-
PDF
- Split View
-
Views
-
Cite
Cite
Katherine B Lininger, Rebecca Lave, River restoration can increase carbon storage but is not yet a suitable basis for carbon credits, BioScience, Volume 74, Issue 10, October 2024, Pages 717–724, https://doi.org/10.1093/biosci/biae083
- Share Icon Share
Abstract
Increasing organic carbon storage in river corridors (channels and floodplains) is a potential cobenefit of some river restoration approaches, raising the possibility of using restoration to produce carbon credits and, therefore, increase restoration funding. However, the uncertainty already associated with existing carbon credits is compounded in river corridors, which are dynamic on daily, seasonal, annual, and longer timescales. We currently do not know how much river restoration approaches could increase carbon storage or how significant increased organic carbon storage from restoration would be compared with other forms of climate mitigation. We also do not know whether river corridor carbon credits could meet market needs for quickly established, stable, and simple credits. Therefore, we argue that biophysical and political economic uncertainties make river corridor restoration carbon credits currently unfeasible but that research on river restoration projects would demonstrate whether restoration carbon credits could be feasible in the future.
Increasing evidence indicates that rivers are not passive pipes carrying carbon from the land to the ocean but, instead, are active participants in organic carbon (OC) fluxes and storage (Cole et al. 2007, Sutfin et al. 2016, Wohl et al. 2017). Storage and fluxes of carbon in inland waters (i.e., the land-to-ocean aquatic continuum) are not well constrained, particularly with respect to burial and potential storage in floodplains (Regnier et al. 2022, Battin et al. 2023), but there is evidence that river corridors (the active channel and floodplain) can have large OC stocks per area relative to surrounding terrestrial-only ecosystems (Norman et al. 2022, Wohl and Knox 2022). OC stocks likely have been reduced by anthropogenic impacts, including wood removal, flow reduction and regulation, floodplain disconnection, and the removal of beavers (Hanberry et al. 2015, Wohl et al. 2017, Lininger and Polvi 2020). Therefore, the types of river restoration intended to address these anthropogenic impacts could also increase carbon storage as a cobenefit of river restoration (Hinshaw and Wohl 2021, Norman et al. 2022, Skidmore and Wheaton 2022).
The recognition that river corridors are areas of OC storage (in floodplain soil, downed wood, and riparian vegetation) has led to discussions of developing river corridor carbon credits (Matzek et al. 2015, American Southwest Carbon Marketplace Workgroup 2023, Virridy 2024). Such credits could lead to increased funding for river restoration in the United States, which has been stuck below $5 billion per year since the early 2000s (Bernhardt et al. 2005, Doyle 2020). River corridor carbon credits have also been discussed in Australia (Williams et al. 2013, Settre et al. 2019) and the European Union (Vermaat et al. 2016, Bechauf et al. 2023). We, the authors, have different professional backgrounds—Lininger in fluvial geomorphology and river science, Lave in stream restoration and environmental markets—and we have both observed increasing consideration of the possibility of river corridor carbon credits. But as we address in the “Political economic uncertainties for river corridor carbon credits” section below, the multiple sources of uncertainty associated with carbon credits in agricultural soil and forests (West et al. 2020, Haya et al. 2023) are compounded in river corridors because of their complexity; river corridors change on daily, seasonal, annual, and longer timescales. Therefore, there are major uncertainties about how much river restoration approaches could increase carbon storage, and if so, how significant they would be compared with other forms of climate mitigation (and compared with methane releases from biophysical changes that might counter some of the benefits of carbon sequestration). Furthermore, as recent articles on agricultural soil carbon and forest carbon credits (West et al. 2020, Haya et al. 2023, but see Guizar-Coutiño et al. 2022) and recent discussion of stream mitigation credits (Lave and Doyle 2021) have made clear, contradictions between economic and environmental goals inherent in ecosystem service markets complicate their scientific credibility.
Therefore, although we agree that it may be possible to develop river corridor carbon credits, we believe it is premature to do so. At present, there is too much uncertainty about river corridor carbon dynamics’ and restoration practices’ ability to increase carbon storage to justify river corridor carbon credits. Given that process-based river restoration is increasingly widespread, it makes more sense to investigate OC storage as a potential cobenefit of restoration approaches whose non–OC storage benefits have already been demonstrated, such as riparian reforestation, floodplain reconnection, beaver reintroduction, and the addition of wood to river corridors (Beechie et al. 2010, Bellmore and Baxter 2014, Pollock et al. 2014, Wohl 2016). In the present article, we first review biophysical uncertainties about how much restoration could increase river corridor OC storage. We then discuss the political and economic uncertainties in markets for ecosystem services (e.g., carbon markets). We end by summarizing the need for additional research prior to considering river corridor restoration as a means of producing carbon credits.
