-
PDF
- Split View
-
Views
-
Cite
Cite
Jean-François Therrien, Nicolas Lecomte, Thomas Zgirski, Mikaël Jaffré, Andréanne Beardsell, Laurie J. Goodrich, Joël Bêty, Alastair Franke, Edmund Zlonis, Keith L. Bildstein, Long-term phenological shifts in migration and breeding-area residency in eastern North American raptors, The Auk, Volume 134, Issue 4, 1 October 2017, Pages 871–881, https://doi.org/10.1642/AUK-17-5.1
- Share Icon Share
Abstract
Climate change can affect the distribution, abundance, and phenology of organisms globally. Variations in the timing of passage during autumn and spring migration can have consequences at individual and population levels. We assessed whether global climatic indexes and increasing air temperature over a 28 yr period were concurrent with shifts in the autumn migration phenology of 16 eastern North American raptor species. We used count data from 7 eastern North American raptor-migration watch sites and examined whether key species-specific traits such as migration strategy (complete vs. partial and trans-equatorial vs. not), diet specialization, body mass, flight strategy (soaring vs. flapping), and latitude of the northern limit of breeding distribution were associated with a shift in the timing of autumn migration. Our results suggest an overall delay across species in autumn migration passage date of ∼1 day decade−1, which coincided with an increase in temperature across eastern North America. This shift in average autumn passage date was more pronounced in short-distance migrants (+1.03 days decade−1); no shift was detected in trans-equatorial migrants. Although we did not detect clear links between annual climatic indexes and the other life-history traits studied, the results nonetheless indicate that the autumn migration phenology of eastern North American raptors may be delayed by ongoing climate change. However, the amplitude of these effects varies on a species-by-species basis. Our results—combined with new evidence of an earlier passage during spring migration for the same species in the same area—suggest that, since 1985, most raptors spent ∼2 additional days decade−1 north of our study's migration sites. Such an increase in time spent at northern latitudes in a large number of terrestrial avian predators over a wide geographic area may have profound impacts on population and ecosystem dynamics. Unraveling such impacts will require linking interspecific and intraspecific variations in phenological adjustments to ongoing climate change.
Résumé
Les changements climatiques peuvent affecter la répartition, l'abondance et la phénologie des organismes à l'échelle mondiale. Des variations dans la chronologie du passage pendant les migrations automnale et printanière peuvent avoir des conséquences aux niveaux individuel et de la population. Nous avons évalué si les indices climatiques mondiaux et l'augmentation de la température de l'air sur une période de 28 ans coïncidaient avec les changements dans la phénologie de la migration automnale chez 16 espèces d'oiseaux de proie de l'est de l'Amerique du Nord. Nous avons utilisé des données de dénombrement provenant de sept observatoires des migrations d'oiseaux de proie dans l'est de l'Amérique du Nord et examiné si des caractéristiques clés spécifiques aux espèces, tels que la stratégie de migration (complète vs partielle et transéquatoriale vs non transéquatoriale), la spécialisation du régime alimentaire, la masse corporelle, la stratégie de vol (en planant vs en battant des ailes) et la latitude de la limite nord de l'aire de reproduction, étaient associées au changement dans la chronologie migratoire automnale. Nos résultats suggèrent un délai généralisé de la date de passage migratoire en automne d'environ 1 jour*décennie−1, ce qui coïncidait avec une augmentation de la température dans l'est de l'Amérique du Nord. Ce changement dans la date moyenne de passage automnal était plus prononcé chez les migrateurs de courte distance (+1,03 jour*décennie−1) par rapport aux migrateurs transéquatoriaux (aucun changement détecté). Bien que nous n'ayons pas détecté des liens clairs entre les indices climatiques annuels et les autres caractéristiques du cycle vital étudiées, ceci indique néanmoins que la phénologie de la migration automnale chez les oiseaux de proie de l'est de l'Amérique du Nord peut être retardée par les changements climatiques en cours. Cependant, l'amplitude de ces effets varie d'espèce en espèce. Nos résultats, combinés aux nouvelles preuves d'un passage plus hâtif lors de la migration printanière pour les mêmes espèces dans la même région, suggèrent que la plupart des oiseaux de proie ont passé près de deux jours de plus par décennie depuis 1985 au nord des sites de migration de notre étude. Une telle augmentation du temps passé à des latitudes nordiques chez un grand nombre de prédateurs aviaires terrestres sur une vaste zone géographique peut avoir de profonds impacts sur la dynamique de la population et des écosystèmes. Décortiquer de tels impacts nécessitera de relier les variations interspécifiques et intraspécifiques dans les ajustements phénologiques aux changements climatiques en cours.
Mots-clés: Amérique du Nord, caractéristiques du cycle vital, changements climatiques, migration, oiseaux de proie, phénologie
Introduction
Climate change is affecting the distribution of biodiversity in time and space (Lemoine et al. 2007, Pereira et al. 2010, Dawson et al. 2011, Bellard et al. 2012). A warming climate can generate global ecological fingerprints (e.g., Parmesan 2006), targeting the distribution, abundance, and phenology of various organisms. Phenology has received considerable scientific attention, with species shifting the onset of key steps in their annual cycle (e.g., early arrival on breeding grounds or late departure for wintering areas; Walther et al. 2002, Cotton 2003, Gordo and Sanz 2006). Yet temporal variation in climate can also trigger contrasting responses among organisms during their annual cycles. The variation in phenological responses has prompted vigorous questioning of our understanding of interactions between species traits and climate change, especially because species without appropriate responses to climate change may decline (e.g., Møller et al. 2008).
The study of species' sensitivity to climate change has become a growing discipline, in which identifying key links between climate and species traits has taken a central place (e.g., Foden et al. 2013, Pacifici et al. 2015, Thackeray et al. 2016). Because migration encapsulates the movements of animals between critical parts of their annual cycle, many investigations have tracked how migratory animals respond to climatic variability (e.g., global warming and climatic oscillations; Jonzén et al. 2006, Gordo 2007). Specific life-history traits, such as migratory strategy (complete vs. partial and trans-equatorial or not), diet specialization, body mass, flight type (soaring vs. flapping migrants), and latitudinal extent of breeding distribution, may be central to the large spectrum of phenological responses displayed by migratory species (for a review, see Usui et al. 2017).
Several studies have suggested that migration distance can affect how a species adjusts to climatic trends (Both and Visser 2001). Overall, short-distance migrants tend to show a greater plasticity in migration phenology than trans-equatorial, long-distance migrants (Jenni and Kéry 2003, Jonzén et al. 2006, Thorup et al. 2007). The degree of diet specialization could also have consequences for a species' ability to respond to climate change, with generalists being more flexible in response to changing environments (Jiguet et al. 2007, Le Viol et al. 2012). Species body mass, which is related to several specific parameters, such as generation time and trophic level, has also been implicated in differential responses of bird species to climate changes (Møller et al. 2008). Given the fact that climate change is occurring faster at higher latitudes (ACIA 2005), the northern latitudinal extent of a species' breeding distribution could also affect how it reacts to ongoing changes.
The complexity of responses to climate change necessitates large-scale, long-term, and multispecies investigations. The monitoring of bird migration is a key example of global-data acquisition for tracking the ecological fingerprint of global changes. However, most of our knowledge is derived from short-lived species (e.g., Jonzén et al. 2006, Van Buskirk et al. 2009, Wood and Kellermann 2015) and for a single period of their annual cycle (for a review, see Gallinat et al. 2015). That said, upscaling phenological models to species with complex life histories remains a challenge. The detailed and relatively long-term monitoring of long-lived species, such as raptors in eastern North America, offers a unique opportunity to derive an overview of phenological changes in species that have an important role at the top of many ecosystems (e.g., Van Buskirk 2012, Sullivan et al. 2016). Various raptor species use highly contrasting flight strategies during migration, with some species relying almost exclusively on thermal and orographic soaring flight (a time-consuming but energetically efficient strategy) while others rely on powered flapping flight for their migration. Species that use mostly soaring flight during migration are expected to be more affected by ongoing climate change, given that their mode of locomotion relies heavily on weather encountered during migration.
Recent studies investigating phenological shifts of migration in raptors have reported mixed patterns in both Europe (Filippi-Codaccioni et al. 2010, Jaffré et al. 2013) and North America (Van Buskirk 2012, Sullivan et al. 2016). Such outcomes point to the need to conduct phenological analyses for both spring and autumn migration passages, in order to have a more complete view of the changes that are occurring. To investigate the long-term phenological adjustments of top predators to ongoing climate change, we analyzed the timing of autumn migration for 16 of the most common diurnal raptors of eastern North America over a 28 yr period (1985–2012). To do so, we gathered data collected following standard protocols across a network of 7 eastern North American raptor-migration watch sites. We hypothesized that, as in short-lived species (Møller et al. 2008), raptor species with a greater potential for adjustment (short-distance migrants, small species, and diet generalists) would exhibit a greater phenological response than long-distance trans-equatorial migrants, larger species, and diet specialists. We also hypothesized a greater phenological adjustment in species that rely heavily on weather conditions and air currents to migrate (soaring species) and in more northerly distributed species, for which climate seems to be changing faster. Lastly, to assess the variation in time spent north of our study's migration sites (i.e. breeding-area residence time [BART]; Thorup et al. 2007) for each species, we integrated results of a complementary study done on spring migration using similar time series over a similar geographic area (Sullivan et al. 2016).
Methods
Migration Data
Several raptor-migration watch sites have operated annually since the 1930s at convergence points, or bottlenecks, along migratory flyways in North America, enhancing our ability to track and understand migration behavior (e.g., Bednarz et al. 1990, Bildstein et al. 2008). Recently, most raptor-migration watch sites have adopted a standard monitoring protocol, designed in the 1970s by the Hawk Migration Association of North America (for details, see Therrien et al. 2012), which has maximized repeatability of observations across years. Here, we used migration count data from all sites located in eastern North America for which we had long-term (>25 yr) datasets (n = 7; Figure 1).
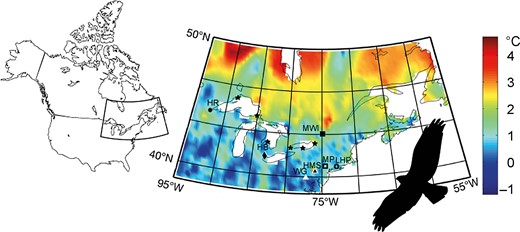
Mean air-temperature changes across eastern North America from 1985 to 2012. Locations of the raptor-migration watch sites used in the study are indicated with solid and open circles, squares, triangles, and diamonds (for details and full names of sites, see Supplemental Material). We also added the spring migration sites of Sullivan et al. (2016; denoted by stars), since we combined these data to calculate breeding-area residence time (BART). Spring estimates are not available for Peregrine Falcon and Black Vulture.
To derive complete series with similar data resolution, we used daily counts collected between 1985 and 2012. For each site, we removed the data collected outside of the monitoring time window conducted by individual sites (for details, see Supplemental Material Table S1 and Figure S1), because such data are generally nonstandardized. We retained only daily counts of ≥2 hr (i.e. the lower limit required to analyze migration; Bensusan et al. 2007, Farmer et al. 2007). Counts were then converted into hourly rates (number observed per hour) to account for variation in monitoring duration.
When studying migration phenology, it is essential to consider the seasonal migration window for each species to ensure that monitoring includes nearly the whole distribution of migration dates (Supplemental Material Figure S1). Here, we defined species-specific seasonal windows as the central 95% of the passage distribution recorded for all years, following Hoffman and Smith (2003) and Farmer et al. (2007). On the basis of these criteria, we obtained a passage window for each species and site. To ensure the homogeneity of our analyses, we further removed from the analyses any time series (year*site*species) with <50% of days overlapping the species-specific passage window and extending beyond the site-specific monitoring time window (n = 39, or <1.3%). Considering all the above steps (Supplemental Material Figure S1), a total of 16 species was included in our analyses.
We used the mean passage date (MPD) as a phenological estimator for each species, site, and year. Compared with other commonly used phenological estimators (i.e. first record, 10th record, 25%, 95%), MPD provides relatively unbiased estimates of phenological change and is less sensitive to sample size, species detectability, and distribution or sampling frequency (Moussus et al. 2010). To estimate BART, we combined our MPD values during autumn migration with those of Sullivan et al. (2016), which were obtained for the spring migration for the same species across 6 raptor migration watch sites and over a similar geographic area.
Climatic data
The North Atlantic Oscillation (NAO) is an established global climate index that reflects a combination of weather patterns at a continental scale over the Northern Hemisphere (Hurrell 1995). High NAO values correspond to a low-pressure system around Iceland with westerly winds, creating hot and humid conditions in northeastern North America. We obtained NAO values for 3 seasons—spring (April–May), summer (June–July), and autumn (August–October, inclusively)—from the Climate Prediction Center of the National Weather Service (http://www.cpc.ncep.noaa.gov).
Temperature has a prominent role in the timing of events of the annual cycle and consequently affects the timing of their succession (i.e. the arrival date of a bird on its breeding ground could explain its departure date; Thorup et al. 2007). We selected all available weather stations situated in a geographic area of 4.106 km2 (55–95°W, 40–55°N; Supplemental Material Figure S2), encompassing the 7 raptor-migration watch sites selected in the present study and stretching to the north in order to reflect the general air temperatures occurring on the breeding grounds for most of the 16 raptor species studied here. We obtained interpolated monthly temperatures from the NOAA database (National Oceanic and Atmospheric Administration 2015).
Species-specific Life-history Traits
We tested whether a species-specific shift in MPD could be explained by the following life-history traits: migration strategy, diet diversity, body mass, flight strategy (soaring vs. flapping flight), and the latitude of the northern limit of each species' breeding distribution. For migration strategy, species were classified as either complete or partial migrants and as either trans-equatorial migrants or not (sensuBildstein 2006). Diet diversity (values ranging from zero for a true specialist to 1.48 for generalists) was calculated as a diversity index of the main prey species classified into groups (mammals, birds, fishes, amphibians, reptiles, and invertebrates) and weighted according to the diet proportions reviewed by Sherrod (1978; Supplemental Material Table S2). The 2 obligate scavenger species were considered generalists because their diet includes carrion from all groups. Biological and ecological data (i.e. average body mass in kilograms, flight strategy, and latitude of the northern limit of each species' distribution) were collected for each species from Birds of North America Online accounts (http://bna.birds.cornell.edu/bna) accessed in 2014 (Table 1 and Supplemental Material Table S3).
Phenological adjustment (days yr−1) in 16 eastern North American raptor species during autumn migration and their associated life-history traits. Estimates whose confidence intervals (95% CI) do not (or marginally) overlap zero are considered biologically informative and are in bold. Sample size (n) refers to the total number of years*sites for which we have a value.
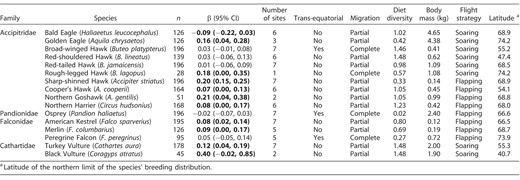
Phenological adjustment (days yr−1) in 16 eastern North American raptor species during autumn migration and their associated life-history traits. Estimates whose confidence intervals (95% CI) do not (or marginally) overlap zero are considered biologically informative and are in bold. Sample size (n) refers to the total number of years*sites for which we have a value.
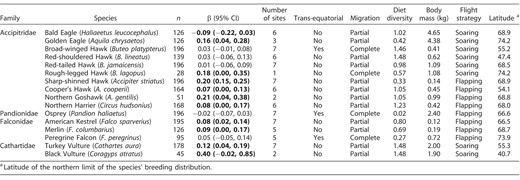
Analyses
We examined the general air-temperature trends over the study years using a linear regression. We ran a second analysis to compare air temperature trends between spring and autumn because we were interested in assessing variation between migratory seasons. We used a linear mixed-effects model (LMM) to examine the overall temporal trend in MPD for all species. We also ran models separately for each species. Only site ID was treated as a random effect in all models. Slope estimates for each species (hereafter “autumn phenological trends”) are provided in Table 1.
We used LMMs to determine which climatic variables were important predictors of MPD and used the following as fixed effects: mean air temperature and mean daily NAO for spring (April–May), summer (June–August), and autumn (September–October). To aid in the comparison of coefficients, we centered all climate variables on the mean and standardized by the standard deviation (Schielzeth 2010). Because we expected high variation among species and years, these variables were included as fixed effects, and site ID was treated as a random effect in all models. Collinearity among independent variables was checked with Pearson correlations, and we did not include highly correlated variables (r ≥ 0.7) simultaneously within models (none of the climate variables were highly correlated among them). We also examined variance inflation factors and did not find any variable going beyond a critical threshold of 3 (Zuur et al. 2010). Models were ranked according to Akaike's Information Criterion corrected for small sample size (AICc; Burnham and Anderson 2002). Model-averaged estimates and 95% confidence intervals (CI) were computed with multimodel inference on the best models (i.e. ΔAICc < 4). To assess the amount of variation explained by our models, we report the marginal R2 (for fixed effects) calculated with the method developed by Nakagawa and Schielzeth (2013) for mixed-effect models.
We examined the relationship between autumn-migration phenological trend and life-history traits of each species using linear regressions. Only trends where the 95% CI of the slope excluded zero or the ones with marginal effects (i.e. <25% of the 95% CI of the slope includes zero; Nakagawa and Cuthill 2007) were considered biologically informative and were used in the model. Because we were also interested in assessing variation in time spent north of our study's migration sites (BART; Thorup et al. 2007) in relation to life-history traits, we ran a second analysis using autumn and spring migration phenological trends (obtained from Sullivan et al. 2016) for each species as a dependent variable. Model development and selection followed the procedure outlined above (linear models, marginal effects).
The lme4 package was used to estimate the parameters of all LMMs (Bates et al. 2015), and the “AICcmodavg” package was used for model selection and multimodel inference (Mazerolle 2016). All analyses were performed in R 3.1.1 (R Core Team 2017).
Results
The annual mean temperature trend showed an increasing pattern (+1.17°C, R2 = 0.32) in the study area during 1985–2012 (Figure 1 and Supplemental Material Figure S2). This increase was similar between autumn (August–September–October; +0.9°C) and spring (April–May; +0.7°C). Mapping the temperature anomalies revealed that the northern latitudes, corresponding to the breeding grounds for most of the study species, exhibited the strongest positive changes (Figure 1).
Annual trends in MPD during autumn migration displayed an overall delay of 1.0 day decade−1 over the 28 yr study period. The autumn phenological trends, however, were highly variable among species (Table 1, Figure 2, and Supplemental Material Figure S3). Overall, species tended to delay their autumn passage date when autumn NAO values were lower, associated with drier but colder weather conditions. We detected marginal effects of summer air temperature and NAO as well as spring NAO on overall autumn passage dates (Table 2).
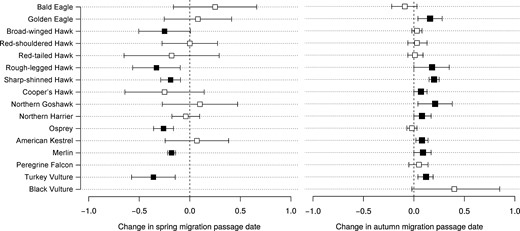
Phenological shifts in migration and variation in breeding-area residence time (BART) of 16 eastern North American raptor species according to spring and autumn annual mean passage dates. Spring migration values were obtained from Sullivan et al. (2016; see text); autumn migration values are derived from the present study. Estimates whose confidence intervals (95% CI) did not overlap zero are marked with solid symbols. For scientific names of species, see Table 1.
(A) Model selection among candidate models explaining mean passage date (MPD; n = 2005) of 16 eastern North American raptor species during autumn migration in relation to global climatic index (NAO) and air temperature (T). Number of parameters (k), ΔAICc values, AICc weights (wi), log-likelihood values (LL), and marginal R2 are presented for the models with ΔAICc < 4. Site ID was used as a random factor in the analysis. (B) Model-averaged parameter estimates from the models with ΔAICc < 4 and their 95% confidence intervals (CI). Estimates whose confidence intervals (95% CI) do not (or marginally) overlap zero are considered biologically informative and are in bold.
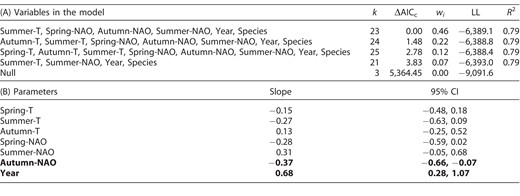
(A) Model selection among candidate models explaining mean passage date (MPD; n = 2005) of 16 eastern North American raptor species during autumn migration in relation to global climatic index (NAO) and air temperature (T). Number of parameters (k), ΔAICc values, AICc weights (wi), log-likelihood values (LL), and marginal R2 are presented for the models with ΔAICc < 4. Site ID was used as a random factor in the analysis. (B) Model-averaged parameter estimates from the models with ΔAICc < 4 and their 95% confidence intervals (CI). Estimates whose confidence intervals (95% CI) do not (or marginally) overlap zero are considered biologically informative and are in bold.
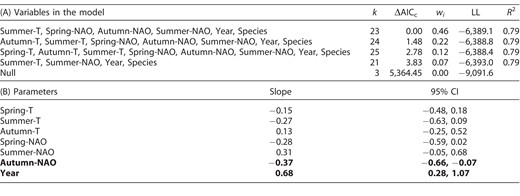
Contrary to our prediction, we did not detect any direct relationship of air temperature (spring, summer, or autumn) on MPD trends in any of the large soaring raptors—Bald Eagle, Golden Eagle, Red-shouldered Hawk, Red-tailed Hawk, and Rough-legged Hawk (Table 3). (For scientific names of species, see Table 1.) Among mostly soaring migrant species, only Broad-winged Hawk and Northern Harrier delayed their MPD in response to an increase in air temperature in autumn (Table 3). Black Vulture, another soaring raptor, showed a negative relationship between MPD and autumn air temperature (Table 3). As expected, however, all 3 species of falcons (non-soaring migrants) did not show any direct variation in their MPD according to variation in air temperature (Table 3). The link between NAO values and MPD were not consistent among species (Table 3). Only 3 species showed direct informative trends between autumn NAO and MPD: for Golden Eagle and Red-tailed Hawk the trend was negative, and for American Kestrel the trend was positive. Overall, several species did not display any direct relationship between MPD and any of the tested climate covariates (seasonal air temperature and NAO; Table 3).
Model-averaged parameter estimates (with 95% confidence intervals) from the models with ΔAICc < 4 explaining variation in outbound phenological trends of 16 eastern North American species in relation to climatic variables. A random effect for site was included in all models (except for Rough-legged Hawk because the number of sites = 1). Estimates whose confidence intervals (95% CI, in parentheses) do not (or marginally) overlap zero are considered biologically informative and are in bold. NA = not retained in the most parsimonious models. For scientific names of species, see Table 1.
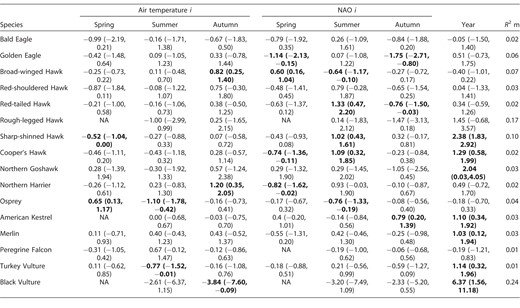
Model-averaged parameter estimates (with 95% confidence intervals) from the models with ΔAICc < 4 explaining variation in outbound phenological trends of 16 eastern North American species in relation to climatic variables. A random effect for site was included in all models (except for Rough-legged Hawk because the number of sites = 1). Estimates whose confidence intervals (95% CI, in parentheses) do not (or marginally) overlap zero are considered biologically informative and are in bold. NA = not retained in the most parsimonious models. For scientific names of species, see Table 1.
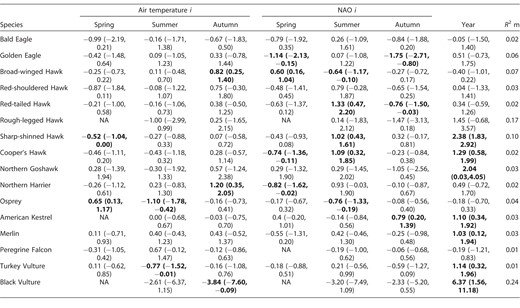
The long-distance trans-equatorial migrants—Broad-winged Hawk, Osprey, and Peregrine Falcon—did not show any phenological adjustment in autumn passage dates, but shorter-distance migratory species (not crossing the equator; 13 species) tended to delay their MPD by 1.03 days decade−1 (Figure 2 and Supplemental Material Table S4). We did not detect any pattern in adjustment of autumn phenological trends according to species body mass, diet diversity, flight strategy, or the latitude of the northern limit of each species' distribution (Supplemental Material Table S4).
Combined with overall earlier spring passage dates (Sullivan et al. 2016), we detected an overall increase in BART of ∼1.9 days decade−1 over the 28 yr study period (Figure 2). The adjustment in BART was not different between short- and long-distance trans-equatorial migratory species or according to diet diversity (Supplemental Material Table S5). Moreover, we did not detect any pattern in adjustment of BART according to body mass, flight strategy, or the latitude of the northern limit of each species' distribution (Supplemental Material Table S5).
Discussion
We found that common migratory raptors of eastern North America exhibited an overall delay in autumn passage dates between 1985 and 2012. This delay during autumn is coupled with an overall earlier passage of the same species during spring migration, as reported by Sullivan et al. (2016). This suggests that, for most of those species, time spent north of our study's migration sites is increasing. Such an increase may result from an earlier arrival to, and/or a delayed departure from, the breeding grounds—resulting in increased time spent on the breeding grounds. However, since we are monitoring birds en route to and from their breeding grounds (and not on the breeding grounds per se), we acknowledge the fact that the observed pattern could also result from an increase in migration duration or a change in the overall migration distance, or a combination of these elements.
Our results parallel ongoing climatic trends and are thus in agreement with predicted reduced migratory distances and increased residency, as suggested by Pulido and Berthold (2010), Van Buskirk (2012), and Jaffré et al. (2013). This increase in time spent north of our study's migration sites—observed in a large proportion of species, comprising the bulk of terrestrial avian predators over a wide geographic area—is likely to have significant effects on mid-term and long-term ecosystem dynamics. However, more research is needed to assess the mechanisms of these changes. These results nonetheless add to the growing evidence that climate change is affecting multiple biological aspects of raptors and of wildlife in general, such as winter distribution and abundance (e.g., Kim et al. 2008, Paprocki et al. 2014), spring migration (e.g., Tøttrup et al. 2006, Sullivan et al. 2016), timing of reproductive activities (e.g., Jonzén et al. 2006), timing of arrival on wintering sites (Carey 2009), and even survival rate (e.g., Franke et al. 2011). Such phenological shifts will have to be taken into consideration for raptor-migration counting protocols, in order to maintain adequate coverage of the migration window.
Annual phenology in raptor passage during autumn migration in eastern North America appeared to be related to global climatic indexes (e.g., NAO), but the mechanism for the relationship remains unclear. Indeed, we found that overall passage dates happened later with decreasing autumn NAO values. Other climatic proxies, such as summer and spring NAO, provided only marginal effects with opposite trends. The fact that most species studied reacted weakly and sometimes in opposing ways (e.g., Table 3) to variations in climate indexes (air temperature, NAO) strongly suggests that the effects of climate change on the migration phenology of avian top predators seem to be acting indirectly, possibly through complex food-web interactions (e.g., Thackeray et al. 2016). To our knowledge, however, comprehensive data to reliably and precisely address this topic on eastern North American raptor species are not available at this time. A species-specific approach is warranted (e.g., Kim et al. 2015) to better understand the underlying processes and decipher how intraspecific and interspecific parameters rank in terms of response to similar climate variance.
Overall, the 3 long-distance trans-equatorial migrant raptor species in eastern North America showed no detectable trend in phenology during autumn migration. This is in agreement with the hypothesis that migration phenology is likely less plastic in trans-equatorial species than in short-distance species and mainly regulated by photoperiod rather than by local climatic conditions (Newton 2008). As suggested by Visser et al. (2004), this phenomenon could have huge impacts on trans-equatorial species because the lack of adjustment may lead to an asynchrony with their prey. Our results nonetheless contrast with those of Sullivan et al. (2016) and Van Buskirk (2012), who found some support, albeit weak, for an earlier passage in some trans-equatorial species during spring migration. The magnitude in delay for autumn migration passage dates was very similar between short-distance migrants from separate continents (1.03 vs. 1.0 days decade−1 in eastern North America and Europe, respectively; Filippi-Codaccioni et al. 2010, present study). Nonetheless, our results contrast with those of Filippi-Codaccioni et al. (2010), who detected earlier autumn-migration passage dates in 6 of 8 long-distance European migratory raptors, albeit at a single migratory watch site. Those authors suggested the need to investigate population structure to understand the heterogeneity in phenological adjustments with age and sex, for instance different responses to migratory constraints such as territory establishment. Altogether, these results call for a species-specific assessment in which population structure, species traits, interspecific interactions, and individual tracking are taken into account.
The phenological adjustments observed in eastern North American raptors remained largely unaffected by other species-specific life-history traits such as diet specialization, body mass, flight strategy, and the latitude of the northern limit of breeding distribution (the latter being possibly confounded by the fact that some species ranges might also be shifting). This negative result could be explained, in part, by the limited statistical power of the analyses. Indeed, even though this comprehensive study investigated the influence of life-history traits on phenological adjustments of 16 species of raptors, the response variable is still limited to 16 units, so the power to reject the null hypothesis remains somewhat low. Nevertheless, this result further illustrates the challenges of predicting the future response of species to current global change and the potential consequences for populations and ecosystems. To tackle such challenges, Thackeray et al. (2016) recently suggested that novel research avenues could incorporate the indirect pathways by which climate can modify predator phenology (e.g., through food-web interactions). Deciphering the mechanism(s) underlying responses to climate change and the potential carryover effects on individuals is essential for determining how phenology and migration changes affect long-term population trends.
Acknowledgments
We are thankful to the various participating raptor-migration watch sites, the many dedicated raptor-migration counters, and the Hawk Migration Association of North America, who provided the archived data for analyses. Constructive suggestions from three anonymous reviewers improved the manuscript. This is Hawk Mountain Sanctuary contribution to conservation science no. 285.
Funding statement: Funding was provided by NSERC and Canada Research Chair to N.L. and by EnviroNorth and Mitacs Accelerate grants to M.J., A.F., and J.B.
Ethics statement: This study followed Hawk Mountain Sanctuary's ethic protocols. All authors declare no conflict of interest.
Author contributions: J.-F.T., N.L., T.Z., M.J., J.B., and K.L.B. designed the study. J.-F.T., N.L., A.B., T.Z., and M.J. performed the analyses. J.-F.T., T.Z., and N.L. wrote the manuscript. All authors commented on the final version of the manuscript.
Literature Cited
Author notes
These authors contributed equally to the paper.