasodilator) totally reversed these alterations. Collectively, these studies support a beneficial role for NO donors in reversing the deleterious effects of sepsis on the microcirculation. Accordingly, there might be a place for an NO donor in early treatment of septic shock in order to open the microcirculation and consequently to improve tissue ischaemia and regional organ dysfunction. This will require rigorous testing in experiments using chronic models of sepsis.
Our results have to be tempered by a recent study, which showed that l-arginine increased mortality in a chronic model of sepsis, even at low dose associated with N-acetyl cysteine.26 One of the explanations by the authors was activation of NO pathway. However, l-arginine affects other pathways and the actions cannot be strictly linked to (NO) donor activity.
There are several possible explanations for the absence of metabolic variation during drug administration. First, the period of drug infusion was too short to show a difference between the tested drugs. However, a longer period of observation might have induced model instability rendering the groups incomparable. Secondly, this lack of difference before and during NO infusion might be attributed to paradoxical effects, i.e. even if NO increases ileal MBF and therefore potentially improves cellular metabolism, NO could have aggravated cellular dysoxia directly, particularly by its effect on mitochondria.27 Thirdly, adequate resuscitation might have limited metabolic deterioration, thereby masking potential beneficial effects of the NO donor via vasodilatory effects.
Interestingly, portal and systemic lactate did not differ in this experiment, in spite of ileal mucosal hypoperfusion. This supports the hypothesis that lactic acidosis during sepsis is not always the result of tissue hypoperfusion or increased levels of tissue hypoxia, rather this might be caused by the so-called cytopathic hypoxia,28,29 despite normal or even supranormal Po2 values in the vicinity of mitochondria within the cells. It is generally accepted that hypoxia is not a major mechanism for regional lactate production during sepsis and that lactate released from the hepatosplanchnic bed is uncommon in septic patients.30 This was further confirmed by Levy and colleagues28 using in vivo microdialysis, revealing skeletal muscle as a leading source of lactate production through exaggerated aerobic glycolysis rather than hypoxia in patients with septic shock. Recently, in resuscitated models of septic shock, lactates were not increased, despite a long observation period.26
Rivers and colleagues31 evaluated the efficacy of 6 h of early goal-directed therapy for severe sepsis and septic shock before admission to the intensive care unit. This approach involves adjustments of cardiac preload, afterload, and contractility to balance oxygen delivery with oxygen demand. These patients had higher central venous oxygen saturation, lower lactate concentration, and less severe organ dysfunction and better mortality than those patients assigned to standard therapy. Therefore, the clinical course of patients who develop multiple organ failure is set early in the resuscitation process. Accordingly, the strategy of resuscitation should evolve and fight against microcirculatory and mitochondrial distress syndrome to decrease the severity of the shock insult. In this regard, vasodilators such as SNP might have a place.
Conclusion
Our data suggest that impaired microcirculatory perfusion in early severe sepsis can be counteracted by cautious i.v. administration of SNP (this should not be attempted without continuous monitoring for substantial hypovolaemia), despite normal arterial pressure after resuscitation.
Acknowledgements
We thank F. Arnal for technical assistance, Dr J. Beissel for checking the manuscript, and Martine Rougier, Annie Martra, and Marie Phan for bacterial preparation.