Biophysical uncertainties related to river corridor carbon storage
River corridors transport, transform, and store OC (Cole et al. 2007, Wohl et al. 2017), with the largest OC storage contained in floodplain soil or sediment, vegetation, and downed wood (figure 1; Sutfin et al. 2016). OC in floodplain sediment or soil comes from deposition via floodplain or via the accumulation of organic matter into the subsurface (Cierjacks et al. 2011, Appling et al. 2014, Lininger et al. 2018, Hupp et al. 2019). Dead, downed wood within the river corridor can be from upstream or can be recruited locally to the channel or floodplain surface from riparian forests or hillslopes (Wohl et al. 2019, Lininger et al. 2021). Floodplain forests are generally more productive than upland forests in similar climates (Naiman and Décamps 1997), and OC buried in floodplain sediment can be protected from decomposition (Blazejewski et al. 2009, Chaopricha and Marín-Spiotta 2014, D'Elia et al. 2017). Despite recent work indicating that river corridors store a disproportionate amount of OC relative to their area (Wohl and Knox 2022), larger-scale carbon budgets rarely account for them separately (Regnier et al. 2013, 2022, Tian et al. 2023). In addition, there is significant uncertainty in the magnitude of carbon fluxes into storage within floodplains, reservoirs, and lakes, with estimates ranging from 0.2 to 1.6 petagrams of carbon per year (Regnier et al. 2013, Drake et al. 2018, Regnier et al. 2022). In the present article, we do not provide a full review of carbon dynamics in river corridors (see Sutfin et al. 2016, Wohl et al. 2017, and Battin et al. 2023) but, instead, summarize the controls on floodplain carbon storage to discuss the potential for river corridor carbon credits. We also do not fully discuss inorganic carbon dynamics, because river restoration actions have the potential to strongly influence biogenic OC storage (e.g., in vegetation, soil, and downed wood), although we acknowledge that inorganic carbon fluxes can be significant (Regnier et al. 2022, Battin et al. 2023).
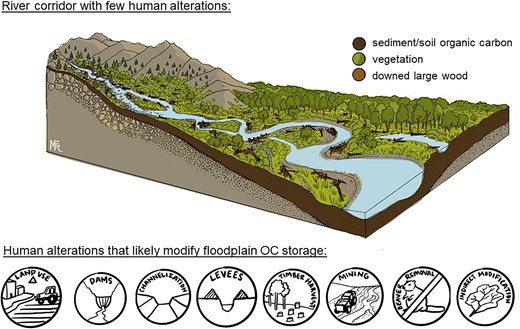
Conceptual figure of relatively unaltered floodplains (top) showing organic carbon in sediment and soil, vegetation, and downed large wood and human impacts (bottom) demonstrating direct modifications of floodplains, as well as indirect modifications to water, sediment, and organic carbon yields to river corridors that likely influence organic carbon storage. Illustration: Mariah Richards.
OC storage in river corridors is in part controlled by climate and geology. Climate influences the rates of primary production and carbon fixation, decomposition, and respiration (Jobbágy and Jackson 2000, Chapin et al. 2012). Geology influences the composition and character of river corridor sediment, which determines its ability to retain OC (Rasmussen et al. 2018, Slessarev et al. 2022). In the present article, we focus on biogenic OC and not on rock-derived petrogenic OC, but, petrogenic OC can contribute to the total OC content of sediment depending on basin lithology. However, OC storage can vary substantially within and between river reaches (typically a length of river between tens of meters to kilometers in extent, depending on the river size), meaning that drainage basin climate and geology are not enough to predict OC storage at a given location (Appling et al. 2014, Lininger et al. 2018, Sutfin et al. 2021). For example, bedrock-dominated reaches with limited areas for fine sediment storage and vegetation growth likely store less OC; similarly, reaches with hillslopes adjacent to channels (more confined) can store less OC than reaches with wider floodplains in mountain river corridors (Sutfin et al. 2021). In addition, river corridors are complex landscapes that rearrange themselves over time through processes such as sediment transport and deposition, channel migration, wood transport and deposition, and flooding events that produce local patches (e.g., geomorphic units such as bars, side channels, older floodplain surfaces) with characteristic grain size distributions, moisture conditions, and vegetation (Stanford et al. 2005, Fryirs and Brierley 2022, Scott et al. 2022). Because grain size, moisture conditions, and vegetation influence OC storage in soil and downed wood, patches can have different OC storage in sediment and wood (Appling et al. 2014, Lininger et al. 2018). Variation in vegetation type and age also result in differences in OC in aboveground biomass in floodplains and influences subsurface carbon storage (Cierjacks et al. 2011, Appling et al. 2014, Güneralp et al. 2014, Lininger et al. 2018, Sutfin et al. 2021).
Because river corridor restoration efforts can modify the controls on OC storage (e.g., grain size distribution, vegetation, soil moisture, and physical complexity), restoration has the potential to modify OC storage. We define river restoration in the present article as any modification of rivers or of river flow or sediment aimed at reestablishing or strengthening existing hydrologic, geomorphic, or ecological processes within degraded watersheds (Wohl et al. 2005). In particular, we emphasize process-based restoration approaches, as was described in Beechie and colleagues (2010). Studies suggest that human modifications (e.g., dams, levees, land use changes, flow alterations, removal of downed wood; figure 1) have reduced OC storage in river corridors (Hanberry et al. 2015, Lininger and Polvi 2020) and that some restoration approaches (such as beaver reintroduction or beaver mimicry structures, riparian reforestation, and channel–floodplain reconnection) might be able to reverse those losses (Bullinger-Weber et al. 2014, Hinshaw and Wohl 2023), even resulting in similar OC storage as unaltered sites in some cases and for some stocks (Matzek et al. 2016).
However, human impacts and the effects of restoration activities on OC storage have not been quantified over larger spatial extents, in diverse environments, or over long time periods, and the complex, variable processes that influence river corridor OC storage make it difficult even to estimate or predict them. For example, it is not yet clear how many soil samples are required to accurately estimate floodplain soil OC stocks across diverse river types (Hinshaw and Wohl 2021), and recent evidence suggests that existing publicly available geospatial data (e.g., soil survey data) may underestimate them (Lininger et al. 2019). Although it is easier to measure OC storage in vegetation and downed wood because these stocks do not require laboratory analyses, accurately estimating total river corridor OC storage is challenging.
Predicting how total OC storage might change with restoration efforts or changing river corridor management requires closing the carbon budget by determining the inputs and outputs of carbon to a river reach over the time period of interest. Doing this requires assessing fluxes of dissolved, particulate, and gaseous carbon and understanding interactions among ecological, biogeochemical, microbial, and geomorphic processes and accounting for variations in fluxes depending on flow level, because high flows can substantially increase OC fluxes (Webster and Meyer 1997, Appling 2012). In addition, changes in one pool of carbon storage may affect other carbon pools (e.g., downed wood can influence dissolved OC export, microbial activity, and gaseous fluxes; Sutfin et al. 2021, Howard et al. 2023). Beaver can increase storage of OC in sediment and aboveground biomass, and OC in sediment in beaver-affected river corridors can persist for multiple decades (Laurel and Wohl 2019). However, whether and how much this increased storage counteracts an observed increase in methane emissions from beaver ponds, a potent greenhouse gas, is not yet clear (Cazzolla Gatti et al. 2018, Nummi et al. 2018). Overall, restoring wetlands likely has a negative radiative forcing (reducing global warming) over time when balancing carbon sequestration and methane emissions (Neubauer 2014, Günther et al. 2020, Nyberg et al. 2022). Methane concentrations—and, therefore, emissions—are higher within streams adjacent to saturated floodplains with high organic matter concentrations (Borges et al. 2019, Rocher-Ros et al. 2023), but determining whether a river corridor reach is a net source or sink of carbon requires measuring storage and fluxes in both the channel and floodplain over the timescale of interest. Closing the carbon budget is therefore difficult, and very few studies quantify all carbon pools and carbon fluxes at the river reach scale and include the entire river corridor (Appling 2012), balancing the carbon budget over timescales relevant to management or carbon markets.
Another key unknown is how long it takes for carbon, particularly in soil, to build up in floodplains. The rates of soil OC sequestration within floodplains and wetlands are highly variable (Sutfin et al. 2016, Craft et al. 2018), and changes in soil OC within floodplains may occur over decades or longer (Van Cleve et al. 1993, Scott and Wohl 2020). Furthermore, the capacity for soil to retain OC over time depends on soil characteristics and the proportion of OC that is mineral associated (and less subject to decomposition and release to the atmosphere) compared with particulate OC (Cotrufo et al. 2019, Georgiou et al. 2022).
Overall, the biophysical basis for earning carbon credits through river corridor restoration is subject to multiple uncertainties, which calls the scientific credibility of river corridor OC credits into question. These uncertainties stem from the difficulty in measuring carbon pools and fluxes, but also from lack of fundamental understanding of OC in dynamic, geomorphically active river corridors. For example, because floodplain surfaces are created and modified by geomorphic processes, how does this geomorphic cycling interact with biogeochemical processes and change OC storage over time? How might disturbances such as floods and anthropogenic climate change affect these processes? It is likely that additional uncertainties (currently unknown unknowns) will emerge as research on carbon in river corridors advances. These questions need to be addressed to understand the timescale of OC changes in river corridors and to determine whether carbon credits are compatible with typical timescales of river management and restoration.
Political economic uncertainties for river corridor carbon credits
The biophysical uncertainties of river corridor carbon credits could perhaps be overcome if carbon markets were scientifically credible and well established. Instead, markets contribute substantial uncertainty of their own, calling the wisdom of river corridor carbon credits into question.
Carbon credits are produced by preventing emission of greenhouse gases or removing them after they have been emitted, and take many different forms, such as reforestation, capturing methane from landfills, and developing alternative energy sources. The markets for carbon credits are a type of market for ecosystem services (MES). MES provide ways to offset environmental damage in one place (e.g., by emitting greenhouse gasses or destroying habitat for an endangered species) by purchasing credits produced via restoration or preservation of comparable environmental goods elsewhere (Business and Biodiversity Offsets Programme 2012, Lave and Robertson 2017), creating a compromise between economic development and environmental protection. Critics have raised many issues about MES, including additionality (would the environmental improvements underlying the credit have been generated anyway?), durability (will the environmental improvements persist over time?), measurability (can we quickly and reliably prove that the supposed improvements actually provide the benefits they claim?), and regulatory capacity (do regulators have the knowledge, time, and funding to effectively manage the economic and ecological aspects of the market? Maron et al. 2012, Quétier et al. 2014, Lave and Doyle 2021).
These and other concerns stem from the overarching struggle that all MES face: how to balance market needs for stable, discrete commodities with the chaotic, dynamic, and interconnected character of most ecosystems (Robertson 2006, Lave and Doyle 2021). It turns out to be surprisingly difficult to turn unruly nature into a cleanly defined commodity and, therefore, to create robust markets that are also scientifically sound. The MES for vernal pool habitat in California provides an instructive example (box 1).
The market for ecosystem services created to protect vernal pool habitat in California is a useful illustration of the conflict between scientific and economic credibility. As was described by Mead (1998, p. 275), the initial market had complex ecologically credible rules that addressed factors including the type and condition of the pool to be damaged or restored and the rarity of species it hosted. Entrepreneurs were not willing to try to produce credits to meet these detailed requirements and the market floundered. Once the vernal pool credits were redefined solely on the basis of acreage destroyed or restored (rather than the suite of characteristics that ecologists considered important), the vernal pool market for ecosystem services became robust (Mead 2008, p. 23). As Fox and Nino-Murcia (2005) explained, this kind of highly simplified credit market is typical in conservation banking.
Carbon markets have demonstrated similar tensions between creating credits that are scientifically sound and establishing a robust market. Carbon credits under the clean development mechanism of the Kyoto Protocol were exactingly specified, and the markets struggled (for a deeper introduction to why carbon markets have been so weak, see the Economist 2012). Some studies have shown modest success in reducing carbon emissions from deforestation through voluntary carbon credit projects (Guizar-Coutiño et al. 2022). However, many scholars and journalists revealed that the credits for sale in both regulatory and voluntary carbon markets are often bogus (West et al. 2020, Haya et al. 2023, Jones and Lewis 2023), issued without proper oversight, and not actually offsetting carbon emissions as claimed. Even the US government recognizes the weakness of current carbon credits (Plumer 2024). It concerns us that the biogeochemical and physical uncertainties of river corridor carbon credits may be even more substantial than these reforestation projects. As was described above, soil OC accrual rates can be variable and develop over relatively long timescales in floodplains. In addition, river corridors are typically dynamic landscapes, with erosion and deposition modifying their physical structure and therefore the spatial distribution of OC storage. Therefore, the biophysical basis for river corridor carbon credits may be even less secure than other carbon credits.
Unlike existing carbon credits, river corridor carbon credits could be integrated into one of the oldest and most economically robust MES in the world: the US stream mitigation banking (SMB) market. Would that institutional stability counteract current uncertainties in river corridor OC dynamics?
SMB allows development projects to physically damage rivers and streams protected under section 404 of the US Clean Water Act as long as that damage is offset via the purchase of mitigation credits. The credits, which currently focus primarily on channel form, are produced mostly by for-profit mitigation companies that acquire the rights to properties with degraded streams and preemptively restore them to produce stream credits (Lave and Doyle 2021).
Although SMB is economically robust, its benefits for river health have been strongly questioned (see chapter 7 in Lave and Doyle 2021) because of the mismatch between market needs for a stable, easily measured commodity and the dynamic, and interconnected nature of river corridors. Although there are recent attempts to address this and create more scientifically credible credits, these efforts are only just getting underway, and their outcomes are uncertain (Meridian Institute 2023). Therefore, although SMB could provide a robust existing market for river corridor carbon credits, the uncertainty of its ecological outcomes and the SMB market's constraints would only compound the uncertainty of OC storage and sequestration described above.
For example, the time scale of SMB is a poor match with the time scale of OC accrual. SMB projects are monitored for no more than 7 years, and often only 3–5 years. OC, whether in soils, vegetation, or downed wood, can take far longer to accumulate, and OC can also decay on these timescales. Under current models, mitigation providers typically donate the land (or the easements) on which stream mitigation projects are located to local land conservancies or parks districts once the credits are sold and the monitoring period is complete. Accruing river corridor carbon credits to sell would require mitigation providers to hold onto and manage projects for substantially longer periods of time—decades rather than years—and it is not clear that the current business model of SMB could be adapted accordingly. The fluctuation of OC storage described in the previous section would further compound the market uncertainty, because credits sold in a previous year cannot be unsold if OC stocks decrease.
Therefore, from both a scientific and economic point of view, creating river corridor carbon credits involves significant, unresolved complications and uncertainties.
Research on river restoration and OC dynamics to reduce uncertainties
Given the uncertainty about how much OC is stored in any given stream reach and whether restoration can increase it over market-relevant timescales, and the tension between economic and scientific credibility in MES, is it worth trying to scale up restoration funding via river corridor carbon credits?
Although it may be in the future, developing river corridor carbon credits is not feasible now, given the current state of knowledge. Instead, study of existing restoration projects that employ techniques with strong potential to increase OC storage and sequestration would enable closing river corridor carbon budgets at the reach scale to determine the potential for carbon credits.
Many restoration projects already employ techniques that may increase OC storage. For example, approaches that restore channel–floodplain reconnection and rewet floodplain environments could encourage deposition of fine sediment, which promotes OC retention, provide saturated locations that slow decomposition, and increase riparian vegetation growth; these processes would likely increase OC storage in soil, wood, and vegetation. Process-based approaches that restore flow regimes in rivers and allow natural flooding or reintroduce beaver (or beaver dam analogues) provide similarly fertile grounds for studying OC storage. Investigating projects such as these in a way that accounts for all fluxes and storage pools would allow us to assess whether they actually increase OC storage and, if so, over what time periods.
Additional research should explore the conditions under which river corridor OC storage increases because of river restoration. Just like a lake level may vary around an average as its inflow and outflow vary over time, OC storage in a river corridor reach may fluctuate around an average in the absence of modifications or disturbance over a market-relevant time period. The implication of a steady-state OC stock at the reach scale would be that river restoration could enhance the floodplain OC reservoir, giving floodplains more capacity to store OC, although that OC may accumulate slowly (figure 2). Provided that the reservoir could fill to a higher steady state over a market-relevant time period, it could then be considered a carbon sink. However, this would necessitate conceptualizing OC storage in river corridors at the reach scale (as opposed to focusing on a smaller areas) because some surfaces may be reworked via erosion and deposition and over market-relevant time periods because OC sequestration rates may vary considerably.
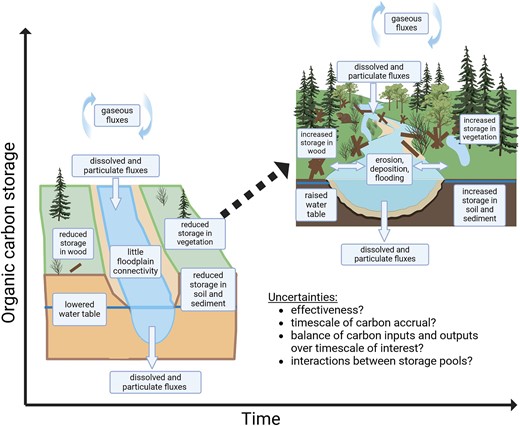
Imagined outcome of restoration actions for enhanced organic carbon storage. A river corridor reach that is incised or with an altered flow regime likely has reduced organic carbon storage in soil or sediment, wood, and vegetation and has the potential for increased average organic carbon storage with differing management or restoration. However, there are significant uncertainties. The arrows in the figure are meant to represent processes and do not imply indications of net fluxes. Image: Created with BioRender.com.
Prematurely pursuing river corridor carbon credits, as some have discussed, could direct climate mitigation funding away from more effective sinks and distract from the urgent need to reduce fossil fuel consumption. By contrast, using existing process-based restoration to examine OC storage has few if any downsides. For example, wood reintroduction can increase habitat for fish and macroinvertebrates (Roni et al. 2015, Pess et al. 2022). If wood reintroduction is created through reforestation in the river corridor, natural wood recruitment, and subsequent vegetation regrowth, increased wood loads within the river corridor would increase the total carbon storage in the river corridor reach and provide the potential for wood burial, which can protect wood from decomposition. Focusing on river corridor OC storage can also promote healthy floodplain soil, which has been overlooked in most restoration projects (Inamdar et al. 2023). Restored and relatively unaffected river corridors also influence biogeochemical cycling—for example, through nitrogen uptake via denitrification (Kaushal et al. 2008).
Furthermore, river restoration approaches with potential to increase OC storage provide crucial climate adaptation benefits, including increased water stored on the landscape, which provides nature-based protection against drought (Norman et al. 2022) and desperately needed thermal refuges for fish and other aquatic organisms as the climate warms (Mejia et al. 2023). In addition, rviers with extensive beaver activity and well connected, wide floodplains provide refuges from wildfire (Fairfax and Whittle 2020) and attenuate fluxes of water and sediment in postwildfire landscapes (Wohl et al. 2022).
Conclusions
Because of the substantial biophysical and economic uncertainties, it is not wise to introduce river corridor carbon credits now. However, many current restoration projects employ techniques with strong potential to increase OC storage. Careful analysis of a selection of these projects could allow for assessment of the timescale of accrual and magnitude of potential carbon sinks. Allocating resources for assessing projects in strategic ways will be important. For example, comparing restoration approaches and their potential for increased carbon storage across different bioclimatic regions will provide guidance about the feasibility of river corridor carbon credits. If research reduced the biophysical uncertainty regarding carbon storage in river corridors and some restoration approaches do indeed increase carbon storage, cautious experimentation with river corridor carbon credits may be justified as a form of climate mitigation, to promote climate adaptation, and to scale up funding for river restoration.
Acknowledgments
This work was funded in part by National Science Foundation awards no. 2237366, no. GSS-1461746, and no. BCS-1213827. We thank Martin Doyle, Laurel Lynch, Dan Scott, and Ellen Wohl for providing insightful and valuable comments on an earlier draft of the article. We also thank two anonymous reviewers whose comments and suggestions significantly improved the manuscript.
Author contributions
Katherine B. Lininger (Conceptualization, Visualization, Writing – original draft, Writing – review & editing), and Rebecca Lave (Conceptualization, Visualization, Writing – original draft, Writing – review & editing)
Author Biography
Katherine B. Lininger ([email protected]) is an assistant professor in the Department of Geography at the University of Colorado Boulder, in Boulder, Colorado, in the United States. Rebecca Lave ([email protected]) is a professor of geography and associate dean for social and historical sciences at Indiana University Bloomington, in Bloomington, Indiana, in the United States; she is also the 2022–2025 vice president, president, and past president of the American Association of Geographers